(1)
Department of Genetics, Case Western Reserve University, Cleveland, OH, USA
Abstract
Animal models of disease in genetically manipulated mice are powerful tools in medical research, including the study of dementia. The time and expense required to make genetically altered mice is considerable, and the importance of this investment is amplified by the long time course of most studies of dementia. Investigators need to be able to make informed choices about the different strategies for transgenics and gene targeting in order to minimize unwanted variation, and to maximize fidelity to the disease. In recent years, large genomic fragments stably cloned in well-characterized libraries, the means to manipulate their sequence, and the ability to make transgenic mice from these clones in inbred strains have increased greatly the power of the transgenic mouse. In addition, new embryonic cell lines from the C57BL/6 inbred strain of mice have become widely adopted for gene targeting, allowing knockins, knockouts, and conditional alleles to be established on the standard C57BL/6 background much more expeditiously than in the past. These methods, the time required, and the probability of success are reviewed with respect to mouse models of dementia.
Key words
Dementiatransgenicgene targetingknockoutrecombineeringinbred strainmice1 Introduction
Gene targeting and transgenic technologies have different strengths and weaknesses with respect to fidelity to the disease, minimized variation, and avoidance of unintended consequences. The generation of genetically manipulated mice for any purpose involves significant time and expense. This investment is amplified in the case of models of dementia because of the aging component in many dementia models. My goal in the following is to help the investigator make wise choices in selection of technologies such that unwanted variation is minimized and fidelity to the disease can be maximized. This is not a cookbook of how to perform gene targeting or transgenics aimed at transgenic or targeting cores, but is intended to aid the investigator in making decisions about which approaches are best suited for achieving their experimental goals while highlighting some of the pitfalls associated with the different approaches.
2 Transgenics and Gene Targeting
In transgenics, DNA is randomly inserted into the genome by injecting the swelling sperm nucleus shortly after fertilization (1). The injected embryos are transferred into recipient female mice. About 20% of the offspring will have the injected DNA inserted into their genome. Each of the transgene-positive offspring is used to establish a line of offspring carrying that insertion of the transgene by breeding founder animals to wild-type mice of the appropriate strain.
In gene targeting, changes are introduced into an endogenous gene using homologous recombination with manipulated DNA from that gene (2,3). Gene targeting is performed in embryonic stem (ES) cells in culture. Because gene targeting is a rare event, drug selection minigenes are incorporated into the targeting DNA construct, and then bona fide gene targeted cell lines must be identified from among the cells surviving drug selection. The fraction of correctly targeted cells in the cells surviving selection can be as large as one-quarter, but can be much smaller. Cell lines are screened for homologous recombination on one side of the targeted construct, then positive cell lines are propagated and characterized in more detail to ensure that homologous recombination occurred on both sides, and all elements that should have been introduced into the gene were in fact recombined in. Correctly targeted cell lines are analyzed to ensure that they have the correct number of chromosomes, since aneuploid cell lines rarely transmit through the germ line. Euploid, correctly targeted cell lines are combined with recipient embryos, and the chimeric embryos are transferred into a host female. The ES cells and the host embryo are typically marked by different coat color genes, and typically the chimeras are mated to mice of a chosen coat color such that offspring derived from the ES cell component can be distinguished from those derived from the host embryo. Half of the ES-derived offspring should carry the targeted gene.
3 Genetic Mechanism of Disease and Choice of Technologies
Consideration must be given to the genetic mechanism of the disease to be modeled. Typical genetic mechanisms of disease include mutations that eliminate gene function, mutations that alter gene activity, and mutations that increase gene copy number.
If a disease results from complete loss of function (a null mutation), then the gene can be inactivated by gene targeting (gene knockout). Both homozygous recessive traits (both gene copies inactivated by mutation) and haploinsufficient traits (one gene copy inactivated) can be modeled by gene knockout.
If a disease results from increased or novel activity of the gene product, then either transgenes that express mutant product or targeted sequence changes (knockin) to the endogenous gene can be used. If the mutations lead to partial loss of function, then gene targeting can be used to introduce a specific compromising mutation into the endogenous gene (knockin).
If the disease is due to increases in gene copy number, then transgenes can be used to increase gene copy number.
