Fig. 1.
Lesion models. Illustration of the conditions of the rodent brains, in particular the striatum and substantia nigra, in healthy unlesioned animals (a) and the different lesion paradigms (b–h). The rat models of stereotaxic 3-NP (b), sequential administration of 6-OHDA + QA (c) as well as simultaneous stereotaxic application of 6-OHDA + QA (d) show unilateral lesions of the relevant areas striatum and substantia nigra to different degrees, whereas systemic application of 3NP (e), sequential injection of MPTP followed by 3-NP (f), sequential administration of 3-NP followed by MPTP (g) or simultaneous injection of MPTP and 3-NP (h) in mice lead to bilateral brain damage with different severity.
Motor behavior tests of 3-NP versus sham lesioned control groups revealed impaired paw reaching in the animals.
Investigating the drug-induced rotation behavior, ipsiversive amphetamine induced rotations as well as ipsiversive rotations following apomorphine were reported. As will be explained later, the PD 6-OHDA lesion models lead to contraversive rotations after apomorphine injections. These differences can be explained by the striatal lesion and suggest that the partial reduction of dopaminergic neurons after 3-NP lesion does not significantly influence the rotation pattern.
The behavioral impairment was directly correlated to the reduction of striatal surface area.
In one study by Brundin and colleagues, they further analyzed the effects of alpha-phenyl-tert-butyl nitrone and found no improvement of the paw-reaching deficits after 3-NP lesion. Also neurorestorative approaches using embryonic neuronal grafts have been tried but a significant amelioration of behavioral deficits could not be achieved (27, 28).
2.2.2 Sequential Double-Toxin, Double-Lesion Model
A study by Wenning and colleagues (29) used 6-OHDA and QA in a sequential protocol (Fig. 1c). For this purpose, the two neurotoxins were stereotaxically injected unilaterally, but in one group 6-OHDA into the medial forebrain bundle (MFB) and 8 weeks later, QA into the striatum, and in the second group the protocol started with striatal injections of QA followed by secondary 6-OHDA injections into the MFB 8 weeks afterwards. Behavioral assessment tests, including the paw-reaching test or the side falling and stepping test, were performed before and after the administration of the second neurotoxin. Extensive histological analysis showed that in animals injected with QA first, the remaining striatal surface area was significantly reduced compared to animals with primary 6-OHDA nigral lesions. These findings correlate well with the relevant behavioral findings like the stepping test, where the contralateral backhand stepping pattern showed significantly higher impairment in the group with primary striatal QA lesion. Both experimental groups also presented severe motor impairment in the paw-reaching test. Loss of TH-positive neurons in the SN could be observed in both groups without significant differences between the different injection protocols. Microglial activation and astrogliosis are also present features of this lesion model. Ipsiversive rotation followed amphetamine injections have been observed; however, apomorphine induced contraversive rotations were absent. Investigators should keep in mind that potential differences in the response to apomorphine and amphetamine may be due to slight variations of the size and locus of the caused lesion (30). The ipsiversive rotation followed amphetamine administration is likely due to a stimulated dopamine release on the intact side (31–33).
Kollensperger et al. also performed a cylinder test with animals treated after this sequential protocol (34). They found that after 6-OHDA lesion a clear preference of wall contacts toward the ipsilateral paw representing the unlesioned side of the striatum and administration of dopaminergic agents could neutralize this asymmetry. The additional QA lesion created 8 weeks later, further shifted the paw use toward the ipsilateral side. The fact that the primary lesion decreases the effects of the secondary neurotoxin is suggested to be due to a release of neurotrophic factors such as BDNF or GDNF induced by the first lesion (29, 35–37). Transplantation of embryonic striatal cells could partly restore the dopamine response in regard to rotation behavior as well as complex motor behavior (21, 38).
