1. Buffers, lungs, and kidneys together maintain acid-base balance. 2. Acid excretion is achieved by proton secretion by tubule epithelial cells, buffering in the tubule fluid, and bicarbonate absorption. 3. Renal ammonia metabolism generates new bicarbonate and promotes acid excretion. 4. The proximal tubule has a high capacity for H+ secretion and bicarbonate reabsorption. 5. The thick ascending limb of Henle’s loop absorbs filtered bicarbonate. 6. The collecting duct determines the final urine pH. 7. The collecting duct can secrete protons, reabsorb bicarbonate, and generate acidic urine. 8. The collecting duct can secrete bicarbonate and generate alkaline urine. Buffering of the tubule fluid is necessary for efficient acid excretion. Buffers accept secreted H+ and minimize the decrease in tubule fluid pH that would otherwise follow rapid H+ secretion by the epithelial cells. In mammals the most important buffers are bicarbonate, phosphate, and ammonia (NH3); to a lesser extent, creatinine and citrate serve as luminal buffers. In birds, urates significantly contribute to titration of secreted acid. Figure 44-1 illustrates the removal of acid by intraluminal buffers. Renal ammonia metabolism is a major component in the maintenance of acid-base balance and is illustrated in Figure 44-2. In proximal tubule cells the amino acid glutamine is metabolized to produce NH4+. This process is called ammoniagenesis. The intracellular NH4+ enters the tubule fluid through secondary active transport by substitution for H+ on the Na+/H+ exchanger. Glutamine metabolism also produces new bicarbonate anions, which are transported across the basolateral plasma membrane. Thus proximal tubule ammoniagenesis enables bicarbonate production and absorption and distal delivery of ammonia. Renal ammoniagenesis is enhanced by acidosis and is an important renal response to an increase in the acid load. High NH3/NH4+ concentrations are enhanced and maintained in the medullary interstitium by a countercurrent multiplication system in the loops of Henle, similar to that described in Chapter 43. This creates a steep concentration gradient for NH3, which favors its movement into the medullary collecting duct. Until recently, the prevailing model for NH4+ excretion in the collecting duct was NH3 diffusion and trapping. The belief was that ammonia freely diffused across plasma membranes and into the luminal fluid where it bound protons, rendering it impermeant across plasma membranes and trapped in the luminal fluid. However, NH3, although not a charged molecule is a polar molecule, much like water, and it is now known that the lipid bilayer is relatively impermeable to NH3. In fact, specific ammonia transporters, the Rh glycoproteins, Rhcg and Rhbg, are present in plasma membranes of the majority of cell types in the collecting duct and facilitate transepithelial ammonia transport. These transporters are required for normal collecting duct ammonia transport and renal ammonia excretion and their abundance and subcellular distribution are regulated in accordance with physiologic conditions that increase renal ammonia excretion, such as acidosis. In addition, in the terminal inner medullary collecting duct, NH4+ is transported by substitution for K+ on the basolateral Na+,K+-ATPase.
Acid-Base Balance
Acid Excretion Is Achieved by Proton Secretion by Tubule Epithelial Cells, Buffering in the Tubule Fluid, and Bicarbonate Absorption
Renal Ammonia Metabolism Generates New Bicarbonate and Promotes Acid Excretion
Stay updated, free articles. Join our Telegram channel

Full access? Get Clinical Tree
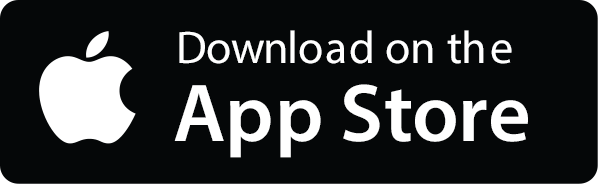
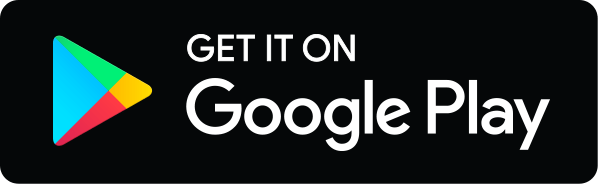