1. Because normal cardiovascular function is essential for life and health, a practical understanding of cardiovascular function and dysfunction is vital to the veterinary clinician. 2. Cardiovascular dysfunctions sometimes reflect primary cardiovascular disturbances or diseases, but more often they are secondary consequences of noncardiovascular disturbances or diseases. 3. Substances transported by the cardiovascular system include nutrients, waste products, hormones, electrolytes, and water. 4. Two modes of transport are used in the cardiovascular system: bulk flow and diffusion. 5. Because diffusion is very slow, every metabolically active cell in the body must be close to a capillary carrying blood by bulk flow. 6. The pulmonary and systemic circulations are arranged in series, but the various organs within the systemic circulation are arranged in parallel. 7. Cardiac output is the volume of blood pumped each minute by one ventricle. 8. The perfusion pressure for the systemic circulation is much greater than the perfusion pressure for the pulmonary circulation. 9. Each type of blood vessel has physical properties suited to its particular function. 10. Blood is a suspension of cells in extracellular fluid (plasma). 11. The cellular component of blood includes red blood cells, white blood cells, and platelets. 12. Most of the oxygen in blood is carried in chemical combination with the protein hemoglobin within red blood cells. We now take for granted that the cardiovascular system is a circulatory system, not a tidal system. However, the circularity of the cardiovascular system is precisely what makes it difficult to understand. It has no clear beginning or ending, and disturbances in one part of the cardiovascular system end up affecting all other parts as well. In recognition of this complexity, Chapters 18 to 26 are written with the goal of identifying the most basic and important concepts of normal cardiovascular function and explaining them in a way that best prepares the reader to understand, diagnose, and treat cardiovascular dysfunction (cardiovascular disease). The remainder of this chapter reviews the general features of the cardiovascular system. Chapters 19 to 25 discuss the various elements of the cardiovascular system in detail. Chapter 26 summarizes cardiovascular function and dysfunction by describing the overall effects of heart failure, hemorrhage, and exercise. It is important to distinguish between perfusion pressure difference and transmural pressure difference (usually shortened to transmural pressure). Transmural means “across the wall,” and transmural pressure is the difference between the blood pressure inside a blood vessel and the fluid pressure in the tissue immediately outside the vessel (transmural pressure equals inside pressure minus outside pressure). Transmural pressure is the pressure difference that would cause blood to flow out of a vessel if a hole were poked in the vessel wall. Transmural pressure is also called distending pressure, because it corresponds to the net outward “push” on the wall of a blood vessel. Figure 18-1 emphasizes the distinction between perfusion pressure and transmural pressure. To understand more fully how the two types of transport (bulk flow and diffusion) are used in the cardiovascular system, consider the transport of oxygen from the outside air to a neuron in the brain. With each inspiration, fresh air containing oxygen (O2) moves by bulk flow through progressively smaller airways (trachea, bronchi, and bronchioles) and finally enters the alveolar air sacs (Figure 18-2, A). The thin walls separating alveoli contain a meshwork of capillaries (see Figure 18-2, B). Blood flowing through these alveolar capillaries passes extremely close (within 1 µm) to the air in the alveoli (see Figure 18-2, C). The blood in an alveolar capillary has just returned from the body tissues, where it gave up some of its oxygen. Therefore the concentration of oxygen in alveolar capillary blood is lower than the concentration of oxygen in alveolar air. This concentration difference causes some oxygen to diffuse from the alveolar air into the capillary blood. As it leaves the lungs, each 100 mL of oxygenated blood normally carries 20 mL of oxygen. About 1.5% of this oxygen is carried in solution; the other 98.5% is bound to the protein hemoglobin within the erythrocytes (red blood cells). The oxygenated blood moves by bulk flow from the lungs to the heart. The heart pumps this oxygenated blood out into the aorta, and from there it is distributed via a complex system of branching arteries to all parts of the body (including the brain and skeletal muscles, as illustrated in Figure 18-2). Capillaries in the brain bring a bulk flow of oxygenated blood very close to each brain neuron (see Figure 18-2, D). Metabolic processes within the neurons consume oxygen, so the oxygen concentration inside neurons is low. The gradient of oxygen concentration between the capillary blood (high) and the neurons (low) provides the driving force for oxygen to diffuse first from the blood into the interstitial fluid and then into the neurons. Figure 18-2, E, shows a capillary carrying bulk flow of blood past a skeletal muscle cell (muscle fiber). Oxygen moves by diffusion from the capillary blood into the muscle interstitial fluid and then into the muscle cell, where it is consumed in the metabolic reactions that provide energy for muscle contraction. The oxygen consumption of a skeletal muscle depends on the severity of its exercise; at a maximum, oxygen consumption may reach levels 40 times greater than the