• Type I hypersensitivities, also called immediate hypersensitivity, are mediated by immunoglobulin E (IgE) attached to mast cells. • Disease is caused by the release of inflammatory molecules from mast cells following the binding of antigens to IgE. • The clinical signs of allergic disease depend in large part on the route by which antigens (allergens) enter the body. • Massive systemic release of inflammatory molecules by mast cells may give rise to allergic anaphylaxis. In this syndrome, animals may collapse and die rapidly as a result of the contraction of critical smooth muscles such as those lining the bronchi. • Animals commonly suffer from allergies to foods, inhaled antigens, vaccines, or drugs. • In many cases, especially in the dog, these allergies may manifest as intense pruritus. • Treatment may include epinephrine for allergic anaphylaxis, corticosteroids for local inflammation, and desensitizing injections of allergen for prolonged control. The most satisfactory solution is to prevent exposure to the offending allergens. Type I hypersensitivity reactions are a form of acute inflammation that results from the interaction of antigens with mast cell–bound IgE. This leads to the release of mast cell granule contents (Figure 28-1). The granule contents in turn cause acute inflammation. The benefits of this type of inflammation are unclear, but it is of major clinical significance in veterinary medicine (Box 28-1). Environmental factors such as childhood infections also influence the development of atopic diseases in humans. Children who have had multiple infections when young appear to be less likely to develop allergies than those not exposed to such infections. On the other hand, contact with allergens on the first day of life predisposes puppies to develop significantly higher IgE levels than puppies sensitized at 4 months of age. As discussed in Chapter 22, there is a growing belief that the development of the immune system, and especially a tendency to develop allergies, is regulated by the intestinal microflora. It is possible that the great increase in allergic disease observed in developed countries is influenced by changes in the microflora brought about by changes in diet or antibiotic use. Normal animals infested by parasitic worms and insects also tend to produce large amounts of IgE. It is believed that the IgE response may have evolved specifically to counteract these organisms. Chitin, the biopolymer that confers structural rigidity to fungi, insects, and helminths, induces the accumulation of cells such as eosinophils and basophils in tissues and may be a key trigger of some of these allergic reactions. Indeed, the self-cure reaction seen in parasitized sheep has long been the only well-characterized beneficial feature of type I hypersensitivity. It is of interest to note that atopic and parasitized dogs may have reduced IgA levels, an observation supporting the concept that a deficiency of IgA may predispose to a compensatory increase in IgE production (Chapter 22). IgE is an immunoglobulin of conventional four-chain structure and is about 200 kDa in size (see Figure 16-7). It is found in serum in exquisitely small quantities (9 to 700 µg/mL in dogs), and its half-life there is only 2 days. Most of the body’s IgE is firmly bound to Fcε receptors on tissue mast cells, where it has a half-life of 11 to 12 days. Some IgG subclasses may also bind to mast cell receptors and mediate type I hypersensitivity reactions. For example, IgG4 is associated with atopic dermatitis in the dog. However, the affinity of these subclasses for mast cells is much lower than that of IgE, and they are of much less clinical significance. Atopic individuals are predisposed to generate Th2 cells. The Th2 cells produce interleukin-4 (IL-4), IL-5, and IL-13. These cytokines, together with co-stimulation from CD40, trigger B cell IgE synthesis. IL-4 is also produced in significant amounts by stimulated mast cells. This mast cell–derived IL-4 may alter the helper cell balance and enhance yet more Th2 cell production and IL-4 release (Figure 28-2). Some allergic humans overexpress IL-4, leading to excessive Th2 cell activity and enhanced IgE production. There are two types of IgE receptors: high-affinity FcεRI and low-affinity FcεRII (CD23). There are two forms of FcεRI. One form is found on mast cells, basophils, neutrophils, and eosinophils. This form consists of four chains, one α, one β, and two γ chains (αβγ2) (Figure 28-3). The α chain binds IgE, the β chain stabilizes the complex, and the γ chains serve as signal transducers. (This same γ chain is also a signal transducer in FcγRI, FcγRIII, and γ/δ TCR.) The affinity of FcεRI for IgE is very high (10−10 M), so they bind almost irreversibly. The presence of FcεRI ensures that mast cells are constantly coated with IgE. The second form of FcεRI consists of three chains: one α and two γ chains (αγ2). It is found on antigen-presenting dendritic cells and monocytes. When an antigen binds to IgE and the complex binds to this receptor, it is ingested and treated as exogenous antigen. The expression of FcεRI on antigen-presenting cells is enhanced by IL-4 from Th2 cells. Thus a positive feedback loop (the allergy