Furthermore, this double-toxin model has been used to test the effects of riluzole and minocycline. The glial activation inhibitor minocycline, which has been shown to have neuroprotective effects in an MPTP PD mouse model, did not lead to any beneficial effects in the sequential double-toxin, double-lesion model (39, 40). Riluloze is a therapeutic agent that is already FDA approved as treatment for amyotrophic lateral sclerosis (ALS) (41). The investigators report significant amelioration of motor impairment and a reduction in the striatal lesion size; however, they did not find significant differences in the number of dopaminergic neurons in the SNc between control and riluzole-treated animal groups.
In summary, this model can be used to reproduce degeneration of the dopaminergic nigrostriatal and GABAergic striatopallidal pathways; however, it should be mentioned that the almost complete nigral and striatal degeneration does not reflect the early stages of human pathology with specific loss of ventrolateral nigral dopaminergic neurons and dorsolateral striatal target neurons (42–44). Therefore, the next model listed here might be more helpful to reproduce the early stage SND.
2.2.3 Simultaneous Double-Toxin, Double-Lesion Model
A method to generate a lesion model for MSA-P, more specifically mimicking the disease pattern of early-stage SND to closely reflect the human disease process, uses simultaneous striatal injections of 6-OHDA and QA (Fig. 1d) (35). The lateral striatum was chosen as target region for the surgery. This area receives projections from the motor cortex, afferent its dopaminergic innervation is restricted to the nigra and it has been reported previously that it is the functional homologue to the human sensorimotor putamen (45–48). The rotation behavior of this model was different compared to the sequential double-toxin double-lesion model. Due to the simultaneous 6-OHDA and QA injections, the characteristic 6-OHDA ipsiversive amphetamine and contraversive apomorphine induced rotation pattern was modified compared to single toxin control groups. This finding can be explained by the loss of dopaminergic responsiveness due to the destruction of striatal postsynaptic outflow (30, 49, 50). Also present in this model is astrogliosis and a significant striatal surface reduction, more restricted to the caudal than rostral striatum, and the loss of TH-immunopositive neurons in the SN was restricted to the medial part of the substantia nigra pars compacta after the stereotaxic administration of the two neurotoxins.
Summing up, this model with its partial dopaminergic and striatal degeneration is a valuable model to investigate treatment options for early-stage SND.
2.3 Neurotoxic Lesions in Mice
2.3.1 Single-Toxin Double Lesion
Intraperitoneal injections of 3-NP lead to reversible striatonigral lesions (see Fig. 1e) (51). After the intoxication phase of 5 consecutive days, animals showed improving scores in evaluation of the motor behavior 3–5 days after the last 3-NP injections and a partial recovery after 7–10 days. The motor phenotype of this model ranges from early motor signs like slowing or transient hindlimb dystonia at low doses (two injections per day, doses increased over time from 10 to 50 mg/kg, total cumulated dose 340 mg/kg) to permanent hindlimb dystonia, hindlimb clasping, truncal dystonia or global postural abnormalities at higher doses (two injections per day, doses increased over time from 20 to 80 mg/kg, total cumulated dose 560 mg/kg). Even though low doses of 3-NP did not cause circumscribed lesions in the experiment performed by Fernagut et al., high dose administration led to lateral lesions or an almost complete destruction of the striatum with significant reduction of the striatal volume. That study also reported correlations between motor deficits and the remaining striatal volume, and also between the motor behavioral impairment and the estimated loss of cresyl violet-nissl stained neurons in the lateral striatum post-intoxication. They further used MRI to detect the 3-NP-induced lesion in vivo. The mentioned study described a useful SND model showing signs of age-dependent oxidative stress, apoptotic striatal neuronal cell death, and histopathologically circumscribed lesions.
2.3.2 Sequential Double-Toxin Double Lesion
MPTP is a meperidine-derivate that leads to a selective degeneration of dopaminergic neurons in the SN and further degeneration of the striatal outflow pathways (52, 53).
