, Debby Van Dam1 and Peter Paul De Deyn1, 2
(1)
Laboratory of Neurochemistry and Behaviour, Institute Born-Bunge, University of Antwerp, Wilrijk, Belgium
(2)
Department of Neurology, Middelheim Hospital, ZNA, Antwerp, Belgium
Abstract
When establishing animal models of Alzheimer’s disease (AD), the aim is to mimic (certain aspects of) the human condition. However, species, strain, and gender specific features interfere with this goal. Only a few species, like primates, dogs, and bears, spontaneously develop histopathological hallmarks of AD. Unfortunately, the use of these species for experimental research is limited by availability, economical and ethical reasons. Commonly used laboratory animals show age-related deterioration, but no AD-pathology. Transgenic models can give solace for this problem. Homology for AD-related genes is discussed for Caenorhabditis elegans, Drosophila melanogaster, Danio rerio, mice, and rats. Pros and cons of species-specific requirements for breeding and housing are argued. Effects of genetic background on pathology should be taken into consideration when studying AD in transgenic mouse models. Cognitive performance and behavior can differ between mouse strains, as well as immunology and vasculature. Strain-specific deficits in auditory or visual acuity or motor impairment can obfuscate measurements, and thus background strains should be selected cautiously. Female mice show greater variation in behavior according to estrus cycling than males, which is why males are often preferred for behavioral research. When studying gender-related differences in AD pathology or risk factors for dementia, both genders are to be compared. Gender-specific differences in learning and memory, aggression, and sexual behaviour should be borne in mind when phenotyping transgenic mice. In conclusion, this chapter provides an overview of background information to keep in mind when working with animal models of dementia.
Key words
Gene homologybreedinghousingcognitionbehaviorneuropathology1 Species Issues
When establishing animal models of Alzheimer’s disease, the aim is to reproduce as many characteristics found in humans as possible. It is obvious, however, that this goal can never entirely be reached due to species-specific characteristics. Pros and contras of different species as animal models of dementia will be discussed in this paragraph.
1.1 Spontaneous Models
There are several species, which have been recognized to exhibit some characteristics of Alzheimer’s disease (AD) neuropathology. Spontaneous plaque pathology was already detected in aged dogs in the 1950s (1). The majority of plaques reported in the canine brain are diffuse, whereas neuritic plaques are rare. Aged canines do not develop neurofibrillary tangles (NFTs) (2). Amyloid β (Aβ) accumulation is related to a decline in global cognitive function and specific types of behavioral tasks are affected (3–6). These features support the use of the canine as a model of the earliest stages of age-related cognitive decline and consequences of Aβ deposition in the absence of NFTs can be determined. This model is termed the canine counterpart of senile dementia of the Alzheimer’s type. The neuropathology of aged cats resembles that of dogs, since Aβ is deposited as diffuse plaques and no mature NFTs are detected (7).
A wide variety of nonhuman primates has been used in aging research with the rhesus monkey as the most studied nonhuman primate (8). Senile plaques have been reported in the brains of various nonhuman primate species, including cynomolgus monkey (9), rhesus monkey (10), chimpanzee (11), marmoset (12), vervet (13), lemur (14), orang-utan (15), and cotton-top tamarins (16). In 2008, NFTs were demonstrated in chimpanzees. These NFTs consisted of dense intraneuronal bundles of paired helical filaments (PHFs) that were identical in size and helical periodicity to those in humans with AD. However, the rarity of these NFTs and the paucity of senile Aβ plaques limits the usefulness of this model (17). No ultrastructural studies have been performed on mouse lemur brains so far. Nevertheless, immunoreactive tau proteins, evidenced using monoclonal antibodies against human PHFs have been detected in the cortex of mouse lemurs. PHF-like material has also been evidenced in the mouse lemurs using immunoblotting (18).
Diffuse plaques as well as NFTs were observed in aged bear (19) and aged polar bear brains (20). In the study of an aged wolverine, senile plaques and NFTs were documented (21).
