Chapter 11 Surgery of the Bovine Musculoskeletal System
11.1 Musculoskeletal Examination in Cattle
Musculoskeletal examination of cattle lacks uniformity, and many techniques for lameness examination have been described. Veterinarians most often subjectively evaluate the animal to determine which limb or region is affected and then rely on physical examination to determine the diagnosis. This system is useful in identifying lameness sites in most cases because the temperament and lack of training of cattle prevents a thorough lameness exam and necessitates consideration of other information, such as most commonly affected sites, diseases more common to a given age group, and historical data. In horses, flexion and extension tests, selective perineural anesthesia, and intraarticular anesthesia are used to isolate a focal area of pain. These techniques can be adapted to use in cattle, but they have not become commonplace because cattle are infrequently halter trained, often do not tolerate limb handling followed by controlled walking and trotting, and commonly are housed on surfaces that have a higher risk of falling (e.g., wet concrete). Further, cattle are less commonly presented to the veterinarian for examination of subtle diseases that cause mild lameness (e.g., osteochondrosis, bowed tendons, stress fracture). Thorough history, observation of stance and stride, and physical examination is critical to the diagnosis of lameness in cattle. The clinician should remember that 80% to 90% of lameness in cattle originates distal to the fetlock. For this particular reason, unless there is an obvious lesion on the limb, such as a swollen joint or fracture, examination of the claws should always be performed.
Lameness Examination
POSTURE
Many lamenesses are obvious by observing the cow’s stance. Attention should be paid to the posture of the cow—including the back, shoulders, pelvis, and major limb joints. Back posture was a primary factor in a lameness scoring system proposed by Sprecher et al. (Table 11.1-1). With the animal standing, observe the general stance first and more specifically of each limb and digit. Compare one region to the opposite side and determine whether obvious swelling, wounds, shifting of weight, and foot posture such as toe touching or displacement of weight-bearing onto the medial or lateral claw are present. A cow with heel lesions (Figure 11.1-1) will have a tendency to relieve its pain by standing on the toes. A cow with laminitis will tend to place the feet such that weight is shifted to the heels. Cows with sole lesions of the medial digits of the front limbs tend to place their feet such that weight is shifted to the lateral digit. Cows in which bilateral sole lesions are present may cross their forelimbs (Figure 11.1-5).
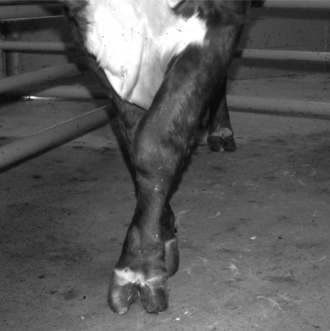
Figure 11.1-5 Bull with bilateral medial sole abscesses; note crossing of limbs to avoid medial weight-bearing.
(Courtesy Thomas J. Divers; Cornell University.)
If only one of the digits is affected and the disease is not severe, the animal will bear weight on the sound digit of the same foot. Examination of the foot reveals excess wear of the wall and sole of the healthy digit. In long-standing diseases with severe lameness, the heels are taller and the wall longer on the affected digit in comparison to that of the healthy claw. A dropped fetlock (e.g., hyperextension of the fetlock joint) may be noticed on the sound limb because of excessive load on the flexor tendons and suspensory ligament (Figure 11.1-2). In young animals, angular limb deformities secondary to uneven weight-bearing occur rapidly with chronic lameness. When chronic lameness occurs in a hind limb, the contralateral tarsus typically will develop varus deformity (the limb is bowed outward so the convex surface occurs in the lateral aspect of the limb; see Figure 15.3-3). When chronic lameness occurs in a forelimb, the carpus typically develops a valgus deformity (the limb is bowed inward so the lateral aspect of the limb has a concave appearance, Figure 11.1-3).
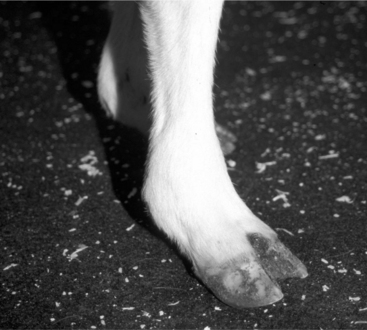
Figure 11.1-2 Dropped fetlock as a result of excessive weight-bearing.
(Courtesy Michael Wildenstein; Cornell University.)
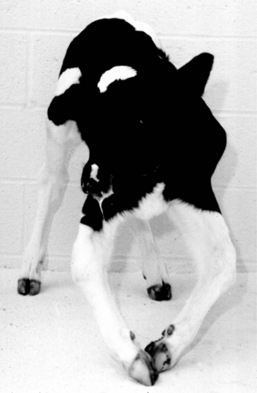
Figure 11.1-3 Calf with bilateral valgus deformity of the carpi.
(Courtesy Norm G. Ducharme; Cornell University.)
Cows with laminitis often stand with the back arched and the feet placed under the body (Figure 11.1-4A and B). They are reluctant to walk. Draining tracts above a swollen coronary band associated with a severe lameness is typical of septic arthritis of the distal or proximal interphalangeal joint (see Figure 11.4-12). Cattle affected with heel erosion or inderdigital dermatitis will tend to keep their heels just on the border of the gutter in a tie stall barn to relieve the pain. They shift weight constantly because of the discomfort. On certain occasions, the animal will relieve the pain on the affected claw by bearing weight on the sound claw only. If both front medial claws are affected, they may cross their legs (Figure 11.1-5).
