1. The eye’s anatomy is adapted to the eye’s role as a visual receptor organ. 2. Through the process of accommodation, the lens changes shape to focus images from various distances onto the retina. 3. The vertebrate retina consists of five major cell types. 4. In some species the fovea minimizes distortion of light compared with other areas of the retina. 5. Tissue layers behind the retina principally absorb light, or can reflect light, depending on the species’ habits. 6. Photoreception and transduction of light occur in the rods and cones. 7. Visual image processing in the retina begins as the response of the photoreceptor to light is synaptically transmitted to the ganglion cells by the bipolar cells. 8. The electroretinogram records the electrical response of the retina to a flashing light. 9. Ganglion cell axons transmit action potentials to the visual cortex by way of the lateral geniculate nucleus. 10. The diameter of the pupil is controlled by the autonomic nervous system. 11. The retina, optic nerve, and autonomic nerve supply controlling the pupil can be tested with a flashlight. Figure 14-1 shows the anatomy of the normal eye in the horizontal plane. The white, outer protective layer encasing most of the eyeball is called the sclera. It is modified anteriorly into a clear region called the cornea that consists of a specialized arrangement of collagen fibrils with an overlying, stratified, squamous epithelial layer. In the posterior two thirds of the eye the inner surface of the sclera is lined with a vascular and pigmented layer called the choroid. Interior to the choroid is the retina, the layer containing the photoreceptors. As light passes through the transparent cornea, it undergoes some bending that will ultimately help to focus it on the retina. After passing through the cornea, the light enters a compartment called the anterior chamber (see Figure 14-1). The anterior chamber and the posterior chamber are filled with a clear, waterlike fluid called aqueous humor that supplies important nutrients to the cornea (as well as the lens). Separating the anterior and posterior chambers is a diaphragm of varying size called the iris. The iris is a pigmented structure containing dilator and constrictor smooth muscle fibers arranged to vary the diameter of the pupil, the hole in the iris through which light passes on its way to the retina. The size of the pupil regulates the amount of light entering the eye. Behind the iris is the lens. The lens is suspended in the eye by suspensory ligaments (known as zonular fibers) which attach to the lens and to the ciliary body, a muscular structure near the base of the iris. As discussed below, the lens provides variable focusing power, in contrast to the fixed cornea. Behind the lens is a chamber filled with a gelatinous fluid called the vitreous humor. Because of the viscosity of this fluid, the pressure generated by the aqueous humor, and the fairly inelastic nature of the sclera and cornea, the globe of the eye is basically spherical. The vitreous humor also contains phagocytic cells that can clear ocular debris capable of obstructing the light path. Behind the vitreous humor is the neural retinal layer where light is transduced into the electrical activity of neurons. The retina is interrupted at a point where axons of the retina’s ganglion cell layer, which travel across the inner surface of the retina, leave the eye on their way to the brain. This point, the optic disc, is a recognizable structure when the eye is examined with an ophthalmoscope (Figure 14-2). The interruption of the light-processing retina at the optic disc produces a blind spot, another name for the optic disc. The retinal ganglion cell axons leaving the eye at the optic disc give rise to the optic nerve (cranial nerve 2), a cranial nerve so rich in axons that there are more axons in both optic nerves than in all the dorsal roots of the spinal cord. Also visible through the ophthalmoscope, on the surface of the retina, are the retinal blood vessels (see Figure 14-2). This network of arteries and veins enters the retina at the optic disc and provides much of the nutrition to the retina. Vessels of the choroid, that enter it after piercing the sclera near the optic disc, provide the remaining nutrition to the retina. Examination of retinal vessels often provides valuable clues to abnormalities elsewhere in the cardiovascular system. Figure 14-3 shows the process of accommodation, whereby the lens adds extra focusing power by changing its shape. The lens of the eye is made up of an elastic lens capsule containing laminae of lens fibers that are arranged like the layers of an onion. Given this anatomy, if the eye’s lens were taken out of the eye, the lens would assume a spherical shape, principally due to the elasticity of its capsule. When suspended in the relaxed eye, however, the suspensory ligaments pull on the equator of the lens, causing it to flatten in its anterior-posterior dimension. This flattened, less convex lens causes less refraction (bending) of light rays and allows the focus onto the retina of objects more than 20 feet away. To focus the image of objects closer to the eye, however, the lens must assume a more spherical, convex shape. This is accomplished by contraction of the ciliary muscle of the ciliary body. This contraction causes the ciliary muscle to move antero-centripetally (forward and inward), which results in a relaxation of tension on the suspensory ligaments. The result, given the inherent elasticity of the lens capsule, is a more spherical lens with more light refraction that focuses onto the retina the image of nearer objects. The more the ciliary muscle contracts, the more spherical the lens becomes. Given its elegant architecture, the retina, the neural portion of the eye, is capable of considerable processing of the visual image before the information is transmitted toward the brain. The vertebrate retina consists of five major cell types: photoreceptor cells, bipolar cells, horizontal cells, amacrine cells, and ganglion cells (Figure 14-4). As in the cerebral and cerebellar cortices, the arrangement of these component cells is fairly consistent across the retina, suggesting a basic underlying processing mechanism and giving the retina a layered histology. However, local variations in the density of some cell types and in synaptic architecture account for particular functional variations within the retina. There are two types of retinal photoreceptor cells: rods and cones (see Figure 14-4). Both rods and cones make direct synaptic connection with the interneurons called bipolar cells, which connect the receptors with the ganglion cells. Ganglion cell axons traverse the inner surface of the retina and converge at the optic disc to leave the eye as the optic nerve, sending action potentials to the brain. Modifying the flow of information at the synapses among the photoreceptors, bipolar cells, and ganglion cells are two interneuron cell types: the horizontal cells and the amacrine cells (see Figure 14-4). The horizontal cells mediate lateral interactions among the photoreceptors and bipolar cells. The amacrine cells mediate lateral interactions among the bipolar cells and the ganglion cells. The retinal ganglion cells are located in the inner retina (closer to the vitreous humor), whereas the photoreceptor cells (rods and cones) are located in the outer retina (closer to the choroid; see Figure 14-4). Therefore, throughout most of the retina, light rays travel through ganglion cells, bipolar cells, amacrine cells, and horizontal cells before reaching the photoreceptors. Although these inner neurons are unmyelinated (the ganglion cell axons become myelinated on leaving the eye) and therefore relatively transparent, they still cause some distortion of light rays. The fovea, an area that demarcates the central retina in many primates, is designed to minimize this distortion. This sloping pit is formed as the neural tissue near the inner surface of the central retina is pushed aside, permitting light rays to have a less obstructed path to the outer retina. Distortion is least in the center of the fovea, in an area called the foveola, where light rays have almost unobstructed access to the photoreceptors (Figure 14-5). This is functionally significant because it allows light to have a less distorted path to the region of the retina associated with the highest visual acuity (ability to discern detail). The optic disc is just nasal to the fovea. In animals that rely heavily on acute, daylight vision, there is a dark melanin pigment in the epithelial layer between the photoreceptors and the choroid. This pigment absorbs light that has passed by the photoreceptors without stimulating them. If such light were reflected back into the retina, the sharpness of the visual image would be blurred. In nocturnal animals and most domestic mammals, however, there is a patch of reflective material within the choroid called the tapetum (see Figure 14-2). When the tapetum is present, the region of the epithelial layer that overlies it does not contain the dark, light-absorbing pigment noted above. This arrangement facilitates the reflection of non-absorbed light back onto the retina allowing it to make optimal use of what light it receives, but at the expense of visual acuity. Reflection of light off the tapetum causes the familiar “night shine” from nocturnal animals’ eyes.
The Visual System
The Eye’s Anatomy Is Adapted to the Eye’s Role as a Visual Receptor Organ
Through the Process of Accommodation, the Lens Changes Shape to Focus Images from Various Distances onto the Retina
The Vertebrate Retina Consists of Five Major Cell Types
In Some Species the Fovea Minimizes Distortion of Light Compared with Other Areas of the Retina
Tissue Layers Behind the Retina Principally Absorb Light, or Can Reflect Light, Depending on the Species’ Habits
Stay updated, free articles. Join our Telegram channel

Full access? Get Clinical Tree
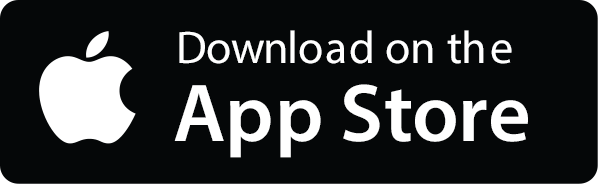
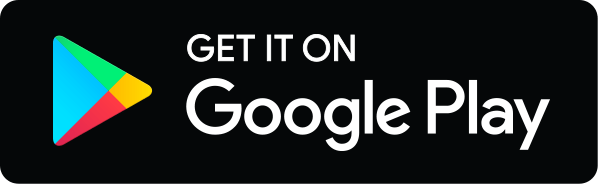