(1)
Department of Experimental Psychology, University of Oxford, Oxford, UK
Abstract
The search for a good animal, preferably mouse, model of Alzheimer’s disease (AD) is one of the most imperative in present medical research, given the increasing prevalence of this disorder in an aging population and its enormous social, economic, and personal impact. In 1996, Karen Hsiao and colleagues showed that transgenic mice, which overexpress a human APP cDNA transgene from a Swedish family whose members showed early onset, familial AD (Tg2576 mice) were cognitively impaired at around 10 months of age and also showed pathophysiological characteristics of AD, notably deposits of amyloid. Much work followed this original publication, yet even now there is only limited consensus as to when, and to what extent, cognition is impaired in this model. This work is reviewed here, as well as research on the neuropathophysiological mechanisms by which the mice become cognitively impaired. The fundamental validity of the Tg2576 model and the role of β-amyloid in the disease processes seen in both Tg2576 mice and humans with AD are also considered.
Most research suggests that Tg2576 mice are moderately cognitively impaired by 12 months of age, and this laboratory therefore first started work on a cohort this age. Further work was done on cohorts aged 3, 9, and 21 months. Marked cognitive deficits were found in three paradigms: reference memory in a Y-maze motivated by escape from shallow water (paddling Y-maze); social memory for a juvenile mouse; and habituation to an open field over 24 h. Only the former has so far been shown unambiguously to be age-dependent. The latter two tests are simple to perform, so hold great promise as potential screens for new therapeutic agents. Reference memory in an appetitively motivated T-maze with both response and visual cues may also reveal cognitive impairments of an acceptable magnitude. However, the development of a new Tg2576 line based on a 129 mouse substrain with good behavioral characteristics may hold the greatest promise for future test development.
Key words
Tg2576dementiaAlzheimer’s diseaseMiceTransgenicb-amyloidBehavior1 Introduction
In 1906, a German physician, Alois Alzheimer, presented the results of a postmortem examination of the brain of one of his patients, 55-year-old Auguste Deter, at a meeting in Tubingen. The following year, a publication followed, describing the characteristic pathology of the disease, which was named after him by Emil Kraepelin (1–3). Ninety years after the initial recognition of this disease, behavioral and pathophysiological results from the Tg2576 mouse model of Alzheimer’s disease (AD) were reported (4). Since then, two major themes have developed. First, despite this model being over 12 years old, there is still disagreement as to when and to what extent cognitive impairments arise. Second, many other genetically modified models of AD have been developed, some of which may better model the disease and its neuropathology. This latter area will not be addressed in detail in the present chapter, which will concentrate on the behavioral and neuropathophysiological characteristics of the Tg2576 mouse, and discuss its validity as a model of AD. This mouse is also called APPsw, APP695SWE, or most completely Tg(HuAPP695.K670N/M671L)2576. A similar transgenic construct is known as the APP23 mouse; it shows greater overexpression of soluble β-amyloid (Aβ) than the Tg2576 mouse.
Sporadic AD accounts for the majority of Alzheimer’s patients. The incidence increases with age, beginning around 60 (1%). By age 85, up to 30% of the population may be affected. Familial AD, around 10% of all AD cases, has an earlier onset and originates from genetic abnormalities. A germane example is a large Swedish family, which suffers from a double mutation in the gene expressing amyloid precursor protein (APP). This mutant gene was used to create the Tg2576 mouse model of AD.
Both types of AD are characterized by a progressive loss of memory and the ability to perform activities of daily living, such as making a meal, selecting the correct TV channel, telling the date, tying up shoe laces, and shopping. Only much later do motor abnormalities sometimes develop. Psychotic episodes and delusions also can occur, for example Alzheimer’s first patient would sometimes scream that someone was trying to kill her. Examples of Capgras syndrome even happen, where the patient believes that a close relative, spouse, or friend is an impostor. Death is generally due to systemic illnesses such as pneumonia. Patients with AD may also be affected by vascular dementia caused by multiple small strokes.
The pathological hallmarks of AD are intercellular amyloid plaques (especially dense cored ones, which are unique to AD) and intracellular neurofibrillary tangles formed by hyperphosphorylation of an intracellular protein called tau, which is associated with microtubules. Neuronal loss and brain atrophy are also characteristic of this disease. Indeed, the progressive thinning of the medial temporal lobe, as measured by imaging techniques, is closely correlated with disease severity (5, 6).