loop) develops (Figure 28-4). The antigen processing cells present antigen more effectively to Th2 cells. The Th2 cells then secrete IL-4 and enhance IgE production. The second IgE receptor, FcεRII (CD23), is a selectin found on B cells, natural killer (NK) cells, macrophages, dendritic cells, eosinophils, and platelets. In addition to binding IgE, FcεRII also binds to the complement receptor CR2 (CD21) (Figure 28-5). Thus B cells expressing FcεRII will bind CR2 on other B cells, T cells, and dendritic cells. By binding B cells to dendritic cells, FcεRII enhances B cell survival and promotes IgE production. Mast cells have long been known to play a key role in allergic diseases. They also play a major role in innate immunity. Because they are located close to body surfaces as well as their ability to release proinflammatory molecules within seconds, mast cells serve as sentinel cells. They are covered by an array of receptors that permit them to react in response to many different stimuli. For example, they release inflammatory molecules in response to microbial invasion or tissue damage (Chapter 3). This release normally occurs in a controlled manner and ensures that the severity and type of inflammation are appropriate to the body’s immediate needs. Mast cells are large, round cells (15 to 20 µm in diameter) scattered throughout the body in connective tissue, under mucosal surfaces, in the skin, and around nerves (Figure 28-6). They are found in greatest numbers at sites in the body exposed to potential invaders such as under the skin or in the intestine and airways. In these locations they are located close to blood vessels, where they can regulate blood flow and influence cellular migration. They are easily recognizable because their cytoplasm is densely packed with large granules that stain very strongly with dyes such as toluidine blue. These granules often mask the large, bean-shaped nucleus (Figure 28-7). (Mast cells are so called because, being full of granules, they were considered to be “well-fed cells” [German Mastzellen]). Mast cells originate from myeloid stem cells in the bone marrow. Their precursors emigrate to tissues, where they mature and survive for several weeks or months. In rodents, mast cells from connective tissue and skin and from the intestinal mucosa differ both chemically and structurally (Table 28-1). For example, connective tissue and skin mast cells are rich in histamine and heparin, whereas intestinal mast cells contain chondroitin sulfate and have little histamine in their granules. Although connective tissue mast cells remain at relatively constant levels, mucosal mast cells can proliferate. It has been suggested that the mucosal mast cells respond specifically to invasion by parasitic worms. Table 28-1 Comparison of Two Major Types of Mast Cell Although there are numerous ways by which mast cells can be activated, the best studied of these is mediated by IgE bound to FcεRI on the surface of mast cells (Figure 28-8). A mast cell coated in this way is primed to bind antigen. The mast cell can reside in tissues, with its attached IgE acting like a mine in a minefield. If an antigen encounters the mast cell and cross-links two bound IgE molecules, the mast cell is triggered to release its granules and their contents into the surrounding tissues. This triggering of granule exocytosis is initiated when an antigen molecule cross-links two FcεRI and activates several tyrosine kinases. These, in turn, activate phospholipase C, leading to the production of diacylglycerol and inositol triphosphate. These mediators then increase intracellular calcium and activate more protein kinases. These protein kinases phosphorylate myosin in the cytoskeleton and make the granules move to the cell surface. Granule membranes then fuse with the plasma membrane, and their contents are released into the extracellular fluid (Figure 28-9). Cross-linking of two FcεRI by an antigen also activates phospholipase A, which acts on membrane phospholipids to produce arachidonic acid. Other enzymes then convert the arachidonic acid to leukotrienes and prostaglandins (see Figure 3-6). Finally, the protein kinases promote transcription and expression of genes coding for many different cytokines as well as the genes for cyclooxygenases and lipoxygenase. These mast cell responses are extremely rapid. For example, degranulation occurs within seconds after antigen binds to IgE (Figure 28-10). Because the release is rapid and extensive, sudden acute inflammation develops. Degranulated mast cells do not die but, given time, will regenerate their granules. In normal inflammation, mast cells may release their inflammatory mediators relatively slowly in a process called piecemeal degranulation. The degranulation of mast cells is the central event in the development of allergic (type I hypersensitivity) reactions. The contents of these granules consist of a mixture of potent proinflammatory molecules (Chapter 3). It is now recognized that there are distinct granule subsets within mast cells and that these different subsets may be released under different conditions. Some granules in mouse mast cells contain serotonin or cathepsin D, whereas others contain histamine