Fig. 1.
Climbing activity in C57Bl/6J mice at 6.5 weeks of age. Mice were tested either during dark phase, or light phase (not both). Mice climb more during their active phase (dark phase). However, gender differences are observed in climbing during the dark phase, which would necessitate large mixed gender group sizes, in order to observe genotype differences, or treatment effects. Larger mixed gender group sizes (or single gender groups) are not necessary if mice are tested during the day phase. Data are shown as mean ± SEM. Data were analyzed using a completely randomized ANOVA, followed by Fisher’s PSD post hoc tests. Effect of phase F(1,30) = 16.5, p < 0.001. ^p < 0.05 compared to male, dark phase. *p < 0.05 compared to male, dark phase. **p < 0.01 compared to female, dark phase. N = 6–10.
5.4 Wheel Running
Running wheels are widely used in research on circadian rhythms and are fully automated. They represent a significant initial investment but are valuable for preclinical drug testing because of the high power to detect differences between groups, the minimal investigator time involvement, and the wealth of information obtained. We have successfully developed the use of running wheels to assess motor deficits in both R6/2 and KI HD mice (44, 45, 100). Mice are placed in individual cages equipped with a running wheel (23 cm diameter, Mini Mitter Company Inc., Bend OR). Each rotation of the wheel is detected by a magnet and recorded by VitalView Data Acquisition Software V 4.0 (Mini Mitter Company Inc.), in 3 min bins. It is important to house running wheel cages in cabinets to minimize light and sound disturbance. Cabinets must be equipped with fans to allow air circulation, and light should follow the same pattern of light dark phases as in the vivarium. Running activity is recorded continuously and activity is recorded in light and dark phases. It is critical to only use mice of the same sex for the calculations as activity of females is different to that of males and also changes during the estrus cycle (45, 86, 87). Wheel running activity (speed of running) during dark and light phases can be calculated using ActiView V 1.2 (Mini Mitter Company Inc.). We have also analyzed patterns of activity over the dark (active) phase and found deficits in R6/2 and CAG140 KI mice (44, 45). In addition, by monitoring performance over time, it is possible to analyze motor learning using this test (45). Running wheel testing is highly amenable to therapeutic testing because of its high power to detect drug effects. For example, we have found that only 8 R6/2 transgenic mice are required to detect an improvement of 50% with 80% power (α = 0.05) at 4.5 weeks of age, while 5 male CAG140 homozygote KI mice are sufficient to detect a 50% improvement in performance at 6m of age (80% power, α = 0.05) (44, 45). Strain is also important to take into account with this test, as it can profoundly affect running performance. In our experience, B6129S4 mice are very poor runners (Fig. 2), while B6129/Sv (45) and B6CBA hybrids are extremely good runners (44).
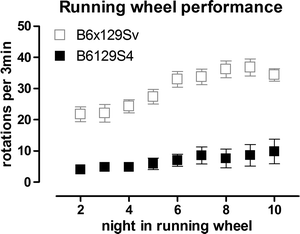
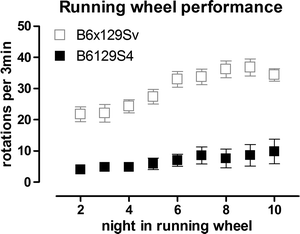
Fig. 2.
Illustration of the poor running activity in B6129S4 mice, compared with B6129Sv mice. B6129Sv mice were N3 on B6 background. Mice are wildtype. Data in gray(B6129Sv) are from Hickey et al. (45). Data are of mean ± SEM, and show mean running per 3 min bin, per night. Mice are housed individually in cages equipped with running wheels, as described in text. Data are collected automatically. These data demonstrate the importance of determining the suitability of each test for each strain as necessary.
5.5 Pole Test
The pole task is commonly used in models of PD (101, 102) but also detects profound anomalies in HD mice. For this test, mice are placed on a vertical pole (1 cm in diameter, 60 cm high). The spontaneous behavior for mice is to turn downwards and descend the pole. After habituation to the task in 2 trials per day for 2 days, on the third day mice are given 5 trials. The time taken to turn to face downwards (t. turn) is measured, in addition to the total time to complete the task (t. total) and the time taken to descend (t. descend), following the turn. We found a marked increase in the total time to complete the task at 4 months in the CAG140 KI mice (45) and this has now also been observed in several lines of different repeat length R6/2 mice (Cummings et al. submitted).