We have now seen that some mammalian species spontaneously develop plaque pathology and some species even exhibit tauopathies. Species like wolverine and polar bear resemble AD more closely than most species examined to date, but their inaccessibility to laboratory researchers limits the usefulness of these species. For nonhuman primates, long lifespan, cost, and ethical consideration are among the limiting factors for utility. The average and maximal lifespan for rhesus monkeys, for example, is about 25 and 40 years (8); Aβ plaques are known to be present over the age of 20 years (8). Mouse lemurs in contrast are considered as aged from the age of 5 years on (18). NFTs were only found in a 41-year-old chimpanzee (17). Economic factors are an important consideration when selecting a primate model, but the limited availability of research subjects is also a problem. Additional regulations and laws concerning the use of primates that may not apply to other model systems can also shift the choice for a model organism in the direction of lower mammalian species.
Aging rodents do not develop plaques and NFT, but they do show age-related cognitive and behavioral alterations that correlate with AD-relevant neurochemical alterations, such as age-associated cholinergic hypofunction (22). In this sense, aging rodents can help to uncover the switch between normal aging and pathological states. Natural age-associated deterioration has culminated in the senescence-accelerated mouse (SAM), a model which was established through phenotypic selection of AKR/J mice (23). The SAMP8 substrain shows age-related learning and memory deficits, and is therefore useful as a model for dementia (24).
1.2 Transgenic Models
The vast majority of transgenic models have been established in the mouse, but other species can also be used in the search to unravel the pathology of AD. Both the nematode Caenorhabditis elegans (C. elegans) and the fruit fly, Drosophila melanogaster (D. melanogaster), are in the leading positions of invertebrate models of diseases. Their short reproduction time, informed genomics, variety of phenotypes, and the ability to express human genes of interest offer fast ways to screen for functional implications of human gene mutations (25). C. elegans was the first multicellular organism of which the genome was sequenced (26); in the meanwhile, the genome of D. melanogaster is also sequenced (27). The simplicity of these invertebrate models allows identifying protein interactions, which could help to unravel entire regulatory pathways. Basic forms of learning and memory and circadian rhythms can be studied in the fruit fly, but it mainly contributes to the knowledge of molecular and cellular processes. Fundamental aspects of cell biology are quite similar in man and flies, including regulation of gene expression, cell signaling, cell death, membrane trafficking, neuronal connectivity, and synaptogenesis (28). Of course, the fly is a much simpler organism with less sophisticated circulatory systems and cognitive processes. In humans, often redundancy exists, duplicated versions of genes are identified that are present in only one copy in flies. This lack of redundancy can simplify analysis of biological processes in the fly (28). Worms and fruit flies offer the opportunity to generate multiple transgenic lines simultaneously. Their short life span allows screening large numbers of gene mutations in a short period of time.
Rat models have certain advantages over mice, such as their larger size, unique genetics, and well-studied behavioral characteristics. Rats are better suited for microsurgery, cell and tissue transplantation, in vivo functional analyses, and studies that require multiple sampling. Stereotaxic injection is a commonly used method in neurological studies, which is easier to perform with precision in rats than in mice (29). However, less transgenic rat models have been established up to now. Compared to the mouse, rat egg microinjection is more problematic and as a consequence survival rates of microinjected rat eggs are often less than 80% (30). Recent advances in transgenesis of the rat promise the upcoming of more transgenic rat models, including AD models (29,30). It is possible that rats in general, or at least some rat strains, are more resistant to Aβ deposition than most strains of mice. Nevertheless, Flood et al. succeeded in the construction of a triple transgenic rat model in Sprague–Dawley rats with Aβ deposits similar to those observed in the transgenic mouse models of AD. Extracellular deposits were found at 7–9 months of age (31). Due to reduced fertility of inbred rat strains, generation of a transgenic model in inbred rats is more difficult to achieve than in outbred rat strains. The use of inbred rat strains, however, minimizes the individual variation among transgenic rats. Some attempts were made to produce inbred transgenic rat models. Fischer 344 rats with expression of the Swedish amyloid precursor protein (APP) mutant gene show a surprising improvement in hippocampal-dependent learning and memory tasks, and only mild increases in brain APP mRNA with no extracellular deposits (32). Another inbred rat model was established by lentiviral infection of Fischer 344 zygotes. This APP21 rat model shows three times greater cerebral APP expression compared to wild-type rats, but no extracellular plaque deposition is demonstrated either (29).