Also, consider that while many disease-causing mutations are not complete loss-of-function mutations (null mutations), null mutations are the most informative mutation for the normal function of the gene, and can be used in conjunction with disease-causing mutations to better understand molecular mechanisms.
4 Minimizing Unwanted Variation
One strength of animal models is that variation can be minimized, such that experiments can have greater sensitivity. Minimized variation results if genetic background, environment, and epigenetic state are uniform. Of course, variation in phenotype can arise directly if different mutations at the disease-causing locus have different effects on gene activity, but this variation is apparent and in the control of the investigator.
Variation can arise through interaction of a disease-causing mutation with segregating variants elsewhere in the genome. The effects of these gene–gene interactions can be quite large (4), and these types of interactions affecting the severity of the disease phenotype have been observed for models of dementia (5–8). Many mouse strains that have no genetic variation at all are available – inbred strains – so variation due to variable gene–gene interactions can be minimized. Genetic models can be generated directly on a small number of inbred strains, or a genetic variant can be generated and crossed onto the inbred strain of choice. The characteristics of inbred strains, which ones are amenable to genetic manipulation, and practical details of breeding inbred model mice are discussed next.
Variable gene expression at the disease-causing locus can arise due to epigenetic variation. DNA methylation and chromatin structure are heritable from cell to cell, and even from generation to generation in some cases. The problem is that the epigenetic state is not completely stable and can switch stochastically, from one permissive for expression, to one that is not. Most genes in the genome probably do not have stochastic variation in epigenetic state, but it is quite common at foreign DNA experimentally inserted into the genome, particularly in intergenic regions. It is assumed that epigenetic silencing of introduced DNA is a protective mechanism against foreign DNA, such as might arise from viral genome insertion. The likelihood of silencing is influenced by the nature of the inserted DNA and the epigenetic state of the DNA surrounding the insertion (9–11). Importantly, variation in epigenetic state is exacerbated with small transgenes inserted in large numbers at one site. Transgenes insert in direct (tandem, head-to-tail) repeats at a single site. For small transgenes, the array can contain hundreds of copies. The repetitive nature of the transgene array promotes epigenetic silencing (12–14). Epigenetic silencing at a transgene array can vary from cell to cell, can increase with age, and can vary from animal to animal (10,12,15). Transgene arrays, once silenced in the germ line, usually remain stably silenced from generation to generation (16–18). Thus, it is important to monitor gene expression from generation to generation in transgenic mice with large transgene arrays.
Modern mouse housing practices seek to eliminate environmental variation through the control of ventilation, temperature, humidity, noise, vibration, photoperiod, enrichment, infectious agents, and diet. Although housing and veterinary care of the mice typically is managed by animal care staff and veterinarians of an animal facility, investigators should take an active interest in the housing, care, and infectious disease state of their animals to ensure that stated standards are met and procedures followed.
Environmental perturbations can come from unexpected sources. Mouse housing units consist in part of plastic, which come into contact with the mice. When cages made from polycarbonate and polysulfone degrade, they release the estrogenic compound bisphenol A (19,20). Polysulfone caging is more stable than polycarbonate, releasing less bisphenol A (19). Visibly degraded or hazed plastics should be removed from use.
5 Artifacts of Genetic Manipulation
Genetic manipulations occasionally have unintended consequences. Transgene insertions can physically disrupt genes at their site of insertion. The frequency of phenotypes arising from insertion site mutation by a transgene (almost 10%) is higher than might be expected from random integration into the genome. The higher than expected mutation rate results because transgenics generated by pronuclear injection can generate large deletions and complex rearrangements at the site of DNA integration (21–31). If a transgene cannot be made homozygous, or the homozygous mice have an unexpected phenotype, then this may indicate that there is an effect from the insertion site. Moreover, if an unexpected phenotype is not seen in other lines of mice from different founder mice with the same transgene, then the phenotype may be due to the insertion site. Thus, it is prudent to generate multiple lines of transgenic mice from independent founder animals and to compare the phenotypes of these lines.
