Target protein or molecule
Target cells
Reason
NeuN
Mature neurones
To visualise loss of striatal neurones
Cresyl violet or Nissl stain
All cells
To visualise the cell loss
DARPP-32
Striatal neurones
To visualise loss of medium spiny striatal projection neurones
Calbindin
Striatal neurones
To visualise loss of medium spiny striatal projection neurones
TH
Dopaminergic neurones
To visualise loss of nigrostriatal dopaminergic input
ChAT or AChE
Cholinergic interneurones
To visualise loss of the giant aspiny striatal cholinergic interneurons in the striatum
GFAP
Astrocytes
To identify astrocytic response to the lesion
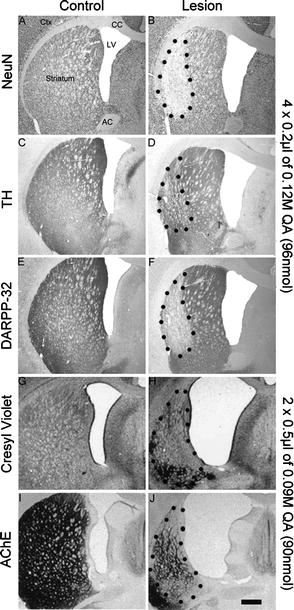
Fig. 1.
Photomicrographs illustrating the effects of unilateral dorsal striatal injections of quinolinic acid. Sections from the control and the lesion groups are typically stained with a selection of markers specific for neurones (NeuN; a–b), dopaminergic afferents (TH, tyrosine-hydroxylase; c–d), medium spiny neurones (DARPP-32; e–f), general cell marker (Cresyl Violet; g–h), or cholinergic interneurons (Acetylcholinesterase, AChE, i–j). The control sections show regular staining and no anatomical deformation. However, lesioned sections have enlarged ventricles, collapsed axons of passage, shrunk striatal tissue, a necrotic core and exhibit a general reduction of staining in the striatum. Interestingly, the injection of 4 × 0.2 μl of 0.12 M QA produced apparently less damage then the injection of 2 × 0.5 μl of 0.09 M QA. This suggests that the excitotoxic lesion achieved is a function not only of the concentration of the QA used but also of the volume and the distribution of the toxin. For more details see (34, 35). Scale bar = 1 mm.
The stained, free-floating sections are mounted on slides, dehydrated, cover slipped and subjected to image analysis. Typically, sections stained with a marker that clearly delineates the extent of the striatal cell loss, such as DARPP-32 or NeuN, are used to quantify the striatal areas that survived or were affected by the lesion. Volumetric analysis is carried out by measuring the lost and remaining striatal tissue on the ipsilateral side, the unlesioned striatal tissue on the contralateral side, and the lateral ventricles; these values are quantified on each consecutive section throughout the anterior-posterior axis of the dorsal striatum. The final volume measurements for the various parameters are calculated by taking into account the sum of the areas and correcting for section thickness and sample frequency: Volume (mm3) = Sum of areas (mm2) × Section thickness (μm) × Sample frequency).
3.5 Factors Influencing Lesion Outcome
Excitotoxins act through glutamatergic receptors present on striatal projection neurones, and as such, factors that interact directly or indirectly with this transmission system can mitigate or accentuate the excitotoxic mechanism. Furthermore, co-ordinate selection, dose, and volume injected are important parameters of the experimental design that need to be considered within the overall scientific rationale. A selection of these issues is discussed below.
3.5.1 Anaesthesia
The choice of anaesthesia used during excitotoxic surgery of the striatum is an important factor to consider as it can influence the lesion outcome. For example, ketamine, a non-competitive NMDA receptor antagonist and widely used anaesthetic agent, shares its target with QA, and hence can mitigate the excitotoxic damage by exerting a neuroprotective effect. It is known for over two decades that ketamine can inhibit and modulate the neurotoxic effect of QA lesions in a dosage-dependent manner (36, 37). Therefore, it is remarkable that it is still used today as an anaesthetic for QA lesion experiments where neurotoxicity is the primary objective, for example, in rodent models of HD (see (38) for review).