The zebrafish (Danio rerio) is an effective and simple model organism for studies of developmental and neurological disease processes. Since the zebrafish is a vertebrate, it is more closely related to humans than invertebrate models such as worms or flies. It is an advantageous model for genetic studies as it is genetically malleable by injection of morpholino antisense oligonucleotides, mRNA, or transgenes. These techniques make it possible to make subtle or drastic changes in gene expression and observe the influences in the developing transparent embryo (33).
1.3 Breeding and Housing
Physiological and anatomical features of primates are strongly dependent on the species. For lemurs, gestation takes 2 months, and the animals breastfeed for 6–8 weeks. In the wild, female animals usually have litters twice a year; in captivity, typically only once. One female animal has one to four babies per litter (18). Rhesus monkeys have a gestation of 5 months, in chimpanzees this can take 8 months. Latter species usually has only one baby per litter (34). Cage dimensions are dependent on the species, but the minimum floor surface can go to 2.5 m² and minimum height of 2 m for chimpanzees.
Dogs need relatively large cages, dependent on shoulder height. Females are ready for breeding when they are older than 12 months. Gestation lasts around 65 days and litters contain three to six animals (35). Dogs of 8 years and older can be considered middle-aged and old (6).
Breeding in mice goes relatively quickly. Mice are capable of breeding from the age of 8–10 weeks on. Female animals are polyestric, which means there are several estral cycles in a year. When groups of mice are housed without males, anestrus occurs. The introduction of a male at this time (or male pheromones) will lead to estrus synchronization, termed the Whitten effect (36). After a successful copulation, no implantation will occur if the female is introduced to another male within 24 h after mating, known as the Bruce effect (37). Until 24 h after mating, a coagulation plug can be found in the vagina. This is a very simple method to confirm mating. Pups are born blind and naked after a gestation of 18–22 days. After 3 weeks, the pups can be weaned, but survival and general health of the pups is better when weaning occurs at 4 weeks of age (38). When mating takes place during postpartum estrus, lactation and gestation can coincide. Lactation can impair the implantation of zygotes, which prolongs the gestation with 3–5 days. Mice can be housed in groups, but it is possible that males become aggressive when housed together. Individually housed animals are more aggressive than permanently group-housed mice (35).
Rats are less aggressive than mice. Males can be housed together without many problems, but large groups of adult animals may lead to territorial aggression. Too many rats in one cage can lead to death by hyperthermia. Reproduction is comparable to the mouse, although the Bruce effect does not occur in rats and estrus synchronization by pheromones is less clear. Synchronization of estrus and gestation can be induced by the administration of progesterone during 4 days (causes anestrus), followed by the administration of pregnant mare serum gonadotrophin, which make most females go to estrus. The estral cycle is light sensitive; lights on 12–16 h per 24 h results in optimal breeding results. Rats are ready for breeding when aged 12–16 weeks. Gestation takes 21–23 days and litters consist of 6–12 pups (39).
Housing requirements and recommendations for commonly used laboratory animals are described in literature in detail, e.g. (40).
C. elegans progresses from embryo to larva to fertile adult in 3 days at room temperature. A single adult can have between 300 and 1,000 progeny. C. elegans primarily exist as self-fertilizing hermaphrodites with the occasional occurrence of males. This is very useful for genetics: the hermaphrodites can maintain homozygous mutations without the need for mating, and males can be used for genetic crosses. C. elegans are grown on nematode growth medium agar plates, covered with a special strain of bacteria that provides the necessary nutrition. At any time, the animals can be cryogenically preserved (41).
The lifespan of D. melanogaster is about 30 days. The developmental period varies with temperature. The shortest development time is achieved at 28°C and takes 7 days. At higher temperatures, development time increases due to heat stress. Females lay about five eggs at a time, into rotting fruit or other suitable material. The eggs hatch after 12–15 h. The resulting larvae grow for about 4 days. During this time, they feed on the micro-organisms that decompose the fruit, as well as on the sugar of the fruit itself. Then, the larvae encapsulate in the puparium and undergo a 4-day-long metamorphosis, after which the adults emerge. Females become receptive to courting males at about 8–12 h after emergence (42,43).