6 Conclusion
In conclusion, both simple but labor intensive (climbing cage, pole test) and almost or fully automated tests (open field, running wheels) can be used to detect early motor deficits in mouse models of HD. Using these tests does not require extensive prior training for the investigator but they require careful planning in term of phase of the day, age of the animals, and gender matching. We have preliminary data indicating that drug effects can be detected using these tests but a systematic evaluation of different lines of mice using the same tests in the same conditions is lacking. In the absence of this information, the use of a battery of tests is recommended with a selection of tests that have demonstrated the greatest sensitivity in detecting motor anomalies in HD models.
References
1.
The Huntington’s Disease Collaborative Research Group. (1993) A novel gene containing a trinucleotide repeat that is expanded and unstable on Huntington’s disease chromosomes. Cell 72: 971–983.
2.
Paulsen JS, Langbehn DR, Stout JC et al (2008) Detection of Huntington’s disease decades before diagnosis: the Predict-HD study. J Neurol Neurosurg Psychiatry 79: 874–880.
3.
Vonsattel JP, DiFiglia M (1998) Huntington disease. J Neuropathol Exp Neurol 57: 369–384.
4.
Harper PS (1996) Huntington’s Disease. London, Saunders.
5.
Lemiere J, Decruyenaere M, Evers-Kiebooms G et al (2004) Cognitive changes in patients with Huntington’s disease (HD) and asymptomatic carriers of the HD mutation–a longitudinal follow-up study. J Neurol 251: 935–942.
6.
Peinemann A, Schuller S, Pohl C et al (2005) Executive dysfunction in early stages of Huntington’s disease is associated with striatal and insular atrophy: a neuropsychological and voxel-based morphometric study. J Neurol Sci 239: 11–19.
7.
Watkins LH, Rogers RD, Lawrence AD et al (2000) Impaired planning but intact decision making in early Huntington’s disease: implications for specific fronto-striatal pathology. Neuropsychologia 38: 1112–1125.
8.
Craufurd D, Thompson JC, Snowden JS (2001) Behavioral changes in Huntington Disease. Neuropsychiatry Neuropsychol Behav Neurol 14: 219–226.
9.
Paulsen JS, Nehl C, Hoth KF et al (2005) Depression and stages of Huntington’s disease. J Neuropsychiatry Clin Neurosci 17: 496–502
10.
Tabrizi SJ, Langbehn DR, Leavitt BR et al (2009) Biological and clinical manifestations of Huntington’s disease in the longitudinal TRACK-HD study: cross-sectional analysis of baseline data. Lancet Neurol 8: 791–801.
11.
Walker FO (2007) Huntington’s Disease. Semin Neurol 27: 143–150.
12.
Dumas EM, van den Bogaard SJ, Ruber ME et al (2011) Early changes in white matter pathways of the sensorimotor cortex in premanifest Huntington’s disease. Hum Brain Mapp. doi: 10.1002/hbm.21205. [Epub ahead of print].
13.
Nopoulos PC, Aylward EH, Ross CA et al (2011) Smaller intracranial volume in prodromal Huntington’s disease: evidence for abnormal neurodevelopment. Brain 134: 137–142.
14.
Say MJ, Jones R, Scahill RI et al (2011) Visuomotor integration deficits precede clinical onset in Huntington’s disease. Neuropsychologia 49: 264–270.
15.
Stout JC, Paulsen JS, Queller S et al (2011) Neurocognitive signs in prodromal Huntington disease. Neuropsychology 25: 1–14.
16.
Tabrizi SJ, Scahill RI, Durr A et al (2011) Biological and clinical changes in premanifest and early stage Huntington’s disease in the TRACK-HD study: the 12-month longitudinal analysis. Lancet Neurol 10: 31–42.
17.
Gomez-Tortosa E, MacDonald ME, Friend JC et al (2001) Quantitative neuropathological changes in presymptomatic Huntington’s disease. Ann Neurol 49: 29–34.
18.
Politis M, Pavese N, Tai YF et al (2008) Hypothalamic involvement in Huntington’s disease: an in vivo PET study. Brain 131: 2860–2869.
19.
Rosas HD, Tuch DS, Hevelone ND et al (2006) Diffusion tensor imaging in presymptomatic and early Huntington’s disease: Selective white matter pathology and its relationship to clinical measures. Mov Disord 21: 1317–1325.
20.