Differential diagnoses for non–weight-bearing lameness should always include the following: sole abscess, fracture, joint luxation, weight-bearing ligament or tendon injury, nerve injury (e.g., radial nerve, femoral nerve, sciatic nerve), septic arthritis, and septic tenosynovitis. An abnormal deviation of the limb is usually related to a fracture, collateral ligament rupture or joint luxation. The stance and position of the limb is abnormal with nerve damage, tendon rupture, or a severe ligament injury. Cattle affected with a radial nerve paralysis will have a dropped elbow, but this must be differentiated from a humerus fracture, radius/ulna fracture, or septic arthritis of the elbow joint. A rupture of the muscular or tendinous portion of the gastrocnemius muscles is shown by hyperflexion of the hock and a dropped calcaneus (e.g., hock is horizontal rather than vertical during weight-bearing; see Figure 11.4-29), but this must be differentiated from a fractured calcaneus or sciatic nerve paralysis (Figure 11.4-31). Careful attention should be paid to muscle atrophy because this may be caused by nerve injury or disuse atrophy. Neurogenic muscle atrophy occurs rapidly and is severe. Muscle atrophy caused by disuse occurs over a longer period of time. Chronic lameness of the front limb will usually bring atrophy of the triceps, biceps, and scapular muscles. The consequence of this atrophy is a more apparent shoulder with joint instability and the animal may be falsely diagnosed with shoulder joint diseases. Similarly, atrophy of the muscles of the rear limb causes pronounced greater trochanter of the femur that may be misdiagnosed as a coxofemoral joint luxation.
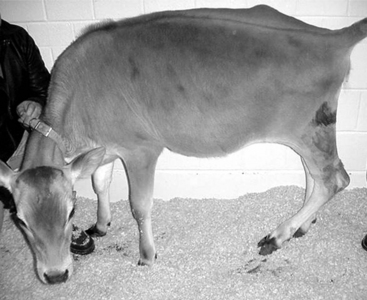
Figure 11.4-29 Jersey calf with acute avulsion of the gastrocnemius muscles after a misdirected intramuscular injection was made near the sciatic nerve. The calf’s weight is partially supported by an assistant to prevent complete plantigrade stance, as seen in Figure 11.4-30.
EXAMINATION IN MOTION
In certain cases, lameness is subtle, and other procedures are necessary to localize the lesion. The characteristics of the lameness can more easily be assessed with the animal walking. The observer should attempt to describe the severity of the lameness and assess the individual components of the gait including the arc of flight, position of the digit when it touches or leaves the ground, and the relative time spent in each phase of the stride. An example is a sole ulcer of the left front medial digit (see Figure 11.1-1). This disease may cause a shortened weight-bearing phase of stride and a prolonged non–weight-bearing phase of stride in the affected limb because the cow is reluctant to place the foot down and quick to relieve pressure by picking the foot up off the ground. This animal may place the foot with the limb carried further under the body in an attempt to transfer weight to the lateral digit and may place the foot closer to the body, rather than extending the limb in an attempt to spare the pressure on the heel.
When diseases of the proximal limb, such as the hip, stifle, or shoulder, are suspected, the individual structures should be palpated as the animal walks. Bone-on-bone crepitation may be felt if a luxation or fracture is present. Soft tissue crepitation may be felt if tendon or ligament injury is present. Gas crepitation may be felt if emphysema of the tissues is present (e.g., sucking wound, clostridial myositis). It is sometimes difficult to pinpoint the location of the crepitation because it can be felt some distance from the lesion. Marked bone-on-bone crepitation that originates from the stifle often feels similar to the coxofemoral joint; they can be difficult to distinguish. Identification of swelling over the greater trochanter and rectal palpation of the hemipelvis and region of the coxofemoral joint during ambulation may help localize the lesion to the coxofemoral joint. Alternatively, auscultation of the suspected regions with a stethoscope during walking or manipulation of the limb may help localize the point of maximum intensity of the crepitus.
GRADING OF LAMENESS
Assessment of the severity of the lameness is helpful to classify lameness and monitor responses to treatment. For this reason, lameness-scoring systems have been created. Equine lameness-scoring systems have been well standardized. However, these systems are difficult to extrapolate for use in cattle because of the need to control the gait (e.g., walk, trot) and perform various tests (e.g., circling, flexion tests). These tests may be performed in show cattle, but no standardization has been established for cattle. Various scoring systems have been described for use in lameness examination of cattle, and these scoring systems are based more on locomotion rather than responses to specific tests. Greenough and Wells, Sprecher et al, and Anderson (see Table 11.1-1) have described lameness-scoring systems that use grades of 1-to-5 or 0-to-4, but none has been universally accepted in practice. Multiple variations of lameness scoring have been published. Whatever the system used, veterinarians should be consistent and coherent to evaluate with precision. All associates in the same practice should agree on a grading system to facilitate communication within the practice and with the clients.
LIMB EXAMINATION
At this point, the clinician should have an idea of which leg is affected and an estimation of the affected region of the limb. Now, the affected limb must be examined carefully. Unless an obvious lesion is apparent, the authors start by a palpation of the limb. The clinician should watch for pain reaction and determine whether swelling, deformation, crepitation, warmth, and wounds are present. A hoof tester may be used to evaluate pain of the claw. The hoof tester should be applied where the common lesions of the sole surface are usually situated, including the apical region of the sole, the white line zone, and the prebulbar region. The hoof tester may be used to impact the dorsal and abaxial hoof wall to evaluate for a pain response that suggests laminitis, submural infection, and fracture of the distal phalanx. These manipulations can be performed with the animal standing and by picking up the affected limb for a short time. Alternatively, these procedures may be done with the animal free-standing and rope restraints used on the limb, restrained in a head gate or chute (see Figure 4.4-29), restrained in a standing hoof-trimming chute, restrained in lateral recumbency on the ground after a casting rope is used, or restrained in lateral recumbency on a tilt table. Sedatives and tranquilizers should be avoided whenever observations of pain responses are desired. After localization of the lesion, local anesthesia or sedation may be required to complete the examination.