1.4 Gene Homology
1.4.1 App
The human APP cDNA was first cloned in 1987 (44–46) and homologous genes are being identified ever since. cDNA sequences have been published for monkey, rat, mouse, frog, chicken, polar bear, pig, rabbit, guinea pig, sheep, and other species. The homology percentages are depicted in Table 1 for the most important species in which models for AD are established. An APP-related gene, Appl, has been identified in D. melanogaster (47). Furthermore, in C. Elegans, an APP-like sequence, apl-1, has been identified (48). Both these species lack, however, a recognizable Aβ sequence. The amino acid sequence of the 43-amino acid Aβ peptide sequence is identical in dogs, polar bears, monkeys, and humans (49). Additionally, amyloid precursor-like proteins (APLPs) have also been identified; i.e., APLP1 sequences from human and mouse, as well as human, mouse, and rat APLP2 sequences. There are three conserved domains between the invertebrate and mammalian APP homologues. These domains correspond to exons 2–5 (D1), 9–13 (D2), and 17–18 (D3; cytoplasmic domain) of human APP. Obvious differences between family members are noted: the recruitment of the Kunitz Protease Inhibitor-domain or exon 8 in both APP and APLP2 members, inclusion of exon 9 (OX-2 homology in human APP); and the variability both in sequence and length of the protein between each of the three conserved domains (50). Deletion of Appl in D. melanogaster results in relatively subtle behavioral changes, such as defects in fast phototaxis, but no morphological defects. These deficiencies can be partially complemented by the expression of human APP, suggesting significant functional conservation (51). Two homologues of APP, appa and appb, have been identified in zebrafish. Both genes have approximately 70% amino acid identity to human APP-695, with 80% identity in the Aβ1–42 region and 95% identity within the transmembrane domain (52).
Table 1
Gene homology in the APP gene
Gene | Identity (%) | ||
---|---|---|---|
Species | Symbol | Protein | DNA |
Homo sapiens | APP | ||
vs. Pan troglodytes | APP | 94.5 | 95.4 |
vs. Canis lupus familiaris | APP | 91.8 | 87.6 |
vs. Mus musculus | App | 97.4 | 89.3 |
vs. Rattus norvegicus | App | 92.2 | 85.9 |
vs. Danio rerio | appa | 73.6 | 70.8 |
vs. Caenorhabditis elegans | apl-1 | 35.3 | 46.9 |
1.4.2 Presenilins
The proteins encoded by the presenilin (PS) genes are 467 (PS1) and 448 (PS2) amino acids long. There is a strong sequence homology between PS1 and PS2 and between homologues of different species, as shown in Tables 2 and 3. Presenilin homologues were found in a variety of organisms, not only in mammals or vertebrates, but even in species as distantly related to humans as nematodes and fruit flies. Three presenilin homologues have been identified in C. elegans: Sel-12 (51), HOP (homologue of presenilin)-1 (53,54), and SPE (spermatogenesis defective)-4, a more divergent member of the presenilin family (55). Presenilins are functionally conserved between humans and C. elegans. The egg-laying defective phenotype of Sel-12 C. elegans mutants can be rescued by the expression of human PS1 (56,57) or HOP-1 (54). In contrast to the other two homologues, which are expressed somatically during all developmental stages in nearly all tissues (53,56), the third PS homologue in C. elegans, SPE-4, is exclusively expressed during the larval stage in spermatogenesis (55,58) and possibly plays a role in preventing spermatid activation (59). D. melanogaster has only one PS homologue, psn (55,58). Orthologues of PS1 and PS2 have been identified in zebrafish, namely psen1 (60) and psen 2 (61). The primary structures are highly conserved, as shown by sequence alignment, though there are highly variable regions at the N-terminus and the C-terminal half of the cytoplasmic loop domains. Transcripts from zebrafish psen1 are ubiquitously expressed from fertilization, implying zebrafish psen1 has an essential function throughout embryonic development (60). Zebrafish psen2 mRNA is present from fertilization, but protein expression has only been detected from the onset of gastrulation (61).