Stefanova et al. (54) tested the sequential, systemic application of MPTP and 3-NP. They analyzed two different procedures (a) a primary MPTP lesion, followed by 3-NP application compared to (b) a primary striatal 3-NP lesion followed by secondary administration of MPTP. In both approaches, the neurotoxins were injected twice a day intraperitoneally, MPTP for 2 consecutive days, and 3-NP over a period of 2 weeks. Primary MPTP lesions were shown to protect against the neurotoxic effects of 3-NP, which well correlates with results from rat models, where the primary striatonigral 6-OHDA injection had protective effects against QA lesions. Other previous studies also showed that dopamine depletion protects striatal neurons against various types of neurotoxins (55, 56).
The behavioral analysis of these double-toxin models revealed no significant difference compared to the administration of 3-NP or MPTP alone regarding locomotor activity.
On the other hand, when applying 3-NP prior to MPTP, Stefanova et al. report reduced toxicity of MPTP to nigral dopaminergic neurons, which is similar to the findings in rat models where striatal QA lesions were protective against nigral 6-OHDA toxicity.
In summary, these different administration patterns resulted in different lesion patterns and should be carefully chosen depending on the study design. The MPTP + 3-NP model pathology can be described as degeneration of SNc with a relative preservation of the striatum as represented in Fig. 3.1f, whereas the 3-NP-MPTP model had a more severe pathology including neuronal loss and glial pathology in the SNc as well as striatum, which can be seen in Fig. 1g.
2.3.3 Simultaneous Double-Toxin Double-Lesion
One approach described by Tison and coworkers (57, 58) is a mouse model with simultaneous systemic injections of the two neurotoxins MPTP and 3-NP (Fig. 1h).
To assess the behavioral characteristics of this model, they used motor-tests such as the rotarod, beam-walking or the pole-test and were able to show significant motor impairment and altered gait-patterns due to striatal dysfunction. Also an open-field test has been performed and detected a reduced amount of rearing in the MPTP + 3-NP treated group, as well as reduced mean and maximum velocity indicating lower activity levels.
Histologically, they report bilateral striatal lesions with increased neuronal loss in the medial part of the striatum, significant reduction of striatal volume and significant loss of dopaminergic neurons in the mid and caudal levels of the substantia nigra pars compacta. Furthermore, when comparing MPTP + 3-NP groups to 3-NP or MPTP treated animals alone, they found a significantly increased number of astrocytes and also different distribution patterns showing that the simultaneous application of the two neurotoxins leads to a more severe pathology. This model can serve as a useful tool to mimic SND; however, after the 9-day intoxication phase, a recovery has been observed and after 3 weeks, the motor impairment was not detectable.
The same group later tested whether the antiglutamateric drug riluzole can serve as a useful treatment option (58). They report dose-dependent neuronal rescue in histopathological analyses, reduced cell loss in the nigral and dorsolateral striatum, and less astroglial activation.
This model has further been used in a study to investigate the potential treatment with human mesenchymal stem cells (59). For this purpose, mice received 9 days of double-toxin treatment before the bone-marrow-derived stem cells were administered via the tail vein on the following day. The behavioral as well as histopathological outcome of this study showed improvement in the stem-cell treated group compared to controls. Also upregulation of several cytokines including p-Akt, Bcl-2 and decreased levels of Bax and cytochrome C were detected.
2.4 Neurotoxic Lesions in Primates
2.4.1 Sequential Double-Toxin, Double-Lesion Model
A model described by Tison and colleagues uses a sequential, systemic intraperitoneal administration of MPTP followed by 3-NP injections in primates (60, 61). They report l-Dopa-responsive parkinsonian phenotype after MPTP injection, increased motor impairment and loss of l-Dopa response after secondary application of 3-NP. Histologically, they found severe dopaminergic cell loss in the SNc and bilateral subtotal striatal lesions.
This model can serve as a useful tool to investigate the human pathology of l-Dopa unresponsive striatonigral degeneration in primates.