In addition to direct physical interruption of genes, integrations can also have effects on the expression of neighboring genes (32–35). This more indirect effect has been observed in a few well-characterized gene knockouts, but in principle this phenomenon could apply to transgenes as well. Where indirect effects on neighboring genes have been observed, the effect is due to a selectable marker minigene introduced into the endogenous, mutated gene. In most cases where this phenomenon has been observed, the affected neighboring genes are close to the targeted gene, and have been closely related to the targeted gene. In gene knockouts, effects on the expression of neighboring genes can be minimized by designing knockouts with the drug resistance gene cassette flanked by recognition sequences for a site-specific recombinase (such as Frt or LoxP sites for Flpe or Cre recombinase, respectively). The drug resistance minigenes can be removed after targeting by transient expression of recombinase in ES cells or by crossing the knockout to a mouse, which expresses recombinase in the germ line.
Individual transgenic lines made with characterized promoters and small transgenes are occasionally ectopically expressed. This aberrant expression may be due to influences from the site of integration, and is observed more frequently with weak promoters.
6 Inbred Strainsof Mice
A large number of inbred strains of mice exist, but only a small number are commonly used to make transgenics or gene targeted mice. The C57BL/6, 129, and FVB inbred strains are commonly used to generate genetically altered mice. Inbred strains are less robust than hybrid strains in knockout and transgenic production, but if the experimental goals are best served with an inbred strain, it is wise to start on an inbred strain to avoid the almost 2½ years needed to cross the mice to a new background. The choice of strain depends on the characteristics of the strain including susceptibility to the disease and whether other genetic variants of interest are present on that strain.
Inbred strains differ in susceptibility to disease phenotype, as well as in neuroanatomy, sensory acuity, and proficiency in behavioral tests (36). The C57BL/6 inbred strain has relatively normal neuroanatomy and is susceptible to dementia phenotypes (5–8,37), and many genetic variants have been established on C57BL/6J. C57BL/6J has age-related hearing loss (38), and is susceptible to dermatitis (39). 129 mice typically have a small corpus callosum and perform poorly in learning tests (37,40), and may have a reduced severity of dementia phenotype (5). The FVB strain is blind due to retinal degeneration and thus performs poorly in behavioral assays requiring vision (41–43). Fewer genetic variants are available on 129 and FVB than on C57BL/6J. Although there are only a small number of studies thus far, C57BL/6 and FVB mice appear to be more susceptible to both Alzheimer’s and Huntington’s disease pathology than 129 mice.
7 Transgenics
Three types of transgene will be considered: small, cDNA-based transgenes; large, genomic DNA-based transgenes; and dual transgene (digenic) systems.
Small cDNA-based transgenes consist of a promoter, a complete protein-coding sequence from a cDNA, and a polyadenylation signal cloned into a high-copy-number plasmid. An intron is sometimes included in the construct to increase expression (47,48). These elements are cloned into the plasmid such that the transgene can be liberated in one piece from the plasmid backbone by digestion with restriction endonuclease(s), and the backbone-free construct isolated from a gel. The backbone is not included in the injected DNA since the backbone promotes epigenetic silencing (49,50). The injected DNA makes an array of head-to-tail copies, which inserts at a single site in the genome (1). The expression levels of the transgene-encoded products typically do not correlate with the number of copies of these cDNA-based transgenes (12). Different transgenic founders can have different expression levels, and this variation can be used to investigate if the phenotype varies with transgene expression level. Expression from a transgene of this kind can be considerably higher than that from the endogenous gene.
Promoters that drive ubiquitous or tissue-specific transgene expression have been developed. A list of characterized brain-specific promoters, which have been used in dementia models, is listed in the supplementary material to Gotz and Ittner [51).
Briefly, promoters which have been used to create models of dementia include those from the Thy1 (Thy1.2), Prnp (PrP), Pdgfb (Pdgf-beta, PDGF), Camk2a (CAMKII), Eno2 (NSE), and GFAP genes. These genes vary in their spatial pattern of expression and the level of expression. The Thy1.2 promoter drives strong expression in most or all neurons beginning at early postnatal stages (52,53). The Prp promoter drives strong expression in neurons in the hippocampus, Purkinje cells, and a few other neuronal cell types (54). The PDGF promoter drives moderate expression in neurons of the cortex and hippocampus (55). The CAMKII promoter drives postnatal expression in forebrain-derived neurons (56). The NSE promoter drives strong expression in postmitotic neurons, beginning on embryonic day 9.5 (57). The GFAP promoter drives robust expression in astrocytes (58).