Table 2
Gene homology in the PSEN1 gene
Gene | Identity (%) | ||
---|---|---|---|
Species | Symbol | Protein | DNA |
Homo sapiens | PSEN1 | ||
vs. Pan troglodytes | PSEN1 | 100.0 | 99.9 |
vs. Canis lupus familiaris | PSEN1 | 94.0 | 91.9 |
vs. Mus musculus | Psen1 | 92.7 | 87.2 |
vs. Rattus norvegicus | Psen1 | 92.9 | 88.0 |
vs. Danio rerio | psen1 | 74.5 | 68.0 |
vs. Caenorhabditis elegans | sel-12 | 54.5 | 56.1 |
Table 3
Gene homology in the PSEN2 gene
Gene | Identity (%) | ||
---|---|---|---|
Species | Symbol | Protein | DNA |
Homo sapiens | PSEN2 | ||
vs. Pan troglodytes | PSEN2 | 99.8 | 99.3 |
vs. Canis lupus familiaris | PSEN2 | 96.0 | 90.7 |
vs. Mus musculus | Psen2 | 95.5 | 89.3 |
vs. Rattus norvegicus | Psen2 | 95.1 | 89.4 |
vs. Danio rerio | psen2 | 74.0 | 69.5 |
vs. Drosophila melanogaster | Psn | 67.7 | 66.7 |
1.4.3 Apolipoprotein E
Apolipoprotein E (ApoE) is a multifunctional lipoprotein made by the liver and by brain astrocytes, which is found in the blood and in cerebrospinal fluid. It has an important role in the transport of cholesterol to steroid-producing cells.
The primary structures of ApoE from several species, including human (62), baboon (63), cynomolgus monkey (64), rat (65), mouse (66), guinea pig (67), rabbit (68), cow (69), dog (70), and sea lion (71), has been determined. They range in length from 279 to 310 amino acid residues for guinea pig and sea lion ApoE, respectively. Overall, there is a high degree of sequence conservation across mammalian species, as can be seen in Table 4, with notable exceptions at the amino and carboxyl termini (72).
Table 4
Gene homology in the APOE gene
Gene | Identity (%) | ||
---|---|---|---|
Species | Symbol | Protein | DNA |
Homo sapiens | APOE | ||
vs. Pan troglodytes | APOE | 97.2 | 98.4 |
vs. Canis lupus familiaris | APOE | 71.2 | 82.9 |
vs. Bos taurus | APOE | 72.2 | 81.8 |
vs. Mus musculus | Apoe | 73.7 | 78.7 |
vs. Rattus norvegicus | Apoe | 71.3 | 77.7 |
vs. Danio rerio | apoeb | 30.2 | 47.4 |
In humans, ApoE has several genetic variants, or isoforms (apoE ε2, ε3, ε4), with the ε3 allele being the most abundant, about 77% in Caucasian populations. The other apoE alleles, ε4 and ε2, comprise about 15% and 8%, respectively (73). On the contrary, primates have only one ApoE isoform that most resembles ε4 (74), like most animals (72). This suggests that the ε4 allele is the common ancestral gene, despite the high abundance of the ε3 allele in humans (73). ApoE ε4 represents the most common risk factor for AD (75) and cardiovascular disease (76). It appears that worms and flies do not have an ApoE gene (77).
1.4.4 Tau
Microtubule-associated protein (MAP) tau, MAP1 and MAP2, are the three major microtubule-associated proteins of a normal mature neuron. These MAPs apparently perform similar functions, i.e. the promotion of assembly and stability of microtubules. In AD and related disorders, tau is abnormally hyperphosphorylated and accumulates as paired helical filaments (78).
D. melanogaster models of tauopathy have been reported. The tau homolog of D. melanogaster has been cloned and characterized (79). Deletion of the fly homolog of tau does not result in any detectable phenotype (80). C. elegans possesses a structural microtubule-associate protein, termed PTL-1 (81). A tau-like protein, XTP, has also been sequenced and biochemically characterized in one amphibian, Xenopus laevis (82). MAP has been identified in numerous mammalia, including Mus musculus (83). Despite the presence of an endogenous tau in most species, investigators have opted to overexpress human tau in order to establish tauopathy models.
