• An animal can be made immune to infection in two general ways—passive immunization and active immunization. • Passive immunization by administering preformed antibodies made in a normal animal provides immediate protection, but the resulting immunity is short lived. • Active immunization using vaccines containing live or dead organisms produces slowly developing but long-lasting immunity. • Live vaccines tend, in general, to stimulate a more effective immune response than vaccines containing killed organisms. However, vaccines containing killed organisms have tended to be somewhat safer. • Innovative molecular techniques, such as the use of DNA vaccines or reverse vaccinology, may permit the development of vaccines against diseases for which current vaccines are ineffective or not available. • Adjuvants are substances added to vaccines to enhance their effectiveness. There are two basic methods by which any animal may be made immune to an infectious disease (Figure 23-1): passive and active immunization. Passive immunization produces temporary immunity by transferring antibodies from a resistant to a susceptible animal. These passively transferred antibodies give immediate protection, but since they are gradually catabolized, this protection wanes, and the recipient eventually becomes susceptible again. Active immunization, in contrast, involves administering antigen to an animal so that it responds by mounting an immune response. Reimmunization or exposure to infection in the same animal will result in a secondary immune response and greatly enhanced immunity. The disadvantage of active immunization is that, as with all adaptive immune responses, protection is not conferred immediately. However, once established, immunity is long-lasting and capable of restimulation (Figure 23-2). Although immune globulins give immediate protection, some problems are associated with their use. For instance, when horse tetanus immune globulin is given to a cow or dog, the horse proteins will be perceived as foreign, elicit an immune response, and be rapidly eliminated (Figure 23-3). To reduce antigenicity, immune globulins are usually treated with pepsin to destroy their Fc region and leave intact only the portion of the immunoglobulin molecule required for toxin neutralization—the F(ab)′2 fragment. If circulating horse antibody is still present by the time the recipient animal mounts an immune response, the immune complexes formed may cause a type III hypersensitivity reaction called serum sickness (Chapter 30). If repeated doses of horse immune globulin are given to an animal of another species, this may provoke immunoglobulin E (IgE) production and anaphylaxis (Chapter 28). Finally, the presence of high levels of circulating horse antibody may interfere with active immunization against the same antigen. This is a phenomenon similar to that seen in newborn animals passively protected by maternal antibodies. Sometimes passive immunization may have unexpected side effects (Box 23-1). The advantages of vaccines such as brucella strain 45/20 that contain killed organisms are that they are safe with respect to residual virulence and are relatively easy to store since the organisms are already dead (Box 23-2). These advantages of killed vaccines correspond to the disadvantages of live vaccines, such as strain 19 or RB-51. That is, some live vaccines may possess residual virulence, not only for the animal for which the vaccine is made but also for other animals. They may revert to a fully virulent type or spread to unvaccinated animals. Thus some vaccine strains of porcine reproductive and respiratory syndrome virus (PRRSV) vaccine may be transmitted to unvaccinated pigs, causing persistent infection and disease. Live vaccines always run the risk of contamination with unwanted organisms; for instance, outbreaks of reticuloendotheliosis in chickens in Japan and Australia have been traced to contaminated Marek’s disease vaccines. A major outbreak of bovine leukosis in Australia resulted from contamination of a batch of babesiosis vaccine containing whole calf blood. Abortion and death have occurred in pregnant bitches that received a parvovirus vaccine contaminated with bluetongue virus. Contaminating mycoplasma may also be present in some vaccines. Scrapie has been spread in mycoplasma vaccines. Finally, vaccines containing living attenuated organisms require care in their preparation, storage, and handling to avoid killing the organisms. Maintaining the cold chain can account for 20% to 80% of the cost of a vaccine in the tropics.
Vaccines and Their Production
Types of Immunization Procedures
Passive Immunization
Active Immunization
Living and Killed Vaccines
< div class='tao-gold-member'>
Stay updated, free articles. Join our Telegram channel

Full access? Get Clinical Tree
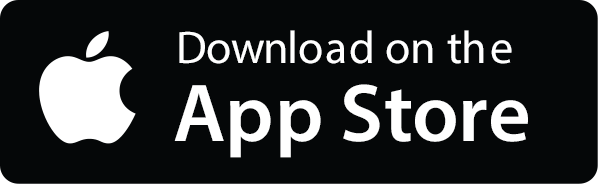
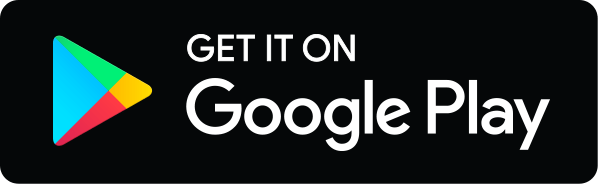