resting level. Because of its tremendous metabolic capacity, muscle tissue has an especially high density of capillaries. In fact, several capillaries are typically arrayed around each skeletal muscle fiber. This arrangement provides more surface area for diffusional exchange than would be possible with a single capillary and brings the bulk flow of blood extremely close to all parts of each skeletal muscle cell. Coronary artery disease and cerebrovascular disease are encountered more often in human medicine than in veterinary medicine. In contrast, cardiac disease (dysfunction of the heart muscle or valves, as distinguished from disease of the coronary arteries) is encountered more often in veterinary medicine than in human medicine. Therefore, Chapters 19 to 26 place more emphasis on cardiac physiology than on vascular physiology. As shown in Figure 18-3, blood is pumped from the left ventricle into the aorta. The aorta divides and subdivides to form many arteries, which deliver fresh, oxygenated blood to each organ of the body, except the lungs. The pattern of arterial branching that delivers blood of the same composition to each organ is called parallel. After blood passes through the capillaries within individual organs, it enters veins. Small veins combine to form progressively larger veins, until the entire blood flow is delivered to the right atrium by way of the venae cavae (pleural of vena cava, includes both superior vena cava and inferior vena cava). The blood vessels between the aorta and the venae cavae (including the blood vessels in all organs of the body except the lungs) are collectively called the systemic circulation. From the right atrium, blood passes into the right ventricle, which pumps it into the pulmonary artery. The pulmonary artery branches into progressively smaller arteries, which deliver blood to each alveolar (pulmonary) capillary. Blood from pulmonary capillaries is collected in pulmonary veins and brought to the left atrium. Blood then passes into the left ventricle, completing the circuit. The blood vessels of the lungs, including the pulmonary arteries and veins, constitute the pulmonary circulation. The pulmonary circulation and the heart are collectively termed the central circulation. The pulmonary circulation and the systemic circulation are arranged in series; that is, blood must pass through the pulmonary vessels between each passage through the systemic circuit. In one pass through the systemic circulation, blood generally encounters only one capillary bed before being collected in veins and returned to the heart, although a few exceptions to this rule exist. One exception occurs in the splanchnic circulation, which supplies blood to the digestive organs. As shown in Figure 18-3, blood that leaves the gastric, splenic, or mesenteric capillaries enters the portal vein. The portal vein carries splanchnic venous blood to the liver, where the blood passes through another set of capillaries before it returns to the heart. This arrangement of two systemic capillary beds in series is called a portal system. The splanchnic portal system allows nutrients that have been absorbed from the gastrointestinal tract to be delivered directly to the liver. There the nutrients are transformed for storage or allowed to pass into the general circulation. The liver also receives some blood directly from the aorta through the hepatic artery. The kidneys also contain a portal system. As shown in Figure 18-3, blood enters a kidney by way of a renal artery and passes through two sets of capillaries (called glomerular and tubular) before returning to the venous side of the systemic circulation. Large amounts of water, electrolytes, and other solutes are filtered out of the blood as it passes through the glomerular capillaries. Most of this filtered material is subsequently reabsorbed into the bloodstream as it flows through the peritubular capillaries. The remainder becomes urine. The kidneys use the renal portal system to adjust the amounts of water, electrolytes, and other critical solutes in the blood. A third portal system is found in the brain and is important in the control of hormone secretion by the pituitary gland. After traversing capillaries in the hypothalamus, blood enters portal vessels that carry it to the anterior pituitary gland (adenohypophysis) and to another set of capillaries (see Figures 33-16 and 33-17). As blood traverses the hypothalamic capillaries, it picks up several signaling chemicals that control the release of pituitary hormones. When this blood reaches capillaries in the anterior pituitary gland, these substances diffuse out of the bloodstream and into the pituitary interstitial fluid, where they act on pituitary cells to increase or decrease their secretion of specific pituitary hormones. This system is called the hypothalamic-hypophyseal portal system.
Overview of Cardiovascular Function
Because Normal Cardiovascular Function Is Essential for Life and Health, a Practical Understanding of Cardiovascular Function and Dysfunction Is Vital to the Veterinary Clinician
Two Modes of Transport Are Used in the Cardiovascular System: Bulk Flow and Diffusion
Because Diffusion Is Very Slow, Every Metabolically Active Cell in the Body Must Be Close to a Capillary Carrying Blood by Bulk Flow
The Pulmonary and Systemic Circulations Are Arranged In Series, But the Various Organs Within the Systemic Circulation Are Arranged in Parallel
Stay updated, free articles. Join our Telegram channel

Full access? Get Clinical Tree
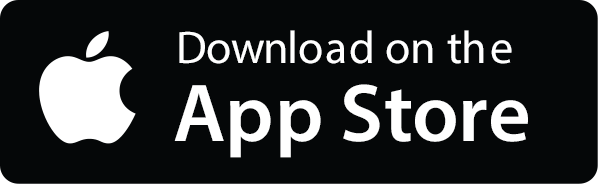
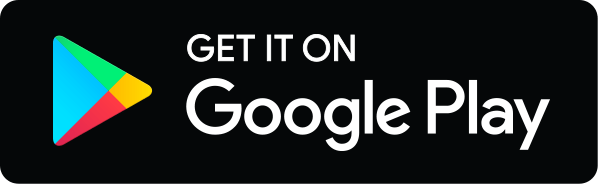