2.5 Transgenic Models in Mice
The neurotoxin models have been of great importance for researching MSA disease pathophysiology and they can still be applied depending on the experimental question. For therapeutic approaches such as neurorestorative cell transplantation trials, the lesion models can provide the necessary test bed. However, their utility is limited in neuroprotective studies that require insights into pathogenesis.
The great advantage of transgenic MSA compared to neurotoxin models is, that transgenic models reproduce the overexpression, misfolding, and aggregation of human α-synuclein or a functional deficit of the degradation mechanisms at normal expression levels, resulting in the formation of GCIs and therefore leading to the characteristic neuronal loss (62). These transgenic MSA models can be used to identify intervention therapies by targeting different steps of the a-synuclein linked neurodegeneration. However, since it is still not fully understood how α-syn and GCIs cause neuronal loss and not all transgenic models reproduce striatonigral lesions or a motor phenotype, more research will be required in this field to generate research models even closer to the human disease that not only feature motor pathology but also autonomic nervous system degeneration.
Since some transgenic MSA modes do not reproduce the characteristic neuronal loss, neurotoxins can be used to lesion the relevant areas and mimic the full-blown pathology of human MSA.
2.5.1 α-Syn Overexpressing Mouse Model Using PLP-Promoter
A well-established method has been developed by Stefanova et al. (63). They used the mitochondrial inhibitor 3-NP in an α-syn overexpressing transgenic mouse model to induce oxidative stress. The overexpression of α-syn in this model is linked to the proteolipid protein (PLP) promoter that is only active in oligodendrocytes and therefore leads to specific overexpression of human wild-type α-syn in oligodendrocytes (64).
Histological analysis of this PLP-h-α-syn model showed half-moon or sickle-shaped GCI-like insoluble α-syn positive inclusions in oligodendrocytes throughout the white matter. Further significant loss of dopaminergic neurons in the SN, microglial activation, and shortened stride length have been reported. Since this transgenic model did not reproduce the neuropathology of MSA, the effects of 3-NP injections were investigated.
The findings reveal that already low doses of 3-NP, which did not have effects in the control group, led to motor impairment and loss of striatal neurons in PLP-h-α-syn mice. In addition, a significantly higher reduction of striatal volume has detected in transgenic animals with low dose 3-NP. In PLP-h-α-syn animals, a significant reduction of TH-immunoreactive neurons in the SNc was found at baseline levels, indicating that the α-syn pathology alone, is sufficient to cause this neuronal loss. Injections of 3-NP further increased this neuronal loss in PLP-h-α-syn animals. Significant neuronal loss after the injections of 3-NP was found in cerebellar purkinje cells, locus coeruleus, pontine nuclei, and inferior olivary nuclei in PLP-h-α-syn animals, but not in wt controls. Stefanova et al. further describe widespread astrogliosis throughout the striatum of 3-NP treated. Moreover, investigating the distribution and amount of activated microglia revealed significantly higher numbers in the PLP-h-α-syn group, further increased by 3-NP injections, but not or to a much lesser degree in control mice.
This model was the first to represent the full-blown human MSA-pathology including SND, astrogliosis, microglial activation as well as the characteristic oligodendroglial α-syn inclusions.
For this reason, the model has been used to test potential neuroprotective effects of a possible therapeutic agent. Rasagiline has been used in the transgenic model with additional 3-NP lesion and shown to have dose-dependent neuroprotective effects (65).
2.5.2 α-Syn Overexpressing Mouse Model Using MBP-Promoter
The other transgenic MSA model that has been tested with additional 3-NP lesions, works under the control of the myelin basic protein (MBP) promoter (66). Since this promoter also has specific expression pattern in oligodendrocytes, this model too presents with overexpression of α-syn in oligodendrocytes (67). Motor impairment could be shown via rotarod and pole-test, and α-syn expression detected in the basal ganglia, brain stem, and cerebellum.