2 Strain Issues
Given the abundance of transgenic mouse models of dementia, this chapter will focus on strain and gender differences in this species, though some of the information given will be generally applicable and can be translated to other species, like rats or other mammalia.
2.1 Effects of Genetic Background
2.1.1 Aβ Deposition
Studies have been undertaken to examine the effects of defined genetic background on APP metabolism, Aβ metabolism, and Aβ deposition in transgenic mouse models of AD. Lehman et al. transferred the transgene with Swedish mutation into three different genetic backgrounds by repeated backcrossing into the inbred mouse strains C57Bl/6J, DBA/2J, and 129S1/SvImJ for ten generations. They show that genetic background impacts several steps leading from APP processing to Aβ metabolism and Aβ deposition. First, the choice between α- and β-secretase cleavage in APP processing was significantly different. Second, levels of Aβ40 and Aβ42 in brain and plasma varied among the congenic lines, with highest levels in the C57Bl/6J background, despite equivalent levels of holo-APP. Analysis of B6 × D2 revealed that C57Bl/6J alleles were dominant over the DBA/2J alleles, resulting in high Aβ40 an Aβ42 levels. C57Bl/6J and B6 × D2 strains exhibit enhanced Aβ deposition. These data demonstrate that significant phenotypic alterations in Aβ metabolism and deposition are conferred by genetic background (84). To map the genetic loci responsible for the observed heritable differences in brain Aβ levels, a dense whole-genome scan in the B6 × D2 background strain was performed, which revealed genomic regions associated with brain Aβ levels. Several of these regions contain biologically interesting candidate genes and are syntenic to regions with indications of potential linkage to human AD risk (85).
Overexpression of APP produces dramatically different phenotypes in transgenic mice depending on the genetic background. Concentrations of APP sufficient for amyloid plaque deposition at 1 year are lethal on susceptible backgrounds, such as C57Bl/6J and FVB/N mice. These effects cannot be subscribed to a decrease in Aβ peptide concentrations in the brain in the more resistant strains (D2 × FVB, B6 × FVB and CAST × FVB), since these were similar, thus modulating genes are to be sought (86). The effect of genetic background was proven to be of influence in the TgCRND8 model too (87). The importance of genetic backgrounds in transgenic AD models was demonstrated as well, by the APP immunoreactivity, which segregated more closely with the B6/SJL genotype than with the presence of the transgene (88).
2.1.2 Tau Models
Mutations in the Disabled-1 (Dab1) gene lead to hyperphosphorylation of endogenous mouse tau. Dab1 mutants are viable in BALB/c but nonviable in 129Sv or in 129Sv × B6 (89). Genetic background genes thus modify the survival of mutants. Some chromosome regions have been identified to affect tau hyperphosphorylation in Dab1-/- mice. In these regions, homologs of several genes associated with human AD are found (89).
2.2 Cognitive and Behavioral Performance
The phenotype of a transgenic mouse is not only the result of the targeted gene, but it also reflects interactions with background genes. Thus, genetic background should be as carefully controlled as any other experimental variable. The simplest way to do this is to derive and maintain mutations in an isogenic genetic background. However, not all isogenic backgrounds are appropriate for a given study, since the behavioral characteristics of certain isogenic strains could overshadow the effects of the introduced gene mutations. Since natural strain differences exist for behavioral traits, the genetic background of the inbred mouse strains must be carefully considered in the interpretation of behavioral phenotypes of transgenic mice (90).
Learning and memory capacity are the traits of primary interest in dementia models. Luckily, the performance of inbred mouse strains on a wide variety of paradigms is well documented. It is important to discern between true learning differences and sensory impairments that lead to poor performance. Visual acuity is necessary for spatial learning tasks (91). Albino mouse strains demonstrate poor vision under bright lights and some mouse strains have the retinal degradation gene, which leads to blindness in adult mice. Auditory function is important for paradigms involving conditioning in the presence of a tone; some strains show deafness as a function of age (92–94). Analogously, the role of strain differences in motivation needs to be dissociated from true learning and memory differences.
