1. Body movement is the result of contraction of skeletal muscle across a movable joint. 2. There are several levels of organization in any skeletal muscle. 3. Action potentials on the sarcolemma spread to the interior of the cell along the transverse tubules. 4. The action potential on the sarcolemma is indirectly coupled to the contraction mechanism through the release of Ca2+ from the sarcoplasmic reticulum. 5. The sliding of actin along the myosin molecule results in physical shortening of the sarcomere. 6. Most skeletal muscle fibers can be classified as either fast-contracting or slow-contracting fibers. 7. Muscles change their strength of contraction by varying the number of active motor units or the rate of motor unit activation. 8. The electromyogram is the clinical measurement of the electrical behavior within a skeletal muscle. 9. The structure of cardiac and smooth muscle differs from that of skeletal muscle. 10. The role of Ca2+ ions in excitation-contraction coupling in cardiac and smooth muscle is different than in skeletal muscle. Skeletal muscle consists of a central, fleshy, contractile muscle “belly” and two tendons, one on each end of the muscle. The muscle and its tendons are arranged in the body so that they originate on one bone and insert on a different bone while spanning a joint. As the muscle contracts, shortening the distance between the origin and insertion tendons, the bones move in relation to each other, bending at the joint (Figure 6-1). When activated by a motor nerve, a skeletal muscle can only shorten. Most joints have one or more muscles on both sides, either to decrease its angle (flexion) or to increase its angle (extension). Figure 6-2 illustrates the levels of organization in a typical skeletal muscle. Each muscle belly seen during dissection is made up of differing numbers of muscle cells (usually called muscle fibers) that span the several inches between the origin and insertion tendons. The fibers range between 5 and 100 µm in diameter and contain multiple nuclei, multiple mitochondria, and other intracellular organelles. The outer limiting membrane of the fiber is called the sarcolemma. It consists of a true cell membrane, called the plasma membrane, and an outer polysaccharide layer that attaches to the tendons at the cells’ extremities. Each muscle fiber is innervated by only one motor neuron, with the neuromuscular junction region located near the middle of the fiber, relative to the ends. Each muscle fiber is made up of successively smaller subunits (see Figure 6-2). Each fiber contains several hundred to several thousand myofibrils arranged in parallel along its length, like a handful of spaghetti. Each myofibril is made up of a linear series of repeating sarcomeres, the basic contractile units of the muscle fiber, which can number in the tens of thousands. The sarcomere has a disk at each end called the Z disk. The sarcomere contains several types of large protein molecules responsible for muscular contraction, many of which are polymerized. Numerous thin protein filaments, called actin, are attached to the Z disks and extend toward the center of the sarcomere, similar to parallel fingers pointing at each other. Each actin filament consists of two intertwined, helical strands of actin protein and two such strands of tropomyosin protein, all wound together as a larger helical complex (see Chapter 1 and Figure 1-5). Also located intermittently along the tropomyosin-actin strand are complex globular protein molecules called troponin that can bind tropomyosin and actin and that have an affinity for calcium (Ca2+) ions. Suspended between and parallel to the actin thin filaments are thicker filaments of myosin protein polymers (Figure 6-3). A myosin molecule contains a tail of intertwined helices and two globular heads that can bind both adenosine triphosphate (ATP) and actin (see Figures 1-3 and 1-4). Approximately 500 myosin heads of a thick myosin filament form cross-bridges that interact with actin to shorten the sarcomere as the myosin heads flex and relax. The sarcomere also contains a large protein, titin, that helps to maintain the side-by-side relationship of actin and myosin, as well as the resting length during relaxation. Beneath the plasma membrane of the muscle cell lies the sarcoplasmic reticulum, an intracellular storage organelle that forms a reticulated network around the myofibrils (Figure 6-4). This extensive storage sac sequesters Ca2+ ions in relaxed muscle and is analogous to the smooth endoplasmic reticulum in other cells. Located perpendicular to the long axis of the muscle fiber are tubes of plasma membrane formed by periodic invaginations of the sarcolemma (see Figure 6-4). These transverse tubules, or T tubules, traverse the diameter of the muscle fiber, similar to a flexible drinking straw passing perpendicularly through the handful of spaghetti (myofibrils) noted earlier. The T tubules snake around the myofibrils, forming junctions with the network of sarcoplasmic reticulum that surrounds the myofibrils (Figure 6-5). These tubules are filled with extracellular fluid and are important because they allow the electrically excitable plasma membrane of the muscle fiber to carry the depolarization of the action potential to the interior of the fiber. Skeletal muscle cells have a resting membrane potential, as do neurons, and the muscle cell membrane can be depolarized by synaptic transmission at the neuromuscular junction (see Chapter 5). At this junction, the acetylcholine released by the motor neuron activates nicotinic acetylcholine receptors on the sarcolemma of the muscle cell. The resulting depolarization is sufficient to open enough voltage-gated sodium (Na+) ion channels, also found at the junctional sarcolemma (see Figure 5-1), to trigger a muscle fiber action potential. Thus, it is at the sarcolemma of the neuromuscular junction that muscle fiber action potentials are generated. When an action potential is generated near the midpoint of the muscle fiber, it spreads in both directions along the length of the fiber by mechanisms similar to action potential spread in unmyelinated neuronal axons. In contrast to those on axons, however, action potentials on the sarcolemma are also transmitted to the interior of the muscle fiber along the T tubules (see Figure 6-5). This allows the action potential to reach the location of the sarcoplasmic reticulum even in the innermost regions of the muscle fiber. The consequences of the action potential’s arrival at the location of the sarcoplasmic reticulum are critical for the coupling of excitation (the action potential) with contraction (shortening) of the sarcomeres of the myofibrils. Whereas in the neuron a rise in cytoplasmic Ca2+ at the terminal is critical for initiating the process of transmitter release, a rise in Ca2+ in the muscle cell sarcoplasm (cytoplasm of a muscle cell) is critical for initiating contraction. At rest, Ca2+ ions are pumped out of the sarcoplasm and stored in the sarcoplasmic reticulum using an energy-dependent pump in conjunction with Ca2+ binding proteins within the sarcoplasmic reticulum. This leaves too low a concentration of Ca2+ in the sarcoplasm to trigger contraction. However, as an action potential spreads along the muscle fiber surface and into the fiber’s core along the T tubules, the depolarization arrives at the junction between the tubules and the sarcoplasmic reticulum (see Figure 6-5). The arrival of the action potential at this junction leads to the release of stored Ca2+ ions from the sarcoplasmic reticulum. These Ca2+ ions diffuse down their concentration gradient into the sarcoplasm, bathe the sarcomere, then trigger contraction. As the action potential passes, Ca2+ is pumped again into the sarcoplasmic reticulum, and relaxation results. This cycle of events is known as excitation-contraction coupling. The link between the action potential on the transverse tubule and Ca2+ release from the sarcoplasmic reticulum is mediated by voltage-gated Ca2+ channels on the T tubule and Ca2+-induced Ca2+ release channels on the sarcoplasmic reticulum (see Figure 6-5). In skeletal muscle a mechanical coupling of these two types of channels is thought to exist; action potential opening of the voltage-gated Ca2+ channels of the T tubule produces a direct configurational change in the Ca2+-induced Ca2+ release channels on the sarcoplasmic reticulum, allowing the stored Ca2+ ions to escape from the sarcoplasmic reticulum into the sarcoplasm. The movement of Ca2+ through the opened voltage-gated Ca2+ channels on the T tubule, and the Ca2+ sensitivity of the Ca2+-induced Ca2+ release channels plays a more important role in excitation-contraction coupling in cardiac muscle than in skeletal muscle (see later discussion).
The Physiology of Muscle
Body Movement Is the Result of Contraction of Skeletal Muscle Across a Movable Joint
There Are Several Levels of Organization in Any Skeletal Muscle
Action Potentials on the Sarcolemma Spread to the Interior of the Cell Along the Transverse Tubules
The Action Potential on the Sarcolemma Is Indirectly Coupled to the Contraction Mechanism Through the Release of Ca2+ from the Sarcoplasmic Reticulum
Stay updated, free articles. Join our Telegram channel

Full access? Get Clinical Tree
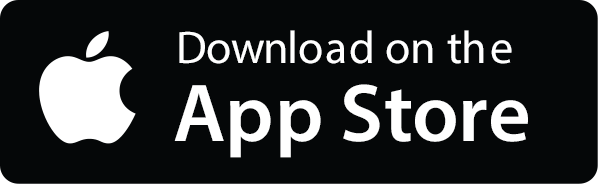
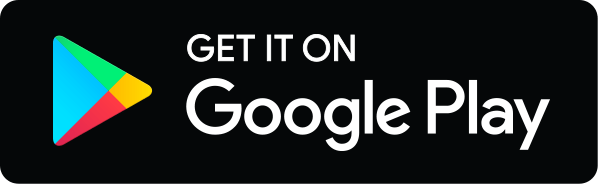