To lead this model even closer to the human pathology, 3-NP has been administered twice daily over 6 days to cause the characteristic striatonigral lesions. They were able to show that the solubility pattern of α-syn changed after 3-NP injection. MBP-h-α-syn animals which received 3-NP treatment had significantly higher amounts of nitrated and oxidized α-syn in the insoluble urea fraction and decreased amounts of soluble nitrated, as well as oxidized α-syn in the TBS fraction compared to vehicle treated MBP-h-α-syn mice, whereas the levels of total and phosphorylated α-syn were not affected by 3-NP.
Histologically they found that the α-syn expression was significantly increased in the basal ganglia and frontal cortex of MBP-h-α-syn animals with 3-NP treatment compared to the saline-treated group. Behavioral tests to investigate potential motor deficits have been performed. In the pole test, as well as the grip test, significant motor impairment could be detected; the MBP-h-α-syn + 3-NP group had the worst motor scores. The difference between non-transgenic mice receiving saline and MBP-h-α-syn + saline was not significant, illustrating that the severity of motor deficits strongly depends on the 3-NP administration.
In summary, like the PLP-h-α-syn + 3-NP model, this model can serve as a useful tool for evaluating potential therapeutic agents, not only testing neuroprotective or neurogenic factors, but it can also find application in investigating the pathways of protein overexpression, misfolding, aggregation, oligodendrogliosis, and finally neurodegeneration (63, 66). Understanding these disease mechanisms will be of great importance for developing therapeutics interfering and inhibiting these mechanisms.
2.5.3 Other Transgenic Models
Currently there are 2 more transgenic mouse models of MSA, one using the phosphodiesterase (CNP) promoter to constitutively overexpress h-α-syn and the second model overexpresses α1B-adrenergic receptor (α1B-AR), leading to an MSA-P-like phenotype (9, 11). However, these models have not yet been analyzed with regard to 3-NP sensitivity.
3 Methods
The methods to evaluate and characterize the relevance of different animal models vary from histological quantifications using classical immunohistochemistry, molecular techniques such as western blots, and behavioral tests to investigate the motor impairment, which play a very important role in analyzing models for movement disorders (12).
The major behavioral tests that have been used to evaluate MSA models are shown in Table 1, and the key features of each test are described in the following paragraphs.
Table 1
Behavioural tests
Functional domain | Behavioral test | Key references |
---|---|---|
General neurological state | General assessment | |
Locomotor activity | Open-field test | |
Rotation test | ||
Balance and motor coordination | Cylinder test | (34) |
Rotarod | ||
Balance beams | ||
Gait analysis | ||
Climbing | ||
Grip strength | ||
Stepping test | ||
Skilled limb use | Reaching test | |
Staircase test |
3.1 General Neurological State
3.2 Locomotor Activity
3.2.1 Open-Field Test
Several parameters of motor activity can be obtained from open-field experiments, including global locomotion, maximum or mean velocity, or the number of rearings. MSA models often present with decreased activity and velocity and also a reduction of rearing events (35, 54, 57, 58).