2 Validity of the Model
This mouse bears the “Swedish” familial AD mutation, in which there is a double amino acid substitution in APP, Lys 670 to Asn and Met 671 to Leu. This results in overproduction of Aβ (11), and several changes characteristic of AD, such as plaques, increased levels of both soluble and insoluble Aβ, and abnormal synaptic plasticity (4, 12). Microglia are activated (13), which leads to inflammation (14). In addition, oxidative stress is increased (15).
However, many features of AD are not faithfully reproduced. Notably, there is relatively little change in the cholinergic system (16), although it has been reported that cholinergic regulation of hippocampal neuron excitability was impaired in Tg2576 mice (17), and cholinergic neurotransmission was reduced (18). Moreover, Aβ is a powerful activator of α7 nicotinic cholinergic receptors (19). Increased glycosylation of acetylcholinesterase was seen in Tg2576 mice at 4, 8, and 12 months of age (20). Impaired REM sleep was seen in Tg2576 mice at 6 but not 2 months, correlating with a reduction in the numbers of pedunculopontine tegmentum choline acetyltransferase-positive neurons (21). This reduction was selective; however, other cholinergic groups involved in REM sleep were unchanged.
Neurofibrillary tangles are not seen in Tg2576 mice, and little neuronal loss or gross brain atrophy occurs (22). The amyloid peptides found in Tg2576 mice are also chemically and physically different from those found in AD (23).
Moreover, familial AD is a small proportion (10% or less) of the total number of cases of AD, the majority being of the sporadic type. Thus, the Tg2576 mouse, derived from a familial AD model, cannot identically replicate most forms of AD. However, since excess Aβ production and deposition always occurs in AD, the mechanism by which this occurs may not be crucial.
A convincing model of AD, in terms of face validity, should show age-related changes in both cognition and neuropathology, and these should be temporally correlated. There seems to be better agreement as to when changes in neuropathology occur than when behavior changes; most researchers report significant neuropathology by 10–12 months of age (4, 24, 25).
2.1 Is Amyloid Causing the Behavioral Changes, and If So, in What Form?
The Tg2576 mouse was originally created to test the “amyloid hypothesis,” namely that the deficits in AD are due to amyloid (rather than, for example, neurofibrillary tangles related to hyperphosphorylation of tau protein) (9). Originally, the amyloid being considered was in the form of plaques, which are easily identifiable at the histological level. Indeed, along with neurofibrillary tangles, these were the classical markers of AD established by Alois Alzheimer in 1907 (1). Plaques can exist as both diffuse and dense-cored forms. However, this hypothesis has fundamental flaws and has been heavily criticized (9); amyloid plaque load does not correlate with cognitive decline in AD (26, 27), nor does plaque count correlate with cognitive deficits in mouse AD models (28, 29), although see (30). Indeed, deficits in contextual fear conditioning have been seen in Tg2576 mice at 5 months of age (19, 31–33), when Aβ levels are elevated but plaques are not yet present (4).
At first, fibrillar forms of Aβ were thought to be responsible for AD pathology (34–36), although McLean et al. suggested that they might be soluble forms (37).
Increasingly, accumulating evidence suggested that soluble rather than aggregated plaque amyloid might be primarily involved in AD (38–40). Westerman and colleagues were the first group to publish in vivo evidence that smaller forms of amyloid were involved in cognitive decline in Tg2576 mice (41). Hardy and Selkoe (42) noted that the extent of dementia in AD correlated better with amyloid assayed biochemically (which also detects soluble forms of amyloid) than with histologically determined plaque counts. Palop et al. (43) found that cognitive impairments correlated with the relative abundance of Aβ, but this did not depend on the amount deposited as plaques. At present, oligomeric and soluble forms of Aβ are the prime candidates for causing the cognitive alterations in AD and the mouse models of it. Soluble Aβ is present as early as 4 months in the Tg2576 model (20).