Early work that established the ketamine/QA combination in rodent models of HD did not recognise this as an issue when high dose of QA (e.g., 240 nmol) were being used (39). Due to the dose-dependent relationship between ketamine and QA, the neuroprotective interference of the two ligands can be overcome by either increasing the dose of QA or reducing the dose of ketamine. Controversially, the previously described profile of QA relating to its ability to achieve selective neuronal death akin to HD pathology, such as killing GABAergic medium spiny neurones while sparing somatostatin, neuropeptide Y, and NADPH-diaphorase immunoreactive striatal interneurons (30, 31) could be related to the lesion modifying aspect of ketamine and not to the inherent properties of the excitotoxin itself. Furthermore, ketamine is commonly used along with xylazine (Rompun), an alpha-2-adrenoreceptor agonist that works as a sedative and muscle relaxant (40), minimising side effects like tremor and muscle rigidity, while increasing the duration of anaesthesia by reducing its renal elimination (41, 42). Doing so, it may increase the bioavailability of ketamine thereby indirectly enhancing its neuroprotective effect, as has been suggested in a recent study where it was directly compared with isoflurane (38) (see Fig. 2).
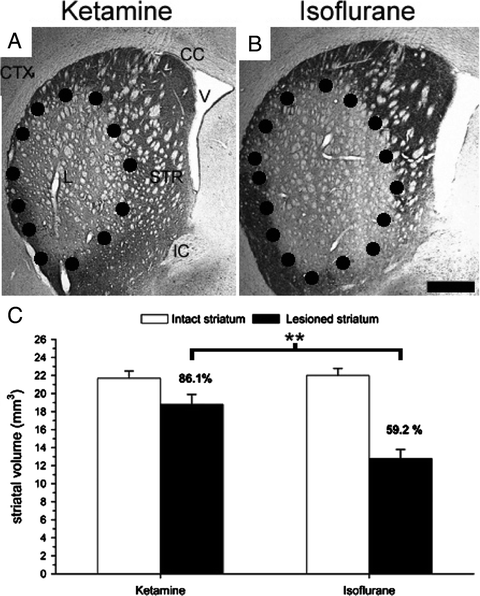
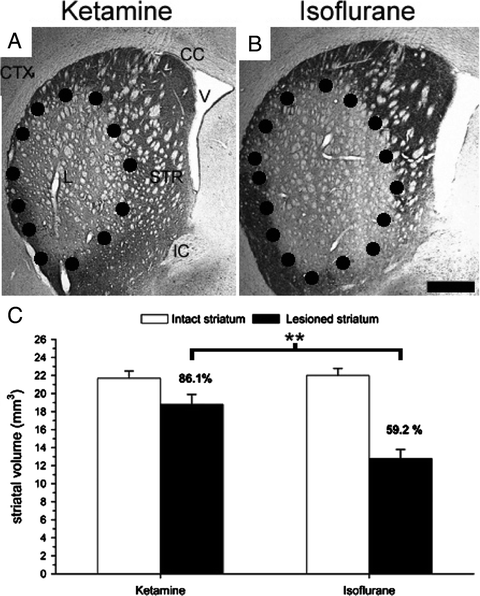
Fig. 2.
Photomicrographs showing typical coronal sections of DARPP-32 stained QA lesioned rat striatum when using ketamine (a) or isoflurane (b) as anaesthetic during the surgery. The dotted lines delineate the lesion area. Analysis confirmed a significant role of the anaesthesia used during the surgery on the eventual striatal damage underlining the neuroprotective effect of ketamine in the context of QA excitotoxic lesions (c). For more details, see (38). CTX cortex, CC corpus callosum, V ventricle, L lesion, STR striatum, IC internal capsule. Scale bar = 1 mm. **, p < 0.001.
Isoflurane is a gaseous anaesthetic that provides for a more predictable alternative and has become the anaesthesia of choice in many labs working with rodent excitotoxic lesion paradigms (43, 44). Although isoflurane has been linked with neuroprotective effects as well, the only study – known to the authors of this chapter – that directly investigated this issue found no interaction between isoflurane and NMDA receptors (45). Isoflurane provides a more rapid induction, greater control and rapid recovery from the anaesthetised state, which means less trauma and danger for the experimental animals. Ketamine, on the other hand, is cheaper, easily applied and does not require additional equipment. The control over the depth of the anaesthesia, however, is far less, the risk of overdosing is higher, and of course, there is the issue of inappropriate use in the context of models of excitotoxic neuronal death.
3.5.2 Lesion Placement: Motor and/or Cognitive Effects
Most common excitotoxic lesions in the striatum are unilateral and this consistently produces impairments in contralateral paw use, or deficits in responding in the contralateral space. Bilateral lesions can be too debilitating, especially if behavioural testing of the animals is planned. The somatotopic organisation of the cortex, and the topographically organised afferent and efferent connections of the striatum mirror the diverse nature of deficits observed following striatal lesions in the rat (46). For example, regional striatal lesions have demonstrated the differential effects of the lateral and medial striatal contribution to motor functions (47). Dorsolateral striatal lesions selectively produce skilled forelimb deficits, while dorsoventral striatal lesions impair both skilled forelimb use and tongue reaching. Medial striatal lesions, receiving inputs mainly from the auditory and visual cortex, do not affect either measurement (48–51). In a choice reaction-time task, lateral and medial striatal lesions induce ipsilateral response bias and increase latency in response initiation, respectively (52).