Examination of the coxofemoral joint requires manipulation of the rear limb. These tests can be performed with the animal standing, but they are easier to perform with the animal in lateral recumbency with the affected limb uppermost. The relative position of the greater trochanter to that of the tuber coxae and the tuber ischii should be determined before than animal is laid down. The normal position of the greater trochanter is ventral to both of these bony prominences and imaginary lines drawn between them will create a “triangle” (Figure 11.1-6). Failure to palpate the greater trochanter may suggest a ventral luxation of the coxofemoral joint. Positioning of the greater trochanter in-line with the tuber coxae and tuber ischii suggests dorsal luxation of the coxofemoral joint. After the animal is laid down with the affected leg uppermost, the foot or the metatarsus III/IV is grasped and the entire limb rotated while performing repeated abduction and adduction motions. Fracture of the physis of the head of the femur (i.e. capital physeal fracture) should elicit crepitation of the hip that can be felt and occasionally heard. Coxofemoral joint luxation should elicit more crepitation, excessive movement of the greater trochanter, and ease of abduction if the luxation is ventral.
Cranial cruciate ligament (CCL) rupture is more difficult to diagnose. Typically, the stifle is swollen and painful to palpation. A “drawer” test can be performed with the animal standing and is easier to perform when the injured limb is weight-bearing. The examiner should stand immediately behind the affected leg and place both hands on the tibial crest by encircling the limb. Then, the examiner’s knee is placed on the back of the calcaneus (see Figure 11.4-20). A drawer test is positive if displacement or crepitation can be felt after firm caudal traction on the tibial crest followed by a sudden release. The anatomy and function of the rear limb of cattle is such that the tibia is already displaced cranial to the femur when the CCL is ruptured. Thus caudal movement of the tibia is a sign of “positive drawer.” The examiner must be careful when performing this test to avoid being kicked. An alternative technique is for the examiner to stand cranial and lateral to the affected limb and place both hands on the tibial crest. Then, a firm, rapid thrust is applied to the proximal tibia, and the limb is observed for displacement and felt for crepitus. Although this test is safer to perform, we have found this technique to be less sensitive for detecting injury to the CCL.
USE OF SELECTIVE ANESTHESIA
Selective perineural anesthesia with lidocaine HCl 2% solution is common practice in equines for lameness diagnosis but is uncommon in cattle. Nonetheless, selective perineural anesthesia can be used to isolate regions of lameness. The clinician must be familiar with the anatomy of the nerves of cattle because these are quite different from those of the horse. The authors often employ regional anesthesia by placing intravenous lidocaine distal to a tourniquet (intravenous regional anesthesia, IVRA). The tourniquet is left in place for 10 to 20 minutes, released, and the lameness reevaluated. IVRA induces anesthesia for a significantly shorter period of time in comparison to that of selective perineural anesthesia. Therefore lameness evaluation must be expedited. This technique is useful when the clinician wants to rule out the digits as a source of pain that has failed to be localized by previously mentioned diagnostic tests. With selective perineural anesthesia, each digit can be anesthetized individually, and this procedure aids in more specific isolation of lameness. Occasionally, intraarticular anesthesia is desired to isolate subtle lameness to a specific joint. We have found this to be most useful for diseases that affect the coxofemoral joint, scapulohumeral joint, femoropatellar and femorotibial joints, and the elbow. In complex joints, the examiner should pay special attention to the frequency of joint communication, depending upon which joint is injected (Table 11.1-2).
TABLE 11.1-2 Joint Communications in Cattle
JOINT | COMMUNICATION | FREQUENCY (%) |
---|---|---|
Fetlock | Medial to lateral digit | 99% |
Stifle | Femoropatellar to medial femorotibial | 100% |
Lateral femorotibial to medial femorotibial and femoropatellar | 65% | |
All three together | 57% | |
Carpus | Antebrachiocarpal—no communications | 96% |
Middle carpal to carpometacarpal joint | 86% | |
Middle carpal joint to antebrachiocarpal and carpometacarpal joints | 14% | |
Carpometacarpal joint to middle carpal joint | 86% | |
Carpometacarpal joint to middle carpal and antebrachiocarpal joints | 22% | |
Tarsus | Tibiotarsal to proximal intertarsal | 100% |
Proximal intertarsal to distal intertarsal | 0% | |
Distal intertarsal to tarsometatarsal | 21% | |
Tarsometatarsal joint to distal intertarsal joint | 43% |
Frequency of communication is based on communication when the first joint listed is injected. The phrase all three together indicates that communication did not depend upon which joint was injected.
Adapted from Desrochers A: Characterization of the anatomic communications of the carpus, fetlock, stifle, and tarsus in cattle using intraarticular latex and positive contrast arthrography. Master’s Thesis. Kansas State University, Manhattan, Kan, 1995.
EXAMINATION OF THE FOOT
Adequate restraint is critical to performing a thorough examination of the claw. Fortunately, claw-trimming chutes have become commonplace and facilitate the ease, safety, and efficiency of examination (see Figure 4.4-29). If a chute is not available, different techniques have been described for restraining the animal. Certain animals require sedation either for restraint or to complete the examination. Xylazine HCl is commonly used because of its rapid onset of action, short duration, and the availability of reversal drugs. It is preferable to withhold feed from these animals for 12 to 24 hours before sedation to avoid bloating and reduce the risk of regurgitation while in lateral or dorsal recumbency. However, we routinely place cattle in lateral recumbency for short periods of time (e.g., <30 to 45 minutes) without restricting feed. Close monitoring of the patient is required to prevent respiratory compromise or regurgitation. Immediately after application of restraint, the foot is thoroughly cleansed. Thorough examination is difficult to perform when mud, manure, and debris contaminate the foot. The total time the animal is maintained in lateral recumbency should be minimized to reduce the likelihood of development of muscle or nerve injury. Heavily muscled and obese cattle are at increased risk of developing myopathy or neuropathy after prolonged periods of lateral recumbency. Excessively thin animals are at increased risk of developing nerve injury. These conditions are caused by direct compression injury and by indirect injury resulting from compression of vessels that causes hypoxia to the tissues.