There is increasing evidence that oligomeric (trimers to dodecamers) forms of Aβ are primarily responsible for its toxicity, rather than the insoluble fibrils that are found in amyloid senile plaques (44, 45). Deposits of plaques and insoluble forms of amyloid may be merely end stages in a degenerative process that is initially started by oligomeric forms of amyloid (45–47). Oligomeric rather than monomeric or fibrillar Aβ inhibits the activity of calcineurin, a phosphatase that is elevated and correlated with memory dysfunction in Tg2576 mice, due to inhibition of the transcription factor CREB (44). All soluble amyloid oligomers share a common structure, which probably mediates their toxicity, since an oligomer-specific antibody blocks their action (46, 48). The distribution of soluble amyloid oligomers in human AD brain is distinct to that of fibrillar amyloid. In control, nondemented brains with cored plaques, oligomer-specific antibody staining was almost absent (48). The key element in Tg2576 mouse cognitive deterioration may be a 56-kDa soluble Aβ oligomer, the concentration of which closely temporally mirrors the behavioral impairments (49). These authors noted that (in their work; but see later) the cognitive impairment in a Morris water maze first became evident at 6 months of age, yet remained stable for months afterwards, a time when the concentration of all other species of amyloid was increasing rapidly. However, Dineley et al. (33) noted that the 56-kDa oligomer is present at 6 months in Tg2576 mice but absent 1 month earlier, although deficits in contextual fear conditioning existed at this time point.
Further evidence that amyloid, or at least in most cases the insoluble fibrillar forms, may not be crucial in cognitive impairment is that the acetylcholinesterase inhibitors, physostigmine and donepezil, improved cognition without affecting Aβ deposition. Although donepezil may influence Aβ characteristics and effects under some circumstances, in the latter experiment it appeared not to (50). Treatment with Gingko biloba reduced spatial learning, and memory deficits yet had no effect on the deposition of hippocampal Aβ plaques (51). Dietary supplementation with the antioxidant α-lipoic acid produced improvements in cognition in treated Tg2576 mice without affecting levels of soluble or insoluble amyloid. (52). Moreover, levels of soluble Aβ are substantially elevated in the brains of Tg2576 mice by the age of 6–8 months, when substantial cognitive changes are often not reported, while by 10–11 months, amyloid plaques have started to form (4) and most researchers report behavioral impairments by then. At 16 months, there is a 4–8% amyloid burden in hippocampal regions of the Tg2576 mouse brain (53), which is a similar amyloid burden to that found in human AD brains, and almost all researchers agree that the mice are then cognitively impaired.
However, chronic nicotine treatment reduced amyloid load in Tg2576 mice but only the insoluble forms, including plaques, were affected; soluble amyloid was not reduced (54). Unfortunately, no behavioral work was done to see if nicotine reduced cognitive deficits.
Further evidence that Aβ is not crucial to the memory deficits in mouse models of AD is that vaccinated PDAPP mice improve in both an object recognition task and a holeboard learning and memory task without a change in amyloid load (55). Tg2576 mice lacking the catalytic subunit Nox2 of NADPH oxidase did not show the normal oxidative stress, cerebrovascular dysfunction, or behavioral deficits normally seen at 12–15 months, yet these improvements occurred without reductions in brain Aβ levels or amyloid plaques (56).
However, some reports suggest that amyloid burden is related to cognitive deterioration in Tg2576 mice. Asuni et al. (57) showed that vaccination of Tg2576 mice with an Aβ derivative from age 11 to 24 months reduced amyloid burden by approximately 30%, while cognitive performance on the radial maze and a modified version of the Hebb-Williams maze improved to wild-type levels. Although this overall result suggests a causative role for Aβ in cognitive impairment, this was not supported by the fact that cognitive performance was not correlated with reductions in amyloid levels between individual mice. The authors suggested that oligomeric forms of Aβ might be the moiety responsible for cognitive impairment. Jensen et al. (58), working on the APP/PS1 double transgenic mouse model, also suggested that their amyloid vaccine attenuated the accumulation of small oligomeric forms of amyloid. They obtained ambiguous results, in that, while chronic immunotherapy reduced cognitive impairment at 15–16.5 months of age, there was no decrease in (or correlation to) amyloid deposition. Yet, factor analysis showed that brain amyloid deposition measures loaded heavily with key cognitive measures. Morgan et al. (59) also showed a partial reduction in amyloid burden and improved cognition when Tg2576 mice were vaccinated with Aβ. Although an overall decrease in Aβ was not seen following treatment with an Aβ antibody, the inverse relationship between amyloid load and cognitive function was eliminated, suggesting that the two were related (60). In an experiment comparing immunization of Tg2576 mice with two different Aβ derivatives, cognition on a radial arm maze was improved in both groups but only one showed a reduction in amyloid plaques (61). The phosphodiesterase-4 inhibitor, rolipram, attenuated impaired contextual fear conditioning at 5 months of age in Tg2576 mice, but the authors concluded that the effect of rolipram was independent of amyloid as rolipram-treated controls also improved (31). However, the γ-secretase inhibitor N-[N-(3,5-difluorophenacetyl)-Lalanyl]-S-phenylglycine-t-butylester also attenuated learning impairment but this was not seen in the controls and was accompanied by reduced brain Aβ load. Exercise (3 weeks on running wheels) produced significant decreases in soluble Aβ40 and soluble fibrillar Aβ in 17- to 19-month-old Tg2576 mice (62). Exercise has been reported to delay the onset of dementia and AD in people over 65 (63).