The Morris water maze is frequently used to examine spatial learning in rats and mice (95). Animals are trained to locate a hidden platform in a circular pool filled with opaque water, using distal room cues. Latency to locate the platform and path length are recorded in each trial. The performance in a probe trial, in which the platform is removed, is examined. C57BL/6J and C57BL/10J are good learners in contrast to 129Sv/J, DBA/2, and BALB/c (96,97). The Barnes maze is a dry land maze in which the animals escape from a brightly lit, exposed circular open field into a darkened box that is hidden beneath one of the 18 holes around the perimeter of the open field. This test for spatial learning and memory is physically less demanding and probably less stressful than the Morris water maze. There was no evidence of strain differences between 129S6, C57BL/6J, and DBA/2J mice during acquisition in the Barnes maze. During the probe trial, C57BL/6J mice made more visits to the target hole than any of the nontarget holes, thus showing the best performance on this test. 129S6 mice performed the worse and DBS/2J mice showed an intermediate profile (98). Spatial memory has also been examined in eight-way radial arm maze tasks, in which animals must remember the arm of a maze in which they previously obtained a food reward. In this paradigm, C57BL/6J and DBA/2 prove to be better learners, while BALB/c and C3H/He perform significantly poorer (99,100). Brooks et al. have also shown that the C3H/He strain failed to acquire a visual discrimination task, but this may be due to a visual deficit in these animals (101). Contextual fear conditioning is based on the association of a shock paired with a tone. Bouts of behavioral immobility, termed “freezing” are used as a measure of performance. Again C57BL/6J and C57Bl10/J prove to be good learners, but also 129/SvJ and BALB/cByJ are among the good learners in this paradigm. FVB/NJ and DBA/2 do not show greater freezing rates in the training context versus the altered context (96). Mouse strain differences were shown as well in long-term fear memory (102), operant learning (103), lever press escape/avoidance conditioning (104), object recognition (105), and social transmission of food preference (98). The best choice of an inbred background on which to explore the impact of a mutation on learning capacities appears to be C57BL/6. These mice are moderate learners, such that either impairment or improvement could be observed.
Motor activity underlies almost every mouse behavioral paradigm. Dysfunctions in physical movement can produce false-positive and false-negative effects on behaviors of interest for transgenic mice. Automated registration of open field activity can measure the total amount of movement, rate of movement, and type of spontaneous activity. The open field test is the simplest way to evaluate emotional behavior. Decreased ambulation and increased defecation in a brightly lit open field indicate heightened emotionality (106,107). Rearing behavior decreases in an anxiogenic environment, and thigmotaxis, i.e. the proportion of time the animal remains close to the walls of the open field, increases (108). In general, the C57 inbred strains of mice, including C57BL/6, C57BL/10, C57BR, and C57L, consistently show high levels of open field locomotion and low levels of anxiety-related measures in the open field. Intermediate strains include the DBA/2, CBA, AKR, and LP. Strains typically exhibiting low locomotor activity and high levels of emotional reactivity include DBA/1, BALB/c, and A/J. Albino strains are overrepresented at the high anxiety end of the distribution. This could be partly due to the added stress of high illumination in albino mice (108).
Tests of intermale aggression in the mouse include the “resident-intruder” paradigm where males are individually housed during several weeks. For the test, an opponent male from a strain, selected for the passivity under attack, is introduced in the cage. Numbers of attacks are counted. There are robust differences among inbred mouse strains in levels of aggressive behavior: the NZB/B1NJ and AKR mice are extremely aggressive, C57BL/10 and C57BL/6 mice exhibit markedly lower proportions of attacking males, and other strains show intermediate levels of aggression (109). Other articles provide information on strain distributions and male offence (110–113). Aggression in females is discussed in the section about gender issues.
