• The immune system is fully formed at birth but has never been used. Hence all adaptive immune responses in the newborn are slow primary responses. • Newborn mammals are temporarily protected against infection by transfer of immunoglobulins from their mother. • Immunoglobulins are derived from the mother either by direct transfer across the placenta as in primates, or by ingestion of immunoglobulin-rich colostrum immediately after birth. • Failure of this passive transfer may result in the newborn animal suffering from overwhelming infections. • Milk provides a constant supply of immunoglobulin (immunoglobulin A [IgA] in most species) that helps protect the newborn against intestinal infections. • Vaccination protocols in newborn mammals must take into account their inability to generate antibody responses in the presence of persistent maternal immunity. When a mammal is born, it emerges from the sterile uterus into an environment where it is immediately exposed to a host of microorganisms. Its surfaces, such as the gastrointestinal tract, acquire a complex microbial flora within hours. If it is to survive, the newborn animal must be able to control this microbial invasion. In practice, the adaptive immune system takes some time to become fully functional, and innate mechanisms are responsible for the initial resistance to infection. In some species with a short gestation period, such as mice, the adaptive immune system may not even be fully developed at birth. In animals with a long gestation period, such as the domestic mammals, the adaptive immune system is fully developed at birth but cannot function at adult levels for several weeks. The complete development of adaptive immunity depends on antigenic stimulation. The proper development of B cells and B cell receptor (BCR) diversity requires clonal selection and antigen-driven cell multiplication (Chapter 15). Thus, newborn mammals are vulnerable to infection for the first few weeks of life. They need assistance in defending themselves at this time. This temporary help is provided by the mother in the form of antibodies and possibly T cells. The passive transfer of immunity from mother to newborn is essential for survival. The development of the immune system in the mammalian fetus follows a consistent pattern. The thymus is the first lymphoid organ to develop, followed closely by the secondary lymphoid organs. B cells appear soon after the development of the spleen and lymph nodes, but antibodies are not usually found until late in fetal life, if at all (Box 21-1). The ability of the fetus to respond to antigens develops very rapidly after the lymphoid organs appear, but all antigens are not equally capable of stimulating fetal lymphoid tissue. The immune system develops in a series of steps, each step permitting the fetus to respond to more antigens. These steps are driven by a gradual increase in the use of gene conversion or somatic mutation to increase antibody diversity. The ability to mount cell-mediated immune responses develops at the same time as antibody production. T cell receptor (TCR) diversity is also limited in the fetus and neonate, and their cytokine production may be low. This may simply be due to their lack of exposure to foreign antigens. Although the gestation period of the cow is 280 days, the fetal thymus is recognizable by 40 days postconception. The bone marrow and spleen appear at 55 days. Lymph nodes are found at 60 days, but Peyer’s patches do not appear until 175 days (Figure 21-1). Blood lymphocytes are seen in fetal calves by day 45, IgM+ B cells by day 59, and IgG+ B cells by day 135. The time of appearance of serum antibodies depends on the sensitivity of the techniques used. It is therefore no accident that the earliest detectable immune responses are those directed against viruses, using highly sensitive virus neutralization tests. Fetal calves have been reported to respond to rotavirus at 73 days, to parvovirus at 93 days, and to parainfluenza 3 virus at 120 days. Fetal blood lymphocytes can respond to mitogens between 75 and 80 days, but this ability is temporarily lost near the time of birth as a result of high serum steroid levels. T cell subpopulations are present in calves at levels comparable to adults, but B cell numbers increase significantly during the first 6 months after birth. The effects of the timing of viral infection are well seen with bovine viral diarrhea virus (BVDV). If a cow is infected early in pregnancy (up to 50 days), she may abort. On the other hand, infections occurring between 50 and 120 days, before the fetus develops immune competence, lead to asymptomatic persistent infection because the calves develop tolerance to the virus (Figure 21-2). These calves are viremic yet, because of their tolerance, fail to make antibodies or T cells against the virus. Some of these calves may show minor neurologic problems and failure to thrive, but many are clinically normal. If the cow is infected with BVDV between 100 and 180 days postconception, calves may be born with severe malformations involving the central nervous system and eye, as well as jaw defects, atrophy, and growth retardation. Vaccines containing modified live BVDV may have a similar effect if administered at the same time. Calves infected after 150 to 180 days’ gestation are usually clinically normal. BVD viruses occur in two distinct biotypes: cytopathic and noncytopathic. (The name derives from their behavior in cell culture, not their pathogenicity in animals.) Noncytopathic strains do not trigger type I interferon (IFN) production and therefore can survive in calves and cause persistent infections. Cytopathic strains induce IFN production and cannot cause persistent infection. These cytopathic strains, however, do cause mucosal disease (MD), a severe enteric disease leading to profuse diarrhea and death (Figure 21-3). Mucosal disease develops as a result of a mutation in a nonstructural viral gene that changes the BVDV biotype from noncytopathic to cytopathic while the animal fails to produce neutralizing antibodies or T cells. The cytopathic strain can spread between tolerant animals and lead to a severe mucosal disease outbreak. Both cytopathic and noncytopathic viruses can be isolated from these animals. Recombination may also occur between persistent noncytopathic strains and cytopathic strains in vaccines and lead to MD outbreaks. Although some of the lesions in MD are attributable to the direct pathogenic effects of BVDV, glomerulonephritis and other immune complex–mediated lesions also develop. The reasons for this are unclear but may reflect superinfection or the production of non-neutralizing antibodies. Because persistently infected calves can reach adulthood and breed, it is possible for BVD infection to persist indefinitely within carrier animals and their progeny. Epidemiological studies suggest that between 0.4% and 1.7% of cattle in the United States are persistently infected in this way. The development of the newborn immune system is largely driven by the intestinal microflora (Chapter 22). In its absence, “germ-free” mammals fail to fully develop their mucosal lymphoid tissues. The commensal flora generates a complex mixture of pathogen-associated molecular patterns (PAMPs) that act through epithelial cell toll-like receptors (TLRs). Likewise, microbial antigens are taken up by dendritic cells and presented to CD4+ T cells. These signals collectively promote the functional development of the immune system. The intestinal microflora also plays a key role in determining any Th1 or Th2 bias in immune function. This is the basis of the “hygiene hypothesis,” the idea that the development of allergies is influenced by microbial exposure early in life (Chapter 28).
Immunity in the Fetus and Newborn
Development of the Immune System
Specific Animal Immune Systems
Foal
Calf
The Immune System and Intrauterine Infection
Immune Response of Newborn Mammals
Role of the Intestinal Microflora
< div class='tao-gold-member'>
Stay updated, free articles. Join our Telegram channel

Full access? Get Clinical Tree
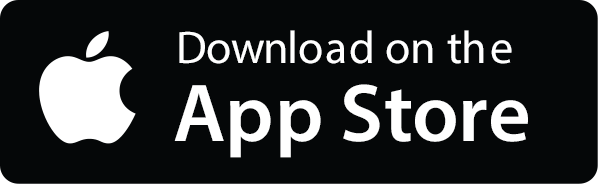
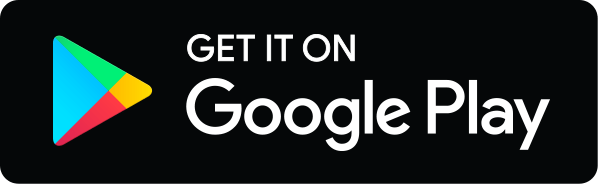