1. Hormones are chemicals produced by specific tissues that are transported by the vascular system to affect other tissues at low concentrations. 2. The endocrine and nervous systems are integrated in their control of physiological processes. 1. Protein hormones are initially synthesized as preprohormones and then cleaved in the rough endoplasmic reticulum to form prohormones and in the Golgi apparatus to form the active hormones, which are stored in granules before being released by exocytosis. 2. Steroids are synthesized from cholesterol, which is synthesized by the liver; steroids are not stored but are released as they are synthesized. Transport of hormones in the blood 1. Protein hormones are hydrophilic and carried in the plasma in dissolved form. 2. Steroids and thyroid hormones are lipophilic and carried in plasma in association with both specific and nonspecific binding proteins; the amount of unbound, active hormone is relatively small. 1. Protein hormones have specific receptors on target tissue plasma membranes, whereas steroids have specific receptors within the cytoplasm or nucleus. 1. Steroids interact directly with the cell nucleus through the formation of a complex with its cytosolic receptor, whereas protein hormones need a messenger because they cannot enter the cell. 1. Steroid hormones are metabolized by conjugation with sulfates and glucuronides, which makes steroids water soluble. 1. The most important feedback control for hormones is the negative-feedback system, in which increased hormone concentrations result in less production of the hormone, usually through an interaction with the hypothalamus or pituitary gland. 2. Endocrine secretory patterns can be influenced by factors such as sleep or light and can produce circadian rhythms. 1. The hypothalamus coordinates the activity of the pituitary gland through the secretion of peptides and amines. 1. The neurohypophysis has cell bodies that originate in the hypothalamus, with cell endings that secrete oxytocin and vasopressin. 2. Oxytocin and vasopressin are synthesized in cell bodies within the hypothalamus and are carried by axon flow to the posterior lobe, where they are released. 3. The main effects of oxytocin are on the contraction of smooth muscle (mammary gland and uterus); the effects of vasopressin are primarily on the conservation of water (antidiuresis) and secondarily on blood pressure. 4. Plasma osmolality controls the secretion of vasopressin. 5. The anterior pituitary produces growth hormone, prolactin, thyroid-stimulating hormone, follicle-stimulating hormone, luteinizing hormone, and corticotropin. 6. Adenohypophyseal activity is controlled by hypothalamic releasing hormones, which are released into the portal system, which in turn connects the median eminence of the hypothalamus and the anterior pituitary gland. • Paracrine effectors, in which the messenger diffuses through the interstitial fluids, usually to influence adjacent cells; if the messenger acts on the cell of its origin, the substance is called an autocrine effector (Figure 33-1). • Neurotransmitters, which affect communication between neurons, or between neurons and target cells; the substances are limited in the distance traveled and the area of the cell influenced (Figure 33-2). • Exocrine effectors, such as hormones produced by the pancreas, are released into the gastrointestinal tract. The major classes of hormones include proteins (e.g., growth hormone, insulin, corticotropin [previously called adrenocorticotropic hormone, or ACTH]); peptides (e.g., oxytocin and vasopressin); amines (e.g., dopamine, melatonin, epinephrine); and steroids (e.g., cortisol, progesterone, vitamin D). The protein and peptide hormones are initially synthesized on ribosomes as larger precursor proteins, which are referred to as preprohormones (Figure 33-3). Synthesis of protein hormones begins in ribosomes, with the “pre” portion immediately attaching to the rough endoplasmic reticulum (RER), which pulls the ribosomes into close apposition with the RER. During synthesis, the preprohormone is secreted into the interior of the RER. The presence of a peptidase within the wall of the RER allows the “pre” portion of the molecule to be rapidly removed and the prohormone to leave the RER in vesicles that have been pinched off from the RER. These vesicles then move to the Golgi apparatus, where they coalesce with Golgi membranes to form secretory granules. The prohormone is cleaved