< div class='tao-gold-member'>
Only gold members can continue reading. Log In or Register a > to continue
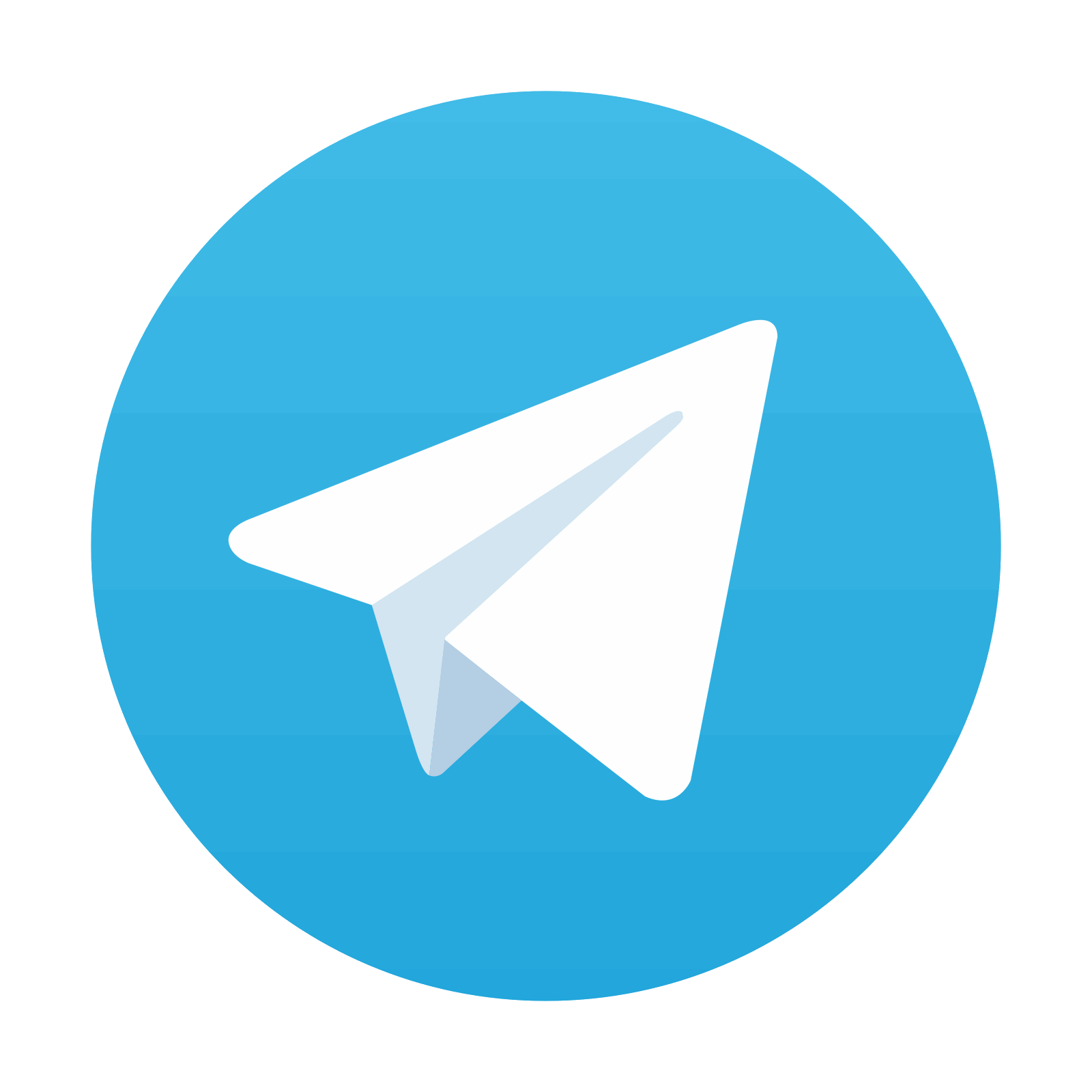
Stay updated, free articles. Join our Telegram channel

Full access? Get Clinical Tree
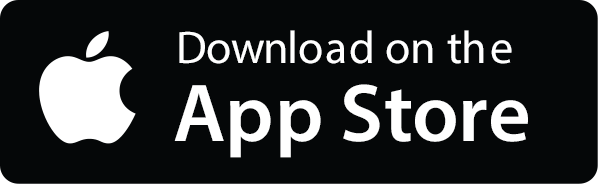
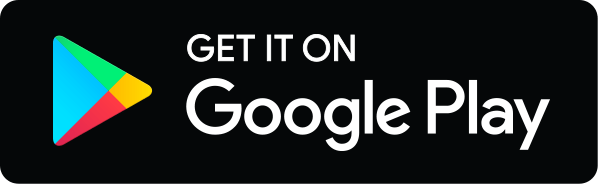