< div class='tao-gold-member'>
Only gold members can continue reading. Log In or Register a > to continue
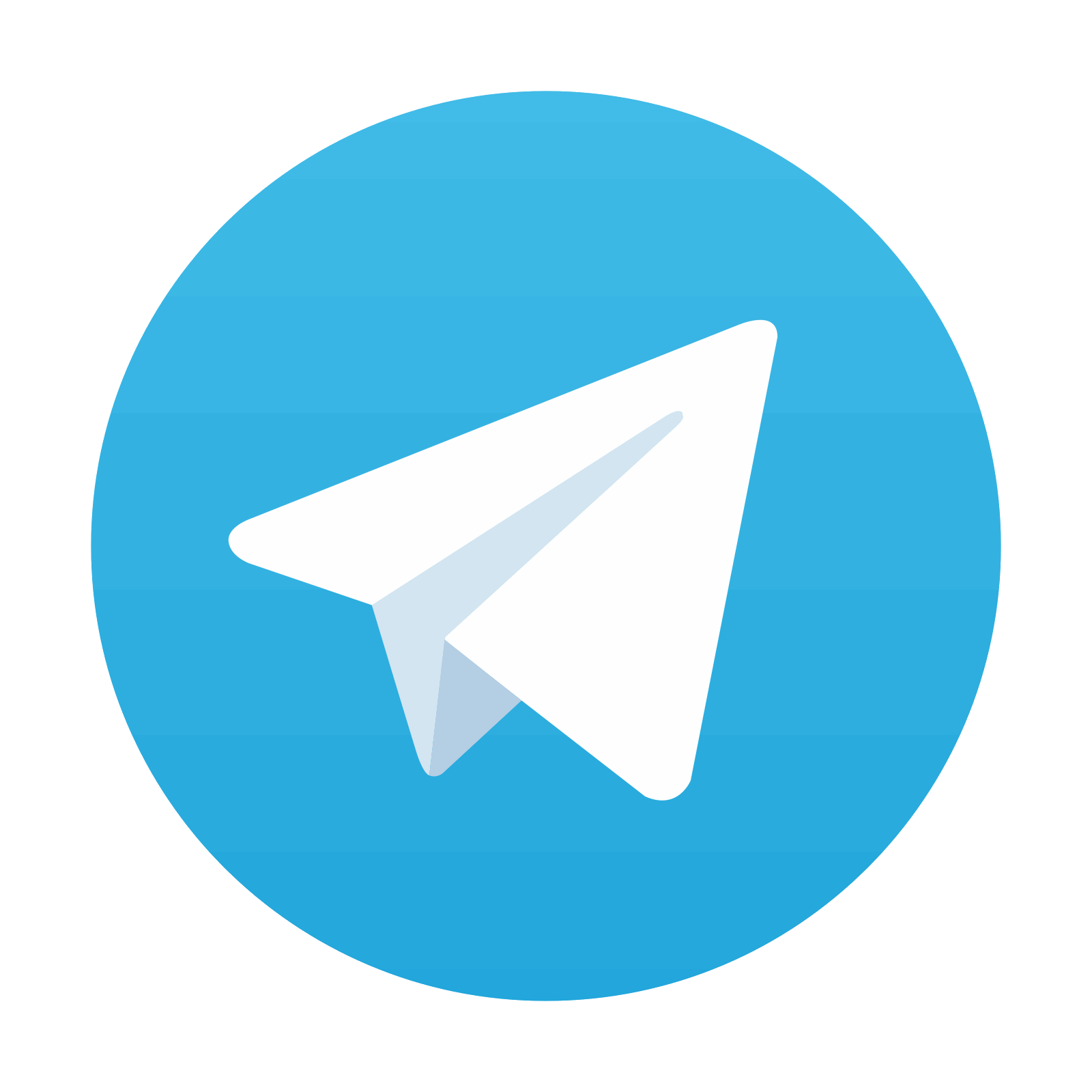
Stay updated, free articles. Join our Telegram channel

Full access? Get Clinical Tree
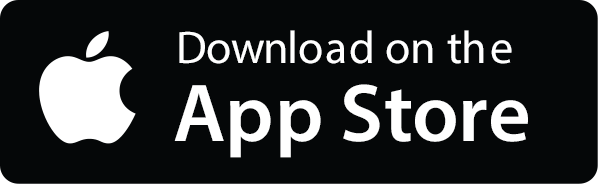
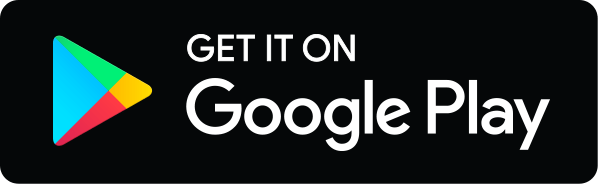