Amstutz HE. Assessment of the musculoskeletal system. Vet Clin N Am Food Anim Pract. 1992;8:383-396.
Clarkson MJ, Downham DY, Faul WB, Hughes JW, Manson FJ, Merrit JB, Murray RD, Russell WB, Sutherst JE, Ward WR. Incidence and prevalence of lameness in dairy cattle. Vet Rec. 1996;138:563-567.
Desrochers A: Characterization of the anatomic communications of the carpus, fetlock, stifle, and tarsus in cattle using intraarticular latex and positive contrast arthrography. Master’s Thesis. Kansas State University, Manhattan, Kan, 1995.
Farrow CS. Digital infections in cattle: their radiographic spectrum. Vet Clin N Am Food Anim Pract. 1999;15:411-423.
Farrow CS. The radiographic investigation of bovine lameness associated with infection. Vet Clin N Am Food Anim Pract. 1999;15:425-441.
Goggin JM, Hoskinson JJ, Carpenter JW, et al. Scintigraphic assessment of distal extremity perfusion in 17 patients. Vet Radiol & Ultrasound. 1997;38:211-220.
Greenough PRLameness in cattle. Basic concepts of bovine lameness, ed 3. WB Saunders; Philadelphia; 1997
Kofler J. Application of ultrasonic examination in the diagnosis of bovine locomotory system disorders. Schweizer Archiv fur Tierheilkunde. 1995;137:369-380.
Ley SJ, Waterman AE, Livingston A. Measurement of mechanical thresholds, plasma cortisol, and catecholamines in control and lame cattle: a preliminary study. Res Vet Sci. 1996;61:172-173.
Manson FJ, Leaver JD. The influence of concentrate amount on locomotion and clinical lameness in dairy cattle. Animal Production. 1998;47:185-190.
Philipot JM, Pluvinage P, Luquet F. Clinical characterization of a syndrome by ecopathology methods: an example of dairy cow lameness. Vet Res. 1994;25:239-243.
Raven ET. Parage. In Soins des onglons des bovins, parage fonctionnel. Ontario, Canada: Collège de Technologie Agricole et Alimentaire d’Alfred; 1992.
Scott GB. Changes in limb loading with lameness for a number of Friesian cattle. Vr Vet J. 1989;145:28-38.
Singh SS, Ward WR, Lautenbach K, et al. Behavior of lame and normal dairy cows in cubicles and in a straw yard. Vet Rec. 1993;133:204-208.
Sprecher DJ, Hostetler DE, Kaneene JB. A lameness scoring system that uses posture and gait to predict dairy cattle reproductive performance. Theriogenology. 1997;47:1178-1187.
Tryon KA, Clark CR. Ultrasonographic examination of the distal limb of cattle. Vet Clin N Am Food Anim Pract. 1999;15:275-300.
Weaver AD. Lameness in cattle: investigational and diagnostic checklists. Vr Vet J. 1985;141:27-33.
Wells SJ, Trent AM, Marsh WE, Robinson RA. Prevalence and severity of lameness in lactating dairy cows in a sample of Minnesota and Wisconsin herds. J Am Vet Med Assoc. 1993;202:78-82.
Whay HR, Waterman AE, Webster AJF. Associations between locomotion, claw lesions and nociceptive threshold in dairy heifers during the peri-partum period. Vet J. 1997;154:155-161.
Selection of Cases for Internal Fixation
SPECIAL CONSIDERATIONS FOR CALVES
Ruminant neonatal bones have a low bone density and thin bony cortices; and their ability to support and sustain implants such as intramedullary pins and screws is a primary concern (Fessler and Adams, 1996). The bone/implant interface is very weak (Figure 11.2-1A-C). Recent studies have evaluated various screws’ holding power in neonatal bones (Kirpenstein et al, 1992; Kirpenstein et al, 1993). No difference between the holding power of 4.5-mm cortical, 5.5-mm cortical, and 6.5-mm fully threaded cancellous screws in neonatal femurs was found, but a direct correlation between the screws’ holding power and bone cortical thickness was found. The 6.5-mm fully threaded cancellous screws had greater holding power than either 5.5-mm or 4.5-mm cortical screws in neonatal metacarpal and metatarsal bones, particularly the metaphysis. No difference between the holding power of 4.5-mm and 5.5-mm cortical screws in the diaphysis or metaphysis was found. In a recent study, the force required for screw pullout seemed to be more directly related to bone mineral density than cortical bone thickness (Shettko et al, 2001). Washers and bolts have been used with screws for calf internal fixation to overcome pullout problems and prevent bony failure at the screw-to-bone-to-implant interfaces in neonatal bone (Ferguson, 1985). Regardless of whether bone mineral density, cortical bone thickness, or both are responsible for a screw’s holding power, it remains a concern in calf internal fixation.
Many fractures that occur in young farm animals involve the physis (growth plate). It has been proposed that younger animals are predisposed to physeal-type fractures because the physis cartilaginous makeup is weaker than bone and the surrounding support structures. The Salter-Harris classification (Figure 11.2-2) is used to describe fractures that involve the physis and adjacent metaphysis. In clinical practice, Salter-Harris type I and II fracture in farm animals makes up a large majority (>95%) of physeal fractures. Type I fractures, which are not as common, involve complete separation of the epiphysis from the metaphysis through the physis. A typical type I physeal fracture is the slipped capital femoral physis, which occurs through the femur prox-imal growth plate (Figure 11.2-3A). Other, even less common Salter-Harris type I fractures occur in the tuber calcaneus and olecranon. These latter two fractures occur through traction growth plates (i.e., where muscles or tendons insert or originate). In Salter-Harris Type II fractures (the most common farm animal physeal fracture), the epiphysis separates from the metaphysis through the physis with a small corner of the metaphysis fractured as well. These most commonly occur in the distal metatarsus and metacarpus, distal femur and radius, or proximal tibia (Figure 11.2-4A).