2.2 At What Age Does Cognitive Impairment Occur in the Tg2576 Mouse?
The Tg2576 mouse shows an impairment in cognition, which is similar to, but (at least initially) less severe than, that caused by complete hippocampal lesions (64). In line with the role of the hippocampus in spatial learning and memory (65), and the extensive Aβ deposition occurring in this brain region in Tg2576 mice (66), it is spatial forms of learning that are primarily affected. Indeed, some forms of memory are preserved (33, 67–69) or even enhanced in Tg2576 mice, probably due to elimination of competing hippocampally driven response tendencies (70). King et al. (69) found no impairment in active or passive avoidance at 3 and 9 months; in our group, no impairment in passive avoidance was seen even at 22 months (71). However, King et al. also failed to detect impairments in water maze acquisition at 9 months, a time when most groups find deficits. In a version of the Barnes maze, they found impairments between wild-type and Tg2576 mice as early as 3 months in females, but neither males nor females were impaired at 9 months in this paradigm. This ambiguity may have arisen because this paradigm does not show very steep learning curves.
The Morris water maze is a standard test of spatial memory. Hsiao et al. (4) found that Tg2576 mice were impaired at 9–10 months, while Westerman et al. (41) and Lesne (49) found impairment at 6 months. King et al. (69) observed deficits in water maze retention at 3 months of age; Arendash et al. (72) showed a comparable water maze deficit in 5-month-old Tg mice. Arendash and King (73), however, failed to find any deficit on water maze acquisition and retention from 3 months to 19 months. King et al. (74) observed deficits on a visible (i.e. nonspatial) water maze in 9-month-old mice, while Horgan et al. found impairment in this paradigm at 6 months. Using a circular platform maze derived from the Barnes maze, where a mouse can escape from an aversive environment through a hole in the floor to a safe refuge, Pompl et al. showed a deficit in Tg2576 mice at 7 months of age, but only during the reversal learning phase of the test (75).
Y-maze alternation is another test sensitive to cognitive changes (despite its drawbacks) (71). As for the water maze, Hsiao et al. (4) found that Tg2576 mice were impaired at 9–10 months; Ognibene et al. (76) at 7–12 months, while Arendash and King (73) even found decreased alternation at 3 months. King et al. (69), however, found no difference between transgenics and controls on Y-maze alternation at 3 and 9 months. Another type of Y-maze paradigm is that devised by Dellu et al. (77); if mice are allowed to explore only two arms of a transparent Y-maze, they prefer to visit the novel arm when retested 30 min later. Park et al. (56) found that whereas there was no difference in novel arm recognition between young (3–4 months) wild-type and Tg2576 mice, a deficit had arisen by 12–15 months. In an operant task, performance impairment was seen at 7–8 months (78). Impaired REM sleep was seen in Tg2576 mice by 6 months (21) and accompanied by selective cholinergic neuronal loss in the tegmental area. Impaired contextual fear conditioning was impaired at 5 months (33). This was unlikely due to the increased activity sometimes seen in Tg2576 mice, as conditioning to an acoustic cue was unaffected. Comery et al. (31) also found impaired learning of this paradigm at 5 months of age. Increased impulsivity, which may model secondary aspects of AD, was seen in 12-month-old Tg2576 mice (79).