< div class='tao-gold-member'>
Only gold members can continue reading. Log In or Register a > to continue
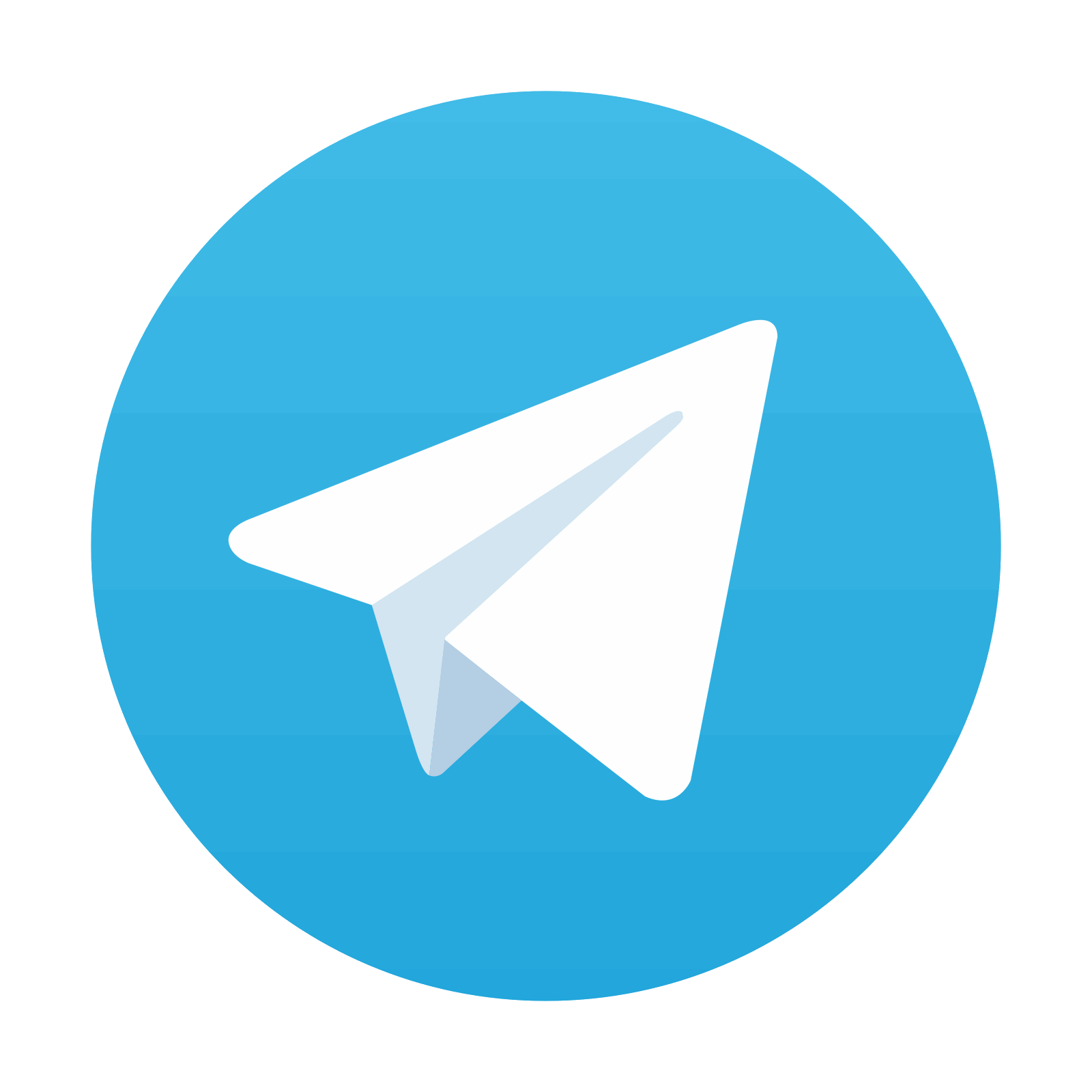
Stay updated, free articles. Join our Telegram channel

Full access? Get Clinical Tree
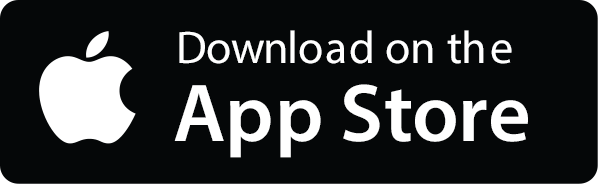
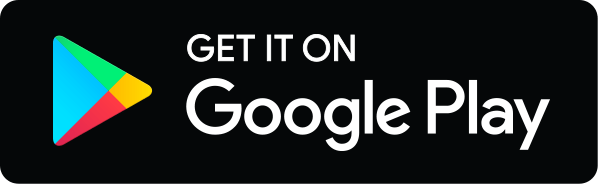