• T cells must be made tolerant to self-antigens. This may be accomplished through central tolerance whereby self-reactive T cells are killed. Alternatively, it may be achieved through peripheral tolerance whereby these T cells are “turned off” by inappropriate signaling. • B cells are much harder to tolerize than T cells. They are generally regulated by peripheral mechanisms and by the absence of T cell help. • Antigens stimulate immune responses, although very low or very high doses of antigen may cause tolerance. • Antibodies tend to regulate antibody production through negative feedback mechanisms. This can prevent the successful vaccination of newborn animals as a result of maternal immunity. • Immune responses may also be controlled by the activities of regulatory T cells (Treg cells). These Treg cells secrete cytokines such as IL-10 and TGF-β. • Another T cell subset, Th17 cells, regulates inflammation by secreting a cytokine called interleukin-17 (IL-17). • The immune system and the central nervous system are closely interconnected and influence each other. The adaptive immune system is a sophisticated defense system. It can recognize and respond to foreign invaders and can learn from the experience so that the body responds faster and more effectively when exposed to the invader a second time. There is, however, a risk associated with this—the risk of collateral damage. One of the reasons why the adaptive immune system is so complex is that much effort must be put into ensuring that it will only attack foreign or abnormal tissues and will ignore normal healthy tissues. As might be anticipated, many different mechanisms minimize the chances of developing autoimmunity. In addition, immune responses are regulated to ensure that they are appropriate with respect to both quality and quantity (Figure 20-1). Support for this suggestion came from observations on chimeric calves. In 1945 Owen noted that when cows are carrying twin calves, blood vessels in the two placentas commonly fuse. As a result, the blood of the twins intermingles freely, and bone marrow stem cells from one animal colonize the other. Each calf is born with a mixture of blood cells, some of its own and some originating from its twin. In dizygotic (nonidentical) twins, this is called a chimera. These “foreign” blood cells persist indefinitely because each chimeric calf is fully tolerant to the presence of its twin’s cells (Figure 20-2). Burnet and Fenner suggested that this could only happen because each calf was exposed to the foreign cells early in fetal life during a period when lymphocytes become tolerant upon encountering antigens. Cells from an unrelated calf would be rejected normally if administered after birth. Subsequent studies have shown that self-tolerance is of two types, central and peripheral. In central tolerance, immature self-reactive lymphocytes within the thymus, bursa, or bone marrow either die or alter their receptor specificity. In peripheral tolerance, mature lymphocytes that encounter self-antigens either die, are turned off, or are suppressed by Treg cells. By reconstituting lethally irradiated mice with T or B cells derived from normal or tolerant donors, tolerance can be shown to occur in both cell populations. However, their susceptibility to peripheral tolerance differs. T cells can be made tolerant rapidly and easily within 24 hours and remain in that state for more than 100 days (Figure 20-3). In contrast, B cells develop tolerance in about 10 days and return to normal within 50 days. Tolerance will result if there are no functional T cells with receptors that can bind self-antigens (Figure 20-4). Although the body generates an enormous diversity of TCRs, far fewer receptors are actually used by mature T cells than might be anticipated. Several processes serve to limit receptor diversity. First, the mechanisms used to generate diverse TCRs inevitably result in the production of nonfunctional receptors. For example, two thirds of possible gene arrangements will be out of frame. Cells with these nonfunctional TCRs undergo apoptosis. As T cells mature within the thymus, positive selection ensures that the cells that recognize self-MHC molecules survive. At this point, however, the cells whose receptors bind too strongly to self-antigens die by apoptosis (Figure 20-5). The timing and extent of this apoptosis depend on the affinity of the TCR for a self-antigen. T