2.3 Behavioral Work Done in Oxford on the Tg2576 Mouse
In work done in the author’s laboratory at Oxford, both age-related and nonage-related behavioral changes were found in Tg2576 mice (71). Mice were supplied by Boehringer Ingelheim, the offspring of Tg2576 hybrid BL6/SJL male mice crossed with F1B6/SJL females. The Tg2576 mice failed to show a deficit on many of the cognitive tests we performed, even at 12 or 21–23 months of age when they should have been impaired. This is not unusual, as some reports show intact learning and memory even at 15 months (80). In some of our tests, however, even the controls failed to show good learning. These tests have been extensively validated in C57BL/6 mice (64, 81–84). This poor performance might have been due to insufficient backcrossing into C57BL/6 mice. Surprisingly, in spite of the wild-type and Tg2576 performance being very poor on the spontaneous alternation T-maze task, pure SJL mice alternated well.
The three cognitive tests, which showed a notable impairment in mice older than 9 months, were social memory for a juvenile, open field habituation, and a Y-maze escape task motivated by shallow water (the “paddling Y-maze”) (71, 85). The latter was age-related, deficits appearing at 10 months; it is not yet known if the former two are. A reference memory test in a T-maze cued by both position and objects (86) showed a potential age-related deficit, but as only two 23-month-old mice were tested, further work needs to be done to confirm impairment at later ages; at 7–8 months, no difference between Tg2576 mice and controls was seen.
Tests of species-typical behaviors showed that Tg2576 mice had a deficit in burrowing behavior at all ages. Burrowing is a newly developed test in which mice spontaneously empty a tube filled with e.g. food pellets (83, 87). Burrowing deficits were present as early as 3 months of age, before extensive Aβ deposition is present in the brain. Burrowing is known to be an extremely sensitive test; it is affected by a dose of lipopolysaccharide three orders of magnitude below that necessary to cause a change in body temperature (88). An age-independent deficit was also seen in nest construction (89), even as early as 3 months, but only when mice were group-housed; most individually housed mice in either group made reasonable nests. This may have been because an individual mouse needs to make a nest more than group housed mice because it has no cagemates to huddle with. Burrowing and nesting both detect the effects of prion (scrapie) infection at a similar early time in the course of the disease (81). It would be extremely interesting to discover whether there is an age at which burrowing and nesting are not affected, to determine whether the effects at 3 months, when no other behavioral deficits exist, is due to task sensitivity or an innate effect of the genotype, probably unrelated to Aβ.
2.4 The Future
The background strain can markedly influence the behavioral expression of a mutation. It has been shown that hybrid 129/Sv × C57BL/6 mice perform better than either of the parental strains in a water maze (90). An interesting development is the new Tg2576 mouse from Taconic farms (model number 002789) (http://www.taconic.com/wmspage.cfm?parm1=941), which is backcrossed at least 16 times on to one of the behaviorally superior 129 strains (129S6/SvEv). This 129 substrain performs well in the water maze, especially reversal learning (91), although it has a more anxious phenotype than the C57BL/6 mouse (92). It is also wholly pigmented, whereas the mixed B6/SJL genetic background of model 001349 (traditional Tg2576 mice) can produce pink-eyed animals or homozygosity for the Pde6brd1 retinal degeneration mutation.
In conclusion, if I were a clinician, would I prescribe a drug that had been shown to attenuate behavioral deficits in year-old Tg2576 mice? Certainly, not least because there would be few alternatives, but I would consider the chances of a successful outcome to be no more than 50% at best. This mouse has been highly productive in AD research, but there may well be better alternatives.