during this process, so most of the hormone is in its final form within the Golgi apparatus, although some prohormone can also be found. Steroids represent a class of hormones that, unlike protein hormones, are lipophilic. In general, they belong to one of two categories: adrenocortical hormones (glucocorticoids, mineralocorticoids) and sex hormones (estrogens, progesterone, androgens). They have a common four-ring, 17-carbon skeleton that is derived from cholesterol (Figure 33-4). Although the steroids can be synthesized de novo within the cell from the two-carbon molecule acetate, the majority of steroids are formed from cholesterol, which is synthesized by the liver (Figure 33-5). Low-density lipoproteins (LDLs) enter steroid-producing cells through interaction with a membrane receptor. Cholesterol is released through the degradation of LDLs by lysosomal enzymes. Cholesterol is either used immediately for steroid synthesis or stored in granules in an ester form within the cell. The first step in the synthesis of all steroid hormones from cholesterol involves cleavage of the side chain of cholesterol to form pregnenolone; this step occurs within the mitochondrion. Subsequent modifications of the steroid molecule may occur within the mitochondrion or may involve movement to other compartments of the cell (Figure 33-6). The control of movement of steroids among cell compartments during the synthesis process is not well understood. The events that follow binding of the hormone and receptor depend on whether a steroid, protein, or peptide hormone is involved. With steroids, the hormone is able to interact within the cell because of its ability to penetrate the lipoprotein plasma membrane (Figure 33-7). The interaction of receptor and steroid hormone results in activation of the subsequent complex translocation to the nucleus, where it interacts with specific sites on the chromatin. The result is the production of mRNA, which, when translocated to the ribosomes, directs synthesis of proteins that produce the desired biological result. Protein or peptide hormones require an intermediary to act in their behalf because they are not able to penetrate the plasma membrane of the cell; the intermediary substance is known as a second messenger (Figure 33-8). The best-documented second messenger is cAMP, which is produced by the activation of an enzyme, adenyl cyclase, through interaction of the hormone and receptor in the plasma membrane. The activation of adenyl cyclase and the production of cAMP result in the phosphorylation of protein kinases, which are responsible for the biological response. Other second messengers include cytosolic calcium and its associated phosphodiesterase, calmodulin, as well as inositol triphosphate (IP3) and diacylglycerol, both of which are products of phosphatidylinositol metabolism. An important action of IP3 is the stimulation of intracellular calcium release. One important response to diacylglycerol is the activation of phospholipase A and the formation of arachidonic acid, which leads to formation of members of the prostaglandin family of molecules. The biological response to a protein or peptide hormone–receptor interaction is more rapid than that to steroids; preexisting enzymes are activated, whereas the biological response to steroid requires the synthesis of enzyme protein.
The Endocrine System
General Concepts
Hormones Are Chemicals Produced by Specific Tissues That Are Transported by the Vascular System to Affect Other Tissues at Low Concentrations
Synthesis of Hormones
Protein Hormones Are Initially Synthesized as Preprohormones and Then Cleaved in the Rough Endoplasmic Reticulum to Form Prohormones and in the Golgi Apparatus to Form the Active Hormones, Which Are Stored in Granules Before Being Released by Exocytosis
Steroids Are Synthesized from Cholesterol, Which Is Synthesized by the Liver; Steroids Are Not Stored but Are Released as They Are Synthesized
Transport of Hormones in the Blood
Protein Hormones Are Hydrophilic and Carried in the Plasma in Dissolved Form
Postreceptor Cell Responses
Steroids Interact Directly with the Cell Nucleus Through the Formation of a Complex with Its Cytosolic Receptor, Whereas Protein Hormones Need a Messenger Because They Cannot Enter the Cell
< div class='tao-gold-member'>
Stay updated, free articles. Join our Telegram channel

Full access? Get Clinical Tree
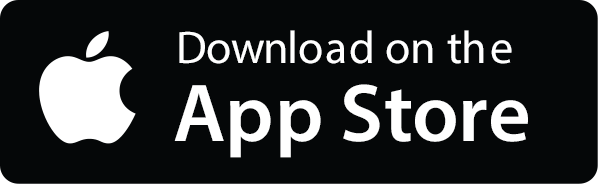
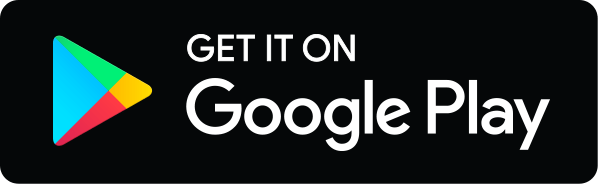