Evaluation of a Fracture for Internal Fixation Repair
RADIOGRAPHY
Intraoperative radiography should also be considered when one is using any form of fixation—but particularly internal fixation. Intraoperative radiography can assess the positioning and placement of implants as well as the reduction of the fracture. Intraoperative radiographs should be completed in more than one view whenever possible to ensure that the repair is appropriate.
EVALUATION OF THE PATIENT
The condition of the surrounding soft tissues should be considered in evaluation of a fracture. The surrounding soft tissues are responsible for extramural blood supply to the fracture site. Open or closed fractures with severely traumatized soft tissues often become infected, which significantly complicates the repair. Infection as a complication is discussed later in this chapter. However, it is paramount to remember that infection and instability are intolerable together. If contamination of the fracture site occurs and persistent infection develops, instability and failure of the repair are highly probable. This is the primary reason open fractures have a much less favorable prognosis than closed fractures. Broad spectrum, preoperative antibiotics are always recommended before fracture repair with internal fixation. Antimicrobials’ duration and withdrawal time needed to achieve proper care must be carefully considered during the selection process because of human public health concerns. The duration and frequency of antimicrobial coverage is controversial among surgeons but should be directly correlated to the type of fracture (open vs. closed) and extent of soft tissue damage.
FRACTURE REDUCTION
The goal of a successful internal fixation is accurate, rigid anatomic reduction. Anatomic reduction allows the bone to share the load transfer and restore function to the leg. Nonanatomical and/or inaccurate reduction predisposes the implant to migration and/or failure. Reduction should be performed by gradually fatiguing the inverse myotactic reflex. Reduction may be achieved by tenting the bone ends out of the incision and toggling them against one another until they align anatomically. However, mechanical devices such as a large animal fracture distractor,* a calf jack, or other pulley system may be needed to provide consistent tension and resultant muscle fatigue in cases in which reduction cannot be performed manually (Ames, 1995). Reduction can be maintained by using bone reduction forceps, placing a lag screw across the fracture gap, or using cerclage wire. The efficacy of neuromuscular blocking agents used is not always predictable, and they do require positive pressure ventilation to overcome respiratory depression.
General Principles of Internal Fixation
BONE PLATES
In large animal orthopedics, a bone plate is a device that shares the load by passing some of the load from the plate to bone fragments. Subjected to bending loads, a plated bone can take on a bending open (compressive surface) or bending closed (tensile surface) configuration (Figure 11.2-5). The plate’s placement relative to the loading direction determines the load proportion supported by the plate. The plate/bone composite is far stiffer in the bending closed position than in the bending open position. This makes it important to anatomically reconstruct the bone cortex opposite the bone plate placement.
Plating provides the most rigid form of internal fixation used in ruminant orthopedics. Application of bone plates should be performed on the tension surface whenever possible because of the biomechanical advantages previously noted. In younger, lighter-weight animals, one plate may be used to achieve stabilization. Oftentimes, two plates are used in larger animals in an effort to obtain adequate stabilization of the fracture. The surgeon should contour the plate(s) to ensure that the entire surface of the plate is in contact with the bone (bone-plate interface). Every effort should be made to optimize the bone-plate interface because this provides a more rigid and stable fracture repair and enhances the likelihood of a positive outcome. Plate(s) should span from the proximal to distal metaphysis to enhance the bone-plate interface and minimize stress concentrated at the bone ends. When two plates are used, they should be placed at 90 degrees to one another and end in a staggered fashion to prevent stress concentration at the bone end and facilitate easier screw placement.
The standard plates used for repair fractures of the long bones are the 4.5-mm narrow and 4.5-mm broad dynamic compression plates; however, other specialty plates are available (Table 11.2-1). The oval shape of the holes in dynamic compression plates allows them to be used to compress fracture fragments. Narrow plates have holes aligned in a straight line, whereas the holes in broad plates are staggered. Narrow plates have a smaller width (12 mm vs. 16 mm) and thickness (3.6 mm vs. 4.5 mm) in comparison to broad plates. Recent reports cite successful use of dynamic condylar screw (DCS) and dynamic hip screw (DHS) plates in long bone fracture repair (Auer et al, 1993) (Figure 11.2-6). The DCS and DHS plates are identical in width to the 4.5-mm broad plates but thicker at 5.6 mm and 5.8 mm, respectively. Other plates—such as the angled, Cobra head, T-, and semitubular plates—have been used in special circumstances.
SCREWS
Two basic types of screws (cancellous and cortical) are used in ruminant orthopedic surgery (Table 11.2-2). The parts of a screw include head, shaft, core, thread, pitch, shaft length, thread length, and total screw length, which vary among the different screw sizes. Cortical screws are completely threaded and have a relatively thin thread width. Cancellous screws are available in various thread lengths and have a wider thread diameter and pitch than cortical screws.
INTRAMEDULLARY PINS AND INTERLOCKING NAILS
Intramedullary devices have several advantages in fracture treatment, including restoration of bony alignment and early recovery of weight-bearing in young, lightweight animals. These devices are intended to stabilize a fracture by acting as an internal splint, thus forming a composite structure of bone and rod in which both contribute to fracture stability. This load-sharing property is fundamental to the rods’ design and should be recognized when they are used for fracture treatment (Figure 11.2-7).
With torsional loading, the unsupported length is determined by the points at which sufficient mechanical interlocking occurs between bone and implant to support torsional loads. The concept of unsupported length is not applicable to simple rod designs without proximal or distal locking mechanisms, since there may be little resistance to torsion. Clinically, placing multiple pins (stack pinning) is recommended to increase intramedullary contact and enhance torsional and bending stability when one uses simple rod designs.