and tumor necrosis factor-α (TNF-α). Different stimuli associated with specific isoforms of membrane fusion proteins determine which granule subsets are exocytosed and thus which mediators are released. The significance of this lies in the possibility that different clinical forms of allergy may be determined by the granule subset released. Some less typical allergic manifestations may be a result of the release of the contents of different granule subsets. Likewise, appropriate treatment of allergies may differ according to the mixture of inflammatory mediators released. Mast cell granules are loaded with a complex mixture of inflammatory mediators, enzymes, and cytokines. Triggering of receptor-bound IgE by antigen causes the mast cells to degranulate and release stored molecules, which triggers the productions of new mediators. All these molecules (both preformed and newly synthesized) generate the acute inflammation characteristic of type I hypersensitivity response (Figure 28-11). The most important include histamine, serotonin, prostaglandins, and the leukotrienes. Mast cells secrete multiple cytokines, chitinases, and the chemokine CCL3. These cytokines are proinflammatory or promote Th2 responses, or both. High levels of these cytokines are found in tissue fluids in allergic reactions. It is no coincidence that mast cells also produce and secrete chitinases. Chitin is characteristically found in insects, fungi, and helminths, and the production of chitinases supports the suggestion that allergic reactions may have evolved to combat these invaders. Chitin itself is a key allergen in some helminth infections. IL-33 is a member of the IL-1 family that plays an important role in inflammation and promotes Th2 responses leading to allergies (Figure 28-12). Unlike IL-1 and IL-18, it is produced as an active 18-kDa molecule that does not require cleavage of a precursor. It is produced by smooth muscle cells, epithelial cells, fibroblasts keratinocytes, dendritic cells, and activated macrophages. IL-33 also escapes when cells undergo necrosis and has similar activities to HMGB1. In the presence of IgE, IL-33 binds to a receptor on mast cells, basophils, and Th2 cells. When bound to mast cells, it induces their degranulation and so triggers acute anaphylaxis in the absence of antigen. IL-33 cannot mediate this degranulation alone. The mast cells must be sensitized by prior exposure to IgE. It also activates basophils and induces basophil differentiation from bone marrow cells. Mast cells express two surface receptors for catecholamines called the α and β adrenoceptors. These G-protein–linked receptors have opposing effects. Molecules that stimulate the α adrenoceptors (such as norepinephrine and phenylephrine) or block the β adrenoceptors (such as propranolol) enhance mast cell degranulation (Table 28-2). In contrast, molecules that stimulate β receptors or block α receptors inhibit mast cell degranulation. β Stimulators include isoproterenol, epinephrine, and salbutamol and are widely used in the treatment of allergies. β Receptor blockers enhance mast cell degranulation and promote allergies. Some respiratory pathogens such as Bordetella pertussis and Haemophilus influenzae can cause β blockade. As a result, the airways of infected animals are more likely to become severely inflamed because of mast cell degranulation. These infections may also predispose animals to the development of respiratory allergies. Table 28-2 Effects of Stimulating α and β Adrenoceptors When antigen is injected into the skin of an allergic animal, two waves of inflammation occur. There is an immediate acute inflammatory response that occurs within 10 to 20 minutes as a result of the release of the preformed mast cell mediators. This is followed several hours later by a second wave called the late-phase reaction, which peaks at 6 to 12 hours and then gradually diminishes. This late-phase reaction is characterized by redness, edema, and pruritus. It is believed that this late reaction results from the release of inflammatory mediators by T cells, endothelial cells, neutrophils, and macrophages attracted by mast cell chemotactic factors. Th17 cells may play a role in this late process (Chapter 20).
Type I Hypersensitivity
Induction of Type I Hypersensitivity
Immunoglobulin E
Immunoglobulin E Production
Immunoglobulin E Receptors
Mast Cells
Structure and Location
Life History
MUCOSAL MAST CELLS
CONNECTIVE TISSUE MAST CELLS
Structure
Few, variable- sized granules
Many uniform granules
Size
9 to 10 µm diameter
19 to 20 µm diameter
Proteoglycan
Chondroitin sulfate
Heparin
Histamine
1.3 pg/cell
15 pg/cell
Life span
<40 days
>6 months
Location
Intestinal wall, lung
Peritoneal cavity, skin
Response of Mast Cells to Antigen
Mast Cell–Derived Mediators
Interleukin-33
Regulation of Mast Cell Degranulation
SYSTEM
α RECEPTOR STIMULATION OR β BLOCKADE
β RECEPTOR STIMULATION OR α BLOCKADE
Mast cells
Enhances degranulation
Suppresses degranulation
Smooth muscle
Contracts
Relaxes
Blood vessels
Constricts
Dilates
Late-Phase Reaction
Stay updated, free articles. Join our Telegram channel

Full access? Get Clinical Tree
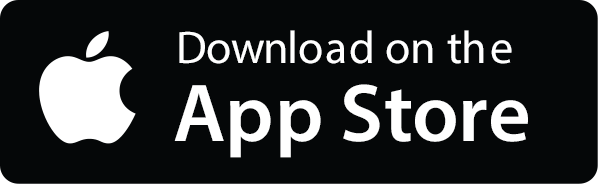
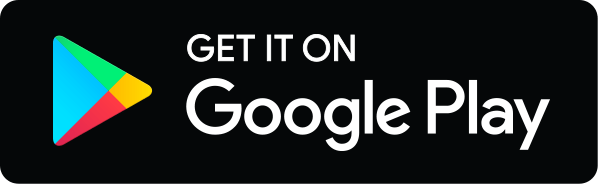