Non-motor, or cognitive behaviours can also be affected by appropriate targeting of the striatal excitotoxic lesion (53, 54). The striatum receives topographically organised projections from the whole of the neocortex, in particular the prefrontal cortex, providing a major system for the selection and initiation of cortically derived action plans. For example, habit, or procedural learning is characterised by gradual and stable acquisition of an association between a stimulus and a response, and is a form of memory believed to be mediated by the striatum where the sensory information is associated with a learned motor output (55–58). Lesions of the anteromedial area of the striatum, a region that receives its afferents predominantly from associative, auditory and visual cortices, induces deficits in delayed alternation and spatial navigation tasks, for example, but leaves visual discrimination performance intact; however, lateral, particularly ventrolateral striatal lesions destroying cortical afferents from sensorimotor areas, disrupt performance on complex visual stimulus–response habit tasks (24, 59).
3.5.3 Striatal Afferents
The excitotoxic lesion of the rodent striatum relies on the excessive stimulation of the glutamate receptors found on the distal portions of the dendritic spines of the medium spiny striatal neurones. Studies have shown that the extent of the QA-induced lesion can be influenced not only by the availability of the glutamate receptors – e.g., in the presence of a ketamine, a non-competitive NMDA receptor ligand – but also by the state of other striatal afferents such as the corticostriatal glutamatergic or the nigrostriatal dopaminergic inputs that form synapses equally on the distal areas of the dendritic spines (60).
The level of endogenous glutamate present in the striatum has a bearing on the extent of striatal lesion induced by excitotoxicity. For example, decortication, leading to the removal of the corticostriatal glutamatergic projection, protects the striatal cells from quinolinic acid-induced necrosis (14, 28). Conversely, glutamate levels within the striatum – and the sensorimotor cortex – have been shown to be higher on the side contralateral to an active, compared to an immobilised limb, and studies have indicated that the exclusive use of a limb can exaggerate the extent of tissue damage in rodent stroke model (61, 62), or following unilateral striatal lesion (44).
Similarly, removal of the nigrostriatal dopaminergic input by 6-OHDA lesion has been shown to significantly reduce the vulnerability of the striatum to subsequent QA lesion (63), and this is particularly important to recognise when trying to establish the double lesion animal model for Multiple System Atrophy (see Kuzdas and Wenning, this volume, ch. 3). Dopamine acting on striatal postsynaptic D1 and D2 receptors can modulate glutamate signalling and excitatory currents by, respectively, increasing or decreasing NMDA and AMPA currents in the striatal neurons (64, 65), which will impact on the overall cell activity and subsequent excitotoxic damage.
4 Conclusions
Despite the emerging transgenic animal models available to study basal ganglia diseases, producing striatal neuronal death using excitotoxic agents still enjoys strong scientific rationale. Excitotoxic lesions do not mimic the progressive degenerative processes that occur in Huntington’s disease or Multiple System Atrophy, for example; however, if the parameters are well chosen, they can recreate the cellular pathology at given stages of the disease with a high degree of authenticity. Once an excitotoxic striatal lesion model is established and characterised, it can open numerous research avenues looking into a multitude of scientific and clinical relevant issues such as neurodegeneration, neuroprotection, cell replacement therapy, circuit reconstruction and repair. The excitotoxic striatal lesions model has its limitations; but if the investigator makes use of it appropriately, it can serve as a highly powerful experimental tool to address scientific questions related to the structure and function of the basal ganglia.
References
1.
King B (1991) Comparison of electrolytic and radiofrequency lesion methods. In: Conn PM (ed), Methods in Neurosciences, Vol. 7: Lesions and Transplantation. Academic Press, New York, 90–96
2.
3.
4.
5.