cells that bind self-antigens strongly die earlier and more completely than weakly binding cells. Thus the T cells that eventually leave the thymus have been purged of dangerous, self-reactive cells. When the antigen receptors of a developing T cell bind to self-antigens, another strategy employed to prevent autoimmunity is receptor editing (Chapter 34). Although cell maturation stops when it leaves the thymus, the RAG genes remain active and as a result, V(D)J recombination continues. Consequently, the TCR genes continue to diversify, and changed receptors are expressed on the cell surface. This process is called receptor editing. If a cell successfully edits its receptors, its maturation can proceed. Failure to do so will result in its apoptosis. This is a potentially hazardous process since it permits the development of self-reactive T cells that have not undergone careful selection within the thymus. As pointed out in Chapter 14, binding of an antigen to a TCR is by itself insufficient to trigger T cell responses. Indeed, occupation of the TCR in the absence of co-stimulation causes tolerance. For example, protein solutions normally contain some aggregated molecules. These aggregated molecules are readily taken up and processed by dendritic cells and thus are highly immunogenic. If a solution of such a protein, such as bovine γ-globulin, is ultracentrifuged so that all the aggregates are removed, then the aggregate-free solution will induce anergy. This is due to the lack of co-stimulation from antigen-presenting cells (APCs) (Figure 20-6). Very high doses of an antigen can induce a form of clonal anergy called immune paralysis (Figure 20-7). The high doses of the antigen probably bypass APCs, reach the Th cell receptors directly, and in the absence of co-stimulation trigger anergy. Because BCRs undergo random somatic mutation within germinal centers, self-reactive B cells can still develop in secondary lymphoid organs. These cells will not, however, make autoantibodies if APCs and helper T cells are absent or if Treg cells are active (Figure 20-8). This is not, however, a foolproof method of preventing self-reactivity. In the absence of T cell help, B cells may be activated by pathogen-associated molecular patterns (PAMPs) such as bacterial lipopolysaccharide (LPS), flagellins, or unmethylated CpG DNA acting through their toll-like receptors (TLRs). B cells may also be activated by either cross-reacting epitopes or a foreign carrier molecule stimulating nontolerant helper T cells (Figure 34-2). Tolerance is not the only mechanism of immune regulation employed by the body. The magnitude of immune responses must also be regulated. An inadequate immune response may lead to immunodeficiency and increased susceptibility to infection. An excessive immune response may result in the development of allergies or autoimmunity (Chapters 28 and 34). Failure to control the lymphocyte proliferation that occurs during immune responses may permit development of lymphoid cell tumors. Failure to control the immune response to the fetus may lead to abortion (Chapter 32). The immune responses must therefore be carefully regulated to ensure that they are appropriate in both quality and quantity. As might be anticipated, many different control mechanisms exist. The nature of the immune response may vary in different parts of the body as a result of processing by different dendritic cell populations. Langerhans cells seem especially suited for promoting T cell responses, whereas follicular dendritic cells prime B cells. DC1 cells are optimized to present antigens to Th1 cells, whereas DC2 cells present antigens to Th2 cells. Adjuvants also influence the type of immune response through their effects on APCs (Chapter 23). Thus, lipids conjugated to protein antigens commonly induce cell-mediated responses rather than antibody production and localize in T cell rather than B cell areas of lymphoid tissues.
Regulation of Adaptive Immunity
Tolerance
T Cell Tolerance
Central T Cell Tolerance
Negative Selection.
Receptor Editing.
Peripheral T Cell Tolerance
Clonal Anergy.
B Cell Tolerance
Peripheral B Cell Tolerance
Control of Immune Responses
Antigen Regulation of Immune Responses
Antigen Processing and Immune Regulation
Stay updated, free articles. Join our Telegram channel

Full access? Get Clinical Tree
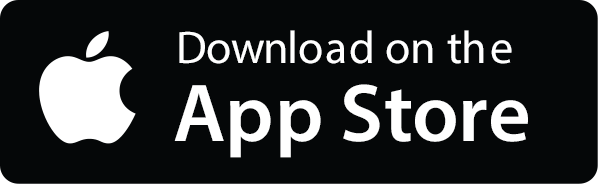
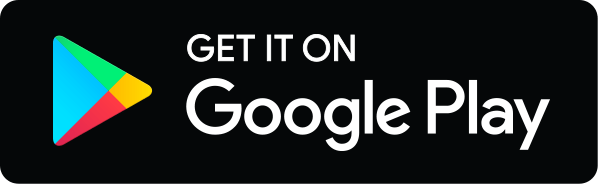