< div class='tao-gold-member'>
Only gold members can continue reading. Log In or Register a > to continue
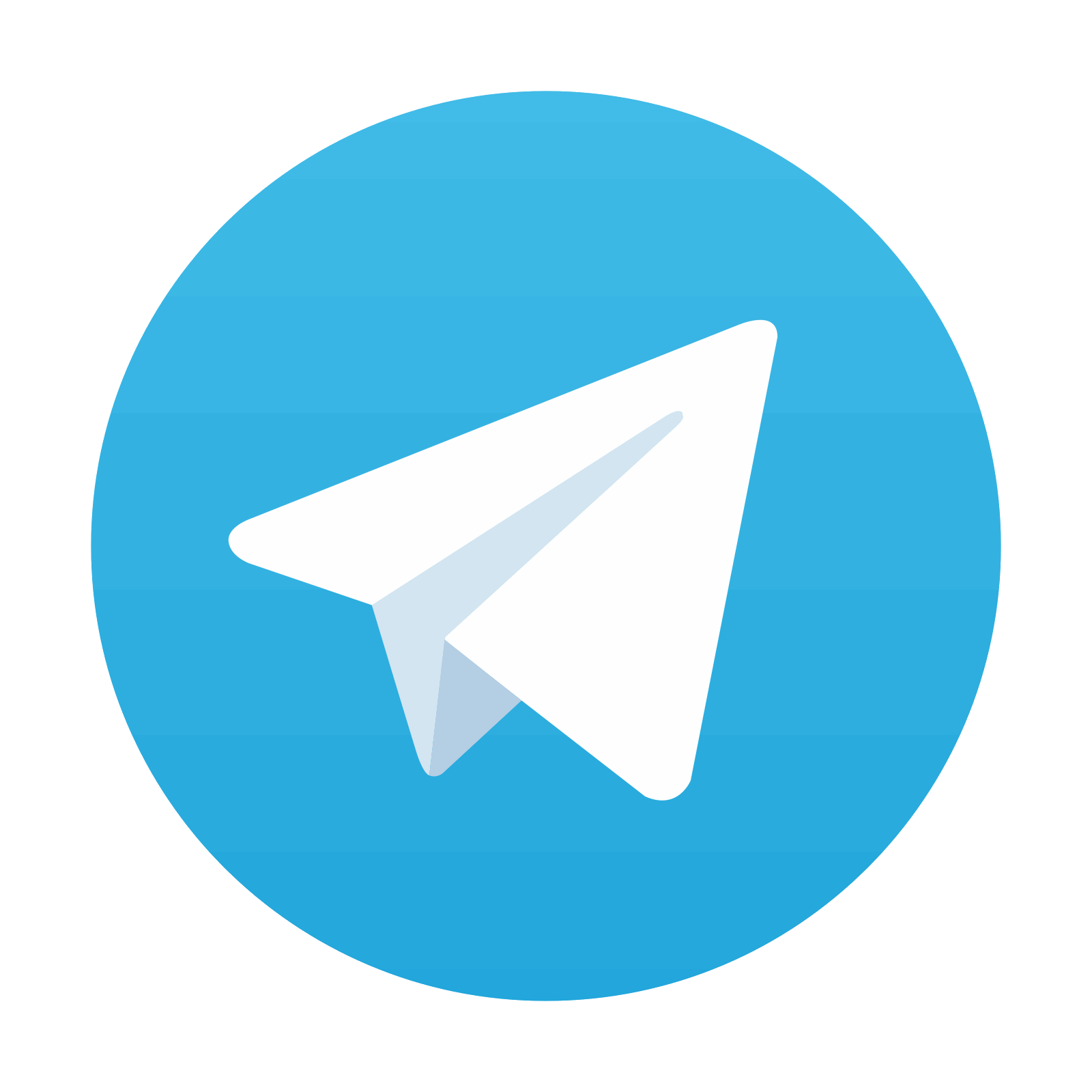
Stay updated, free articles. Join our Telegram channel

Full access? Get Clinical Tree
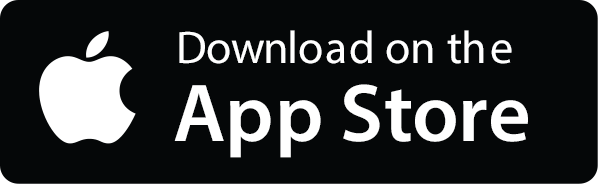
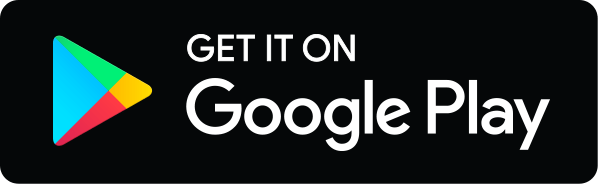