Interlocking nails have been used in human surgery to repair fractures of the humerus, tibia, and femur (Figure 11.2-8). Similar to intramedullary pins, interlocking nails provide stiffness in bending. The use of both proximal and distal locking with screws can prevent axial displacement of the bone along the rod and provide enhanced torsional rigidity. For interlocking nails, the distance between the proximal and distal locking points typically determines the unsupported length. Interlocking nail systems for large animal surgery are commercially available.* Interlocking nails have a single trocar point, are 13 mm in diameter, and vary in length. The interlocking nails have 5.7-mm diameter screw holes placed 16.5 mm apart over their entire length. An open surgical technique is recommended with interlocking nails because of their large diameter. A 6.35-mm diameter intramedullary pin placed in normograde fashion from the proximal location into the medullary cavity and seated distally in the subchondral bone of the distal epiphysis creates a pathway for interlocking nail insertion. The intramedullary pin is then removed. The interlocking nail replaces the intramedullary pin in the same pathway, which decreases the likelihood of the bone fracturing during interlocking nail placement. A slightly smaller-diameter (11 or 12 mm) reaming device is an alternative. The interlocking nail is inserted as described above with a large hand chuck. The aiming device is then attached to the proximally exposed portion of the interlocking nail. The aiming device ensures that drilling and tapping the bone is achieved within the screw holes of the interlocking nail. Longer-shanked drills and taps may need to be used to accomplish this task. Generally two screws each are placed in the distal and proximal primary fracture fragments. Interlocking nails have been used to repair humeral and femoral fractures in calves. The quality of the surrounding bone in neonates may determine the strength of repairs that use interlocking nails. Distal placement of intramedullary devices should be checked by intraoperative radiography or stifle arthrot-omy. Intramedullary pins should be cut proximally at the level of the skin to allow for pin removal and prevent impingement of the sciatic nerve. Intramedullary pin removal can be difficult to perform in rapidly growing animals that have achieved clinical union but poses no problem to the animal if they remain in situ. Removal should be considered if the intramedullary pins have migrated or are associated with an infective process. Interlocking nail length should be selected so soft tissues and skin can cover the proximal end. Interlocking nails cannot be removed without a second surgical procedure.
Fractures of the Femur
CLINICAL PRESENTATION
The definitive diagnosis of a femoral fracture is made by radiography (see Figures 11.2-7 and 11.2-8). At least two standard radiographic views should be performed to assess the fracture. This is most easily accomplished if the animal is tranquilized and placed in lateral recumbency to obtain both mediolateral and craniocaudal views. The most commonly reported femur fractures are an oblique-to-spiral fracture of the mid-to-distal diaphysis or a short transverse-to-oblique fracture of the distal metaphysis, although proximal diaphyseal fractures have been reported (Ferguson, 1994). Comminution is usually present, and high-quality radiographs should be obtained to accurately evaluate the degree of comminution. Spiral and oblique fractures are generally thought to be caused by torsional and bending forces acting upon the bone. Reasons for the high prevalence of distal metaphyseal/physeal region fractures are not completely understood but may be related to the high torsional forces placed on the distal femur.
METHODS OF INTERNAL FIXATION REPAIR
Retrograde, intramedullary stack pinning has been used to repair neonatal bovine femoral fractures with success (St-Jean et al, 1992a). In a recent report, 83% of the fractures treated in this manner were considered to have healed satisfactorily. However, complications such as pin migration occurred in 50% of the cases, and osteomyelitis developed in one case. The authors suggested the use of partially threaded pins to aid in preventing pin migration. All calves in this study had considerable callus formation present, thus suggesting instability. Although intramedullary pins are noted for their strength and stiffness in bending, fracture fragments tend to rotate or displace axially along single intramedullary pins. Stack pinning, external fixators, and cerclage wires have been used to help stabilize the torsional and compressive instability of single intramedullary pin fixation repair; but the results have been variable (Figure 11.2-9).
Fractures of the Femoral Head
CLINICAL PRESENTATION
Fractures of the femoral head or slipped capital physeal (Salter-Harris type I) fractures occur in two primary populations. One is young calves after forced fetal extraction associated with hip lock and shear forces placed across the coxofemoral region. The second population is young (1 to 2 years old) bulls in group housing. Blunt trauma in a lateromedial direction to the coxofemoral region results in shear force across the physis and ultimately failure. Cattle with slipped capital femoral physis have lameness but generally bear weight on the affected leg. In chronic cases, marked muscle atrophy may appear, but visually it is difficult to distinguish between it and other abnormalities. Palpation of the right coxofemoral joint while the left is being placed through a full range of motion typically elicits crepitus. If an assistant is not available, one can often detect a noticeable clicking and crepitus by placing one hand over the coxofemoral joint while pulling the animal’s tail towards the affected side with the other hand. Ventrodorsal or lateral positioned radiographs with the affected leg up, flexed, and abducted (frog-legged) provide a definitive radiographic diagnosis (see Figure 11.2-3, A).
SURGICAL APPROACH
The animal is placed in lateral recumbency with the affected leg uppermost. A dorsolateral approach just cranial to the greater trochanter is used to expose the coxofemoral joint. The incision is extended distally to the distal aspect of the femur. A plane of dissection is created through the fascia lata and cranial border of the biceps femoris. A partial tenotomy of the origin of the vastus lateralis and sometimes middle gluteal and gluteus accessorius is required to provide exposure of the joint capsule (Figure 11.2-10).
METHODS OF INTERNAL REPAIR
The leg is internally rotated to facilitate reduction of the fracture. The fracture can then either be stabilized with 5- to 6.32-mm Steinman pins or 7- or 7.3-mm cannulated screws (Wilson et al, 1994; Hull, 1996) (see Figure 11.2-3, B). The implants are inserted retrograde from the insertion of the accessory gluteal through the femoral neck and into the fragment. Care should be taken not to invade or leave implants in the joint. Radiographic guidance (including fluoroscopy) at surgery is extremely helpful when one is placing implants. Devascularization of the femoral head may occur as a result of fracture or surgical dissection. This is because the blood supply to the femoral head flows through the round ligament of the head of the femur, joint capsule, and femoral neck. The latter two are disrupted by a fracture. During a surgical procedure, every effort should be made to preserve the blood supply to prevent femoral head necrosis from occurring. This is followed by revascularization, bone resorption, flattening of the femoral head, and osteoarthritis (Figure 11.2-11).