The coding sequence typically is derived from a full-length cDNA. Mutations or tags can be incorporated into the coding sequence by many different methods.
Polyadenylation signals are needed for transgene-encoded RNA to accumulate. The AAUAAA in the 3’ untranslated region of typical mRNAs is not sufficient to promote 3’ end cleavage and polyadenylation in this context. Two different polyadenylation sequences are in common use: the bovine growth hormone and SV40 polyadenylation sequences (59,60).
Given the propensity of small transgenes to be epigenetically silenced in mice, and in order to fully characterize different transgenic lines for expression, plan to monitor expression of the transgene products. Ideally, the transgene-encoded protein is monitored, rather than the RNA. This can be accomplished if the protein is from a different species and species-specific antibodies are available, if antibodies detect the transgene-encoded mutant form of the protein, or the protein is tagged with an epitope.
Recently, large genomic fragments containing entire genes have become easier to manipulate to generate transgenic mice (61,62). Large fragment genomic DNA libraries in bacterial artificial chromosome (BAC) vectors have been made from many species, including humans and many strains of mice. The average size of the cloned genomic DNA (typically 150 kb) is such that most genes and the cis-acting sequences required for their expression can be contained within a single clone (63). BAC clones are stable in their bacterial hosts, and can be isolated with commercial large plasmid purification kits (64). Some of these libraries, both human and mouse, have had many clones sequenced at both ends. The paired end sequences were used to tile the clones on the genome assemblies (65–67). The partially sequenced clones are available for purchase in their bacterial hosts. Many BAC genomic clones, when injected as transgenes into mice, recapitulate the normal temporal and spatial pattern of expression of the endogenous gene at comparable expression levels (63). A technology for introducing mutations into the BAC clones, recombineering, is available (64,68–70). If use of the recombineering technology will be limited to a small number of constructs, it may be expedient to use a commercial recombineering service.
Genomic clones in BAC vectors are typically injected as intact circular molecules including the vector. The DNA concatemerizes and a small number of intact copies integrate at a single site (71). Unlike small, cDNA-based transgenes, the expression level of these large transgenes correlates with copy number (71,72). Epigenetic silencing has not been reported to be a problem with these large transgenes, presumably because they resemble mouse genes rather than foreign DNA. Detection of expression from unmodified mouse BAC clones can be difficult because of similarity or identity to the endogenous gene, thus use of human clones, or tagging or modifying a mouse coding sequence by recombineering should be considered.
Large genomic DNA fragments cloned in yeast artificial chromosome (YAC) vectors have also been used to generate transgenic mice. Although YAC vector libraries have larger DNA fragments on average, clones are much more prone to DNA chimerism, are much more difficult to isolate and use to generate transgenics than BAC clones (73).
Temporal control of expression is possible with digenic transgene systems. The most prevalent of these are the variants of the tetracycline-regulated transcription factor (74–76). One transgene of the pair consists of a responder transgene with the target coding sequence under control of a minimal promoter and binding sites for the trans-acting factor. The activity of the trans-acting factor is regulated by binding the tetracycline analogue doxycycline, which is supplied in drinking water. The trans-acting factor is typically expressed from a tissue-specific promoter in a second, independently established transgene. The two transgenes are brought together by breeding. In this way, both timing and tissue of expression can be precisely controlled. A number of well-characterized driver genes are available.
Most but not all components of digenic systems are small transgenes, and thus are susceptible to the epigenetic silencing that affects cDNA-based transgenes. Therefore, monitoring of the expression of both transgenes of the digenic system may need to be factored into the research plan.
For the creation of models of dementia, BAC transgenes have the advantages of expression which better mimics the endogenous gene and relative resistance to epigenetic silencing. For experiments that require high-level expression of a transgene product, the plasmid-based transgene approach works well. If control over the timing of expression of the transgene product is desired, the tetracycline-regulated system would be most suitable. The genetic background of choice is the C57BL/6J inbred strain.