Lowe CU, Zavon MR., Olney JW, Sharpe LG (1970) Monosodium glutamate: specific brain lesion questioned. Science 167: 1016–1017PubMedCrossRef
< div class='tao-gold-member'>
Only gold members can continue reading. Log In or Register a > to continue
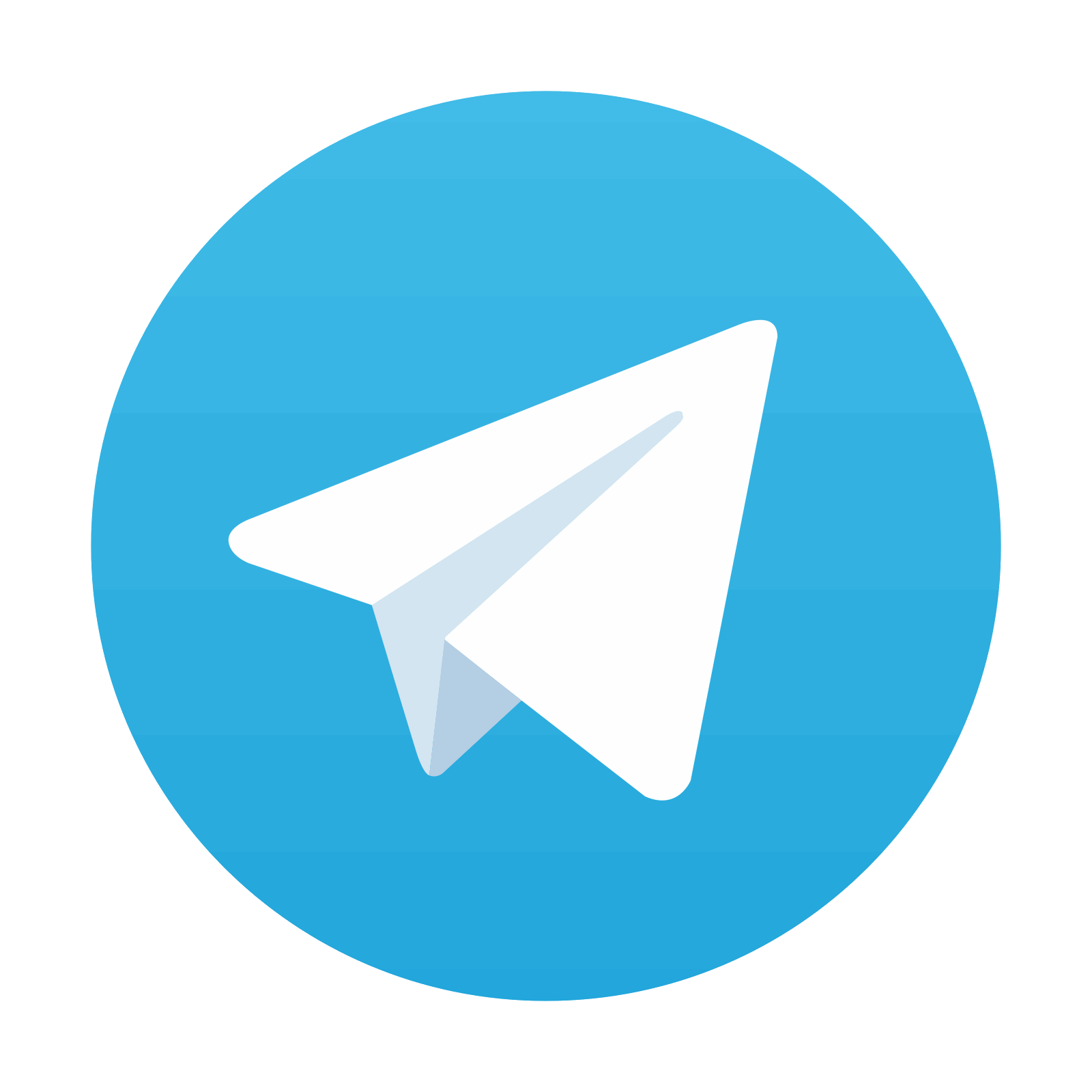
Stay updated, free articles. Join our Telegram channel

Full access? Get Clinical Tree
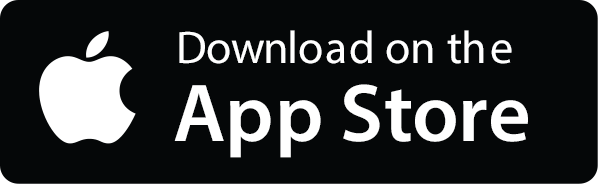
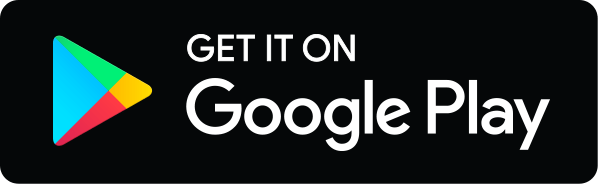