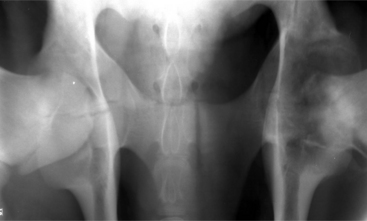
Figure 11.2-11 Femoral head necrosis as viewed on a ventrodorsal radiograph.
(Courtesy of Dr. Ryland B. Edwards III; University of Wisconsin.)
In case of irreversible damage to the articular cartilage of the acetabulum and/or femoral head, femoral head excision should be considered (Squire et al, 1991). In this case an osteotomy of the greater trochanter is performed. Before the osteotomy, the greater trochanter is drilled and tapped, and two or three 6.5 mm cancellous screws are inserted into the intended proximal fragment before the osteotomy. A Gigli wire is used to perform the osteotomy. The femoral neck is transected with an oscillating saw or Gigli wire. The greater trochanter is put back in place with a 1.2-mm wire in a tension band fashion (see Figure 11.4-35). Closure is done by reapposition of the various anatomical planes.
Fractures of the Humerus
SURGICAL APPROACHES
The two described surgical approaches to the humerus include a lateral and cranial approach. The radial nerve is the most important vital structure that must be identified and preserved when either surgical approach is performed. The lateral approach provides exposure to the irregular lateral surface of the humerus and is not a desirable surface for plating the humerus. However, this approach is useful for assisting in placement of intramedullary pins or interlocking nails. The cranial approach provides access to the straighter and more regular surface of the humerus.
For the cranial approach, the animal is placed in lateral position with the affected leg uppermost (Figure 11.2-12). The skin and subcutaneous tissue is incised from the cranial aspect of the greater tubercle of the humerus distally over the cranial aspect of the extensor carpi radialis muscle. The plane of dissection is established by proximally splitting the brachiocephalicus muscle cranial to the deltoid tuberosity. At the distal half of the incision, the caudal border of the brachiocephalicus is separated from its attachment to the brachial fascia. The cephalic vein is identified, double-ligated, and transected. The insertions of the brachiocephalicus muscle on the crest of the humerus distal to the deltoid tuberosity are transected. The underlying deltoid muscle is elevated from the deltoid tuberosity, thus exposing the brachialis muscle along the lateral aspect of the humerus in the musculospiral grove. The belly of the biceps muscle is elevated from the humerus. To gain further access to the distal part of the humerus, a portion of the origin of the extensor carpi radialis muscle is elevated from its attachment to the lateral humeral condyle. The radial nerve is identified along the caudal border of the brachialis muscle and distally between the brachialis and extensor carpi radialis muscle. Retraction of the brachialis muscle caudally and the biceps brachii muscle medially provides sufficient exposure to the cranial cortex. Retraction of the brachialis muscle cranially allows exposure to the lateral aspect of the humerus.
METHODS OF INTERNAL FIXATION REPAIR
Intramedullary pins or interlocking nails may be used to treat diaphyseal fractures of the humerus. Intramedullary pins can be placed in either normograde fashion starting proximally from the greater tubercle passing distally to the medial side of the humeral condyle. Care should be taken to avoid driving pins into the olecranon fossa of the humerus. A single intramedullary pin can be used, or multiple stack pins can be used to increase rotational stability and decrease the likelihood of longitudinal collapse of the fragments. Cerclage wire or interfragmentary compression with lag screws is also com-monly performed when utilizing intramedullary pins (see Figure 11.2-8B). Interlocking nails can also be used to repair diaphyseal and distal fractures of the humerus. Interlocking nails insertion and placement landmarks are similar to those of intramedullary pins.
Fractures of the Radius/Ulna
CLINICAL PRESENTATION
Fractures of the radius are not very common in cattle. Fractures of a cow’s radius usually occur because a leg in a fixed position is struck, typically either in a lateral-to-medial or cranial-to-caudal direction. Fractures of the radius commonly occur in the mid diaphysis or distally through the growth plate as a Salter-Harris type II fracture (see Figure 11.2-1). In adults, fractures of the radius are commonly highly comminuted. Because of the large amount of soft tissue covering the radius, most radial fractures are typically closed. Cattle with fractures of the radius have an appreciable lameness with associated soft tissue swelling of the antebrachium. They tend to rest with the leg pointed and are minimally weight-bearing. On occasion, marked angulation is visible at the fracture site; it is best viewed with a cranial-to-caudal leg position. Radiographs provide the definitive diagnosis. Olecranon fractures are extremely rare (Hague et al, 1997) and present as severe forelimb lameness and an inability to bear weight. The elbow is dropped because of the lack of functional triceps activity. Palpation of the olecranon reveals pain and swelling. The diagnosis is confirmed by radiography (Figure 11.2-13).
SURGICAL APPROACH TO RADIUS
The animal is positioned in lateral recumbency with the affected leg uppermost. A curvilinear incision through the skin and subcutaneous tissue is started at the lateral epicondyle of the humerus and extended distally to the lateral tuberosity of the radius where the collateral ligament of the carpal joints originates (Figure 11.2-14). The apex of the curvilinear incision is directed cranially to help gain exposure to the dorsal aspect of the radius. The skin is reflected, and the plane of dissection is established between the common digital extensor muscle and extensor carpi radialis muscle. Care must be exercised not to damage the radial nerve as it courses the most proximal aspect of the radius. The cranial and lateral surfaces of the radius are readily exposed by this surgical approach. The abductor digiti I longus closely adheres to the lateral aspect of the distal radius and may need to be bluntly reflected.