8 Gene Targeting Strategies
In gene targeting, an endogenous gene can be altered in a wide variety of ways: it can be rendered nonfunctional by deleting essential sequences (gene knockout), have sequences replaced or added (gene knockin), or made into a conditional mutant (e.g. a “floxed allele”).
Because the genomic DNA used to construct the targeting vector needs to be from the same strain as the cell line (77), first a decision must be made about which ES cell line to use. Historically, most gene targeting was done in lines derived from the 129 inbred strain. Cell lines derived from 129 were better able to retain their chromosomes in culture, and thus were more likely to transmit the mutations through the germ line of mice (78). Recently, a number of excellent cell lines have become available from C57BL/6N mice (45,78–80). C57BL/6N and C57BL/6J diverged in 1951. There are a handful of known genetic differences between the two strains, including a null mutation in nicotinamide nucleotide transhydrogenase (Nnt) in C57BL/6J not present in C57BL/6N (81). After targeting in C57BL/6N, the chimeras can be bred to C57BL6/J directly. If the known genetic differences are a concern, they can be eliminated in two crosses to C57BL6/J by monitoring for the variants in the offspring.
End-sequenced genomic clones in BAC vectors, which can be used for the construction of targeting vectors, are available for both 129 and C57BL/6J (65,66).
Gene targeting has great fidelity to the genetics of the disease since the endogenous gene is targeted. However, there are disadvantages to gene targeting. Production of a mouse model by gene targeting takes more time than by transgenics. Importantly, success is less assured with gene targeting than with transgenics. Occasionally, a gene targeting vector will not produce gene targeted cell lines at all. It is not clear why targeting fails in these instances, and the usual resolution involves increasing the extent of homologous DNA or choosing a different part of the gene to target. In our experience, about 80% of gene targeting vectors target successfully. In addition, only about 80% of ES cell lines with a normal number of chromosomes will transmit through the germ line. If multiple correctly targeted cell lines were generated, then this second issue can be overcome by making chimeras with two or more cell lines for each targeting experiment.
9 Timeline for Transgenics and Gene Targeting
Moving a mutation or transgene to an inbred strain requires nine consecutive crosses to mice of the target strain, including at least one cross through each sex. This process takes a minimum of slightly more than 2 years. DNA closely linked to the gene variant will remain from the original strain on which the variant was generated.
In transgenics, it is 3 weeks from DNA injection to birth of offspring, 3 weeks to weaning when the mice containing the injected DNA can be identified, and another 3–5 weeks for females and males, respectively, to reach sexual maturity. Transmission of the transgene into offspring requires 6 weeks to weaned offspring. Thus, a minimum of 15–17 weeks is required from DNA injection until a transgene from a founder mouse is established in multiple mice, the start of a line of transgenic mice.
In gene targeting, it takes a minimum of 8 weeks to electroporate the DNA construct and do an initial characterization of cell lines to identify potential targeted clones, then a minimum of another 6 weeks to fully characterize targeted cell lines for the construction of chimeras. From injection of targeted embryonic stem cells into host embryos, it takes 12 weeks for chimeras to reach sexual maturity, and another 6 weeks for weaned offspring, which will be tested for germ line transmission. Thus, a minimum of 32 weeks is required after the targeting vector is constructed to reach heterozygous, gene targeted mice.
These timelines do not include the time required to build DNA constructs or develop assays to identify founder mice or targeted ES cells. The DNA constructs for gene targeting are more complicated to construct, and the verification of gene targeting is more involved than identifying transgenic founder mice.