METHODS OF INTERNAL REPAIR
The radius does not lend itself to intramedullary fixation. Bone plating is the only form of internal fixation that should be considered when one is fixing a fracture of the radius. The tension surface of the radius is located along the cranial cortex. In calves less than 200 kg, a single 4.5-mm broad DCP can easily be contoured to the cranial aspect of the radius. In heavier and older calves, double plating should be considered. Plates can be placed cra-niolaterally and craniomedially or cranially and laterally. True placement of a lateral plate is difficult because of natural cranial-to-caudal bowing of the radius. If plates are applied to the radius, external coaptation proximal to the carpus should be avoided because this changes the tension surface of the radius from the cranial-to-caudal cortex and significantly weakens the mechanical advantage of the repair.
In Salter-Harris type II fractures of the distal radial physis, dynamic condylar screw (DCS) plates and screws should be considered in adult cattle (see Figure 11.2-6). In young calves, lag screw fixation with 5.5-mm cortical screws or 6.5-mm cancellous screws and application of a cast up to the elbow level has also been successful.
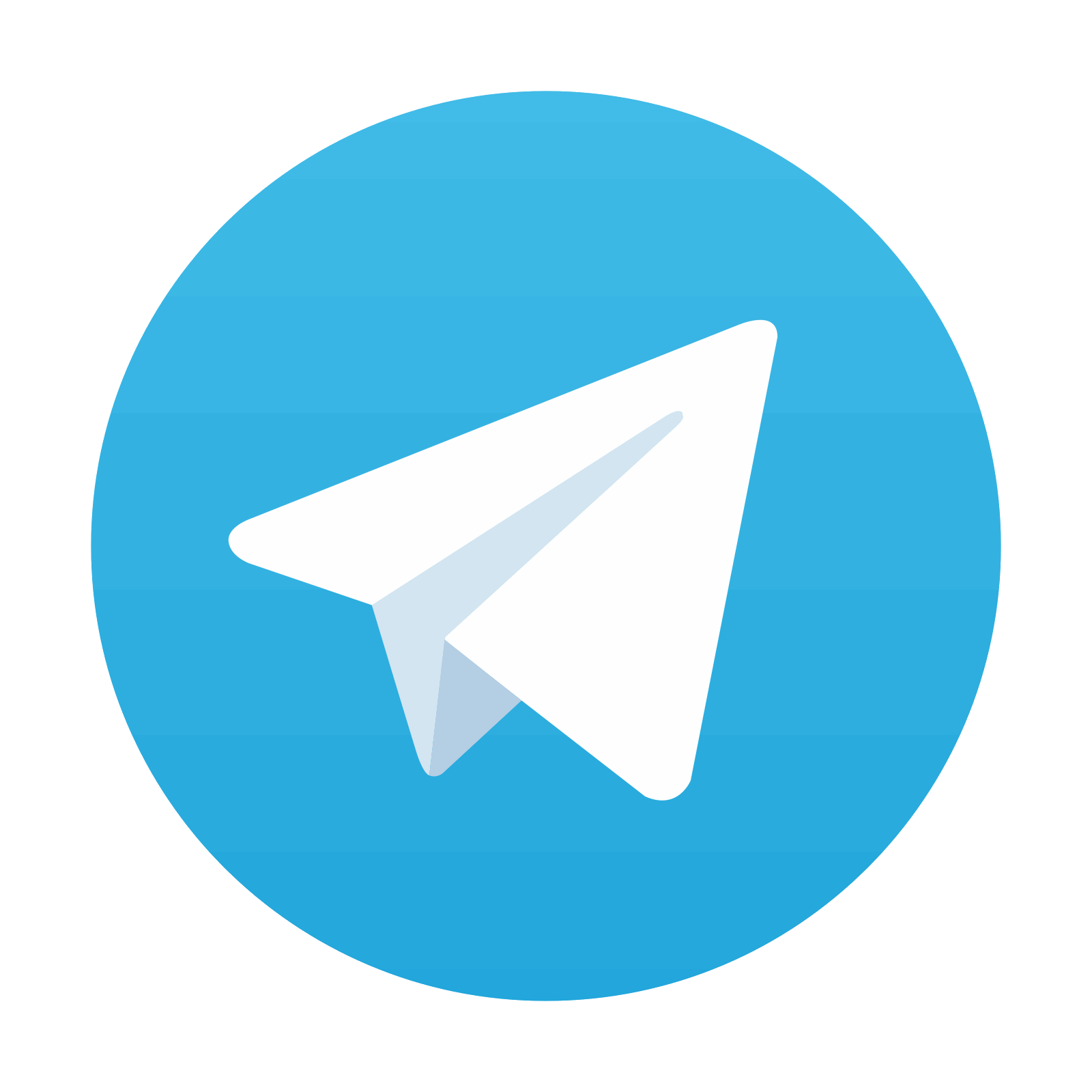
Stay updated, free articles. Join our Telegram channel

Full access? Get Clinical Tree
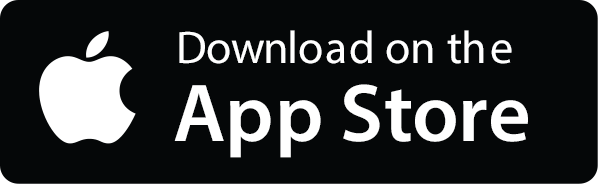
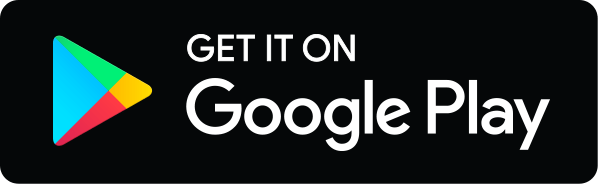