< div class='tao-gold-member'>
Only gold members can continue reading. Log In or Register a > to continue
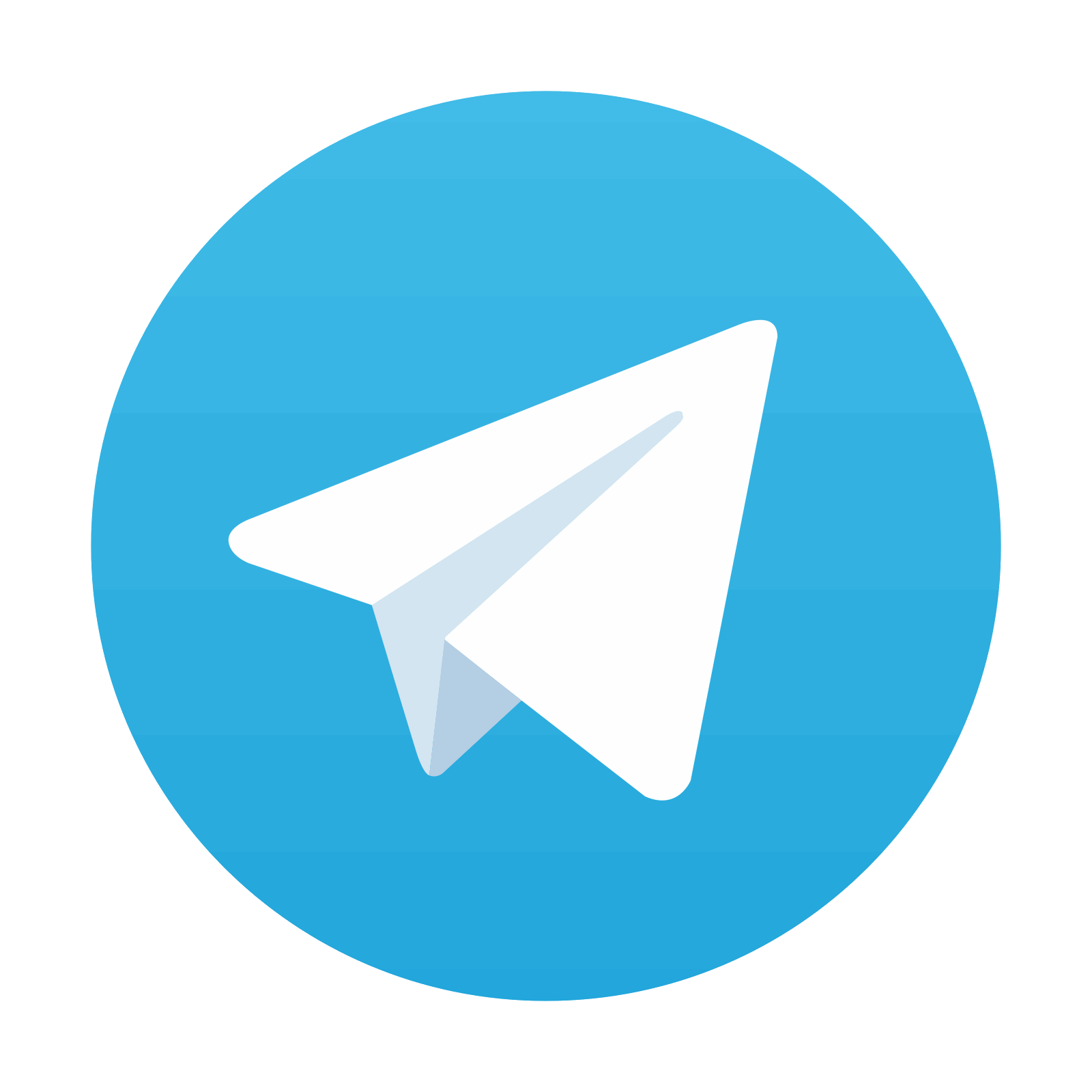
Stay updated, free articles. Join our Telegram channel

Full access? Get Clinical Tree
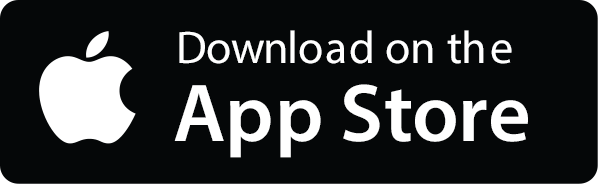
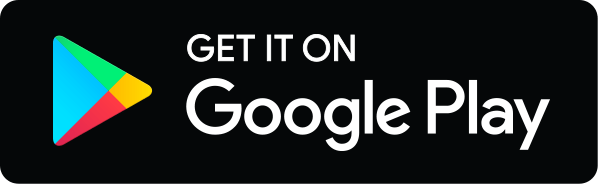