Chapter 5 Reflexes and motor systems
Key points
A reflex is a stereotypical somatic or autonomic activity triggered by a specific stimulus. The reflex arc involves sensory input, connection in the CNS to the UMN, the LMN, neuromuscular junction and muscle.
Muscle tone and bulk depends on LMN function.
UMNs initiate, regulate, modify and terminate the activity of the LMN. UMNs may inhibit or facilitate LMNs. Loss of inhibitory UMN function results in increased muscle tone and spinal reflexes, whereas loss of facilitatory UMNs results in paresis or paralysis.
The UMN system originating from the motor cortex is responsible for voluntary and learned movement of the face, body and limbs using the corticonuclear and corticospinal/pyramidal tracts, respectively. It is more important in primates and humans than quadrupeds.
The extrapyramidal system is responsible for maintaining posture and rhythmical/semiautomatic activities including locomotion. Extrapyramidal UMN tracts originate primarily in the brainstem and their fibres do not travel in the pyramids. In quadrupeds, this system is of primary importance.
Specific UMN tracts are excitatory or inhibitory to LMNs. Movement is ultimately expressed through the LMNs stimulating muscles.
Loss of UMN input typically results in paresis or paralysis with normal to increased muscle tone and spinal reflexes caudal to the lesion. Muscle atrophy is mild and due to disuse.
Loss of the LMNs results in paresis/paralysis, with decreased to absent muscle tone and reflexes. Muscle atrophy can be severe and is neurogenic in origin.
The ‘Neuro RAT’ helps differentiate between UMN and LMN signs – Reflexes, Atrophy and Tone.
Planning of motor activity takes place in the forebrain and motor function is modulated by input from the cerebellum and basal nuclei.
Spinal reflexes
Key points
Reflexes are hard-wired into the nervous system and most are polysynaptic.
Reflexes can be ipsilateral, contralateral, intrasegmental or intersegmental, somatic or autonomic.
Muscle spindles detect stretching of muscle. They provide sensory input to the CNS using 1a afferent fibres and their intrafusal fibres receive motor input from the CNS via γ-efferent fibres. α-LMN innervate the extrafusal muscle fibres.
The myotatic (‘stretch’) reflex has a significant role in maintaining body posture. Gravity causes muscle stretching, stimulating muscle spindles that induce reflex contraction of the muscle.
The Golgi tendon organ inverse myotatic reflex protects the skeletal muscle from excessive contraction by causing reflex muscle relaxation.
Muscle tone is controlled by activity of α and γ-LMN; these LMNs are stimulated by muscle spindles and specific UMN tracts.
That the reflex is the most basic functional unit of the nervous system is evidenced by its presence in simple metazoan animals. In vertebrates, incoming sensory input into the dorsal horn can synapse directly with LMNs in the ventral horn of that spinal cord segment and stimulate a motor output. This simplest reflex is called a monosynaptic reflex and is exemplified by the patellar reflex. Most other reflexes involve interneurons interposed between the input and output side. Interneurons permit divergence (see Fig. 4.9) so that the input can be distributed to a wider population of output neurons. Thus one type of input can stimulate both agonist muscle contraction and antagonist muscle relaxation, e.g. the patellar reflex can stimulate quadriceps muscle contraction and hamstring muscle relaxation (see Fig. 4.4).
There are a number of different types of reflexes including:
(a) Somatic reflexes that permit the animal to respond to the external environment. An example of this is the palpebral reflex, where stimulation around the eyelids causes blinking to protect the eyeball.
(b) Autonomic reflexes that permit the animal to respond to changes in its internal environment. An example of this is the increase in heart rate in response to reduced blood pressure.
Muscle spindles
Muscle spindles are spindle/fusiform-shaped receptors located within striated/skeletal muscles. They are called stretch receptors as they detect stretching of the muscle. Muscle spindles comprise modified muscle cells called intrafusal fibres that have contractile elements at the ends of the spindle and sensory receptors in the middle. The surrounding normal muscle fibres are referred to as extrafusal. Muscle spindles are located in parallel with the extrafusal muscle fibres and, consequently, are stretched when the muscle is stretched. The contractile elements of muscle spindles are innervated by γ-motor neurons that, when stimulated, cause contraction at the ends of the spindle, thus stretching the centre of the spindle. The intrafusal fibres and the γ-motor neuron comprise the fusimotor system. Stretching of the muscle spindle causes firing of the sensory receptors in the spindle and stimulation of the 1a afferent nerve fibres. Sensory impulses travel via the spinal nerve to the spinal cord, or, if arising from head muscles, via cranial nerves to the brainstem. In the CNS the 1a afferent fibre synapses with α-LMNs resulting in stimulation of extrafusal fibres and muscle contraction. The extrafusal fibre plus the α-LMN is the skeletomotor system (Fig. 5.1).
The myotatic reflex
The myotatic (‘stretch’) reflex arc is a feature of limb and trunk muscles especially. It uses the stimulus of muscle stretching to generate nerve impulses in the 1a afferent fibre. The impulses travel to the spinal cord, synapse (usually via interneurons) onto the α-LMN supplying that same muscle, stimulating nerve impulses in that α-LMN. This causes contraction of the extrafusal fibres surrounding the muscle spindle, thereby shortening the muscle and reducing the stretch of the muscle spindle. Thus, activity in the 1a afferent nerve fibre stimulates agonist muscle contraction. Simultaneously, it also causes reciprocal inhibition; that is, it inhibits contraction in the antagonist muscle. For example, the effect of gravity on the weight-bearing stifle joint is to make it collapse (stifle flexion), thereby stretching the quadriceps muscle and its muscle spindles. Spindle stretching causes reflex contraction of the quadriceps muscle and stifle extension to support the animal’s weight. Simultaneously, the stifle flexors (hamstring muscles such as the semimembranosus and semitendinosus muscles) will be inhibited. The input from the 1a afferent is also forwarded to the brain to provide proprioceptive information that is essential for planned and coordinated motor function (see Chapter 6).
(a) The effect of gravity causing the joint to flex and passively stretching the extensor muscles. This causes reflex contraction of α-LMN and is used to sustain posture (see ‘Posture and the myotatic reflex’ in this chapter).
(b) Stretching of tendon and hence the muscle, by tapping of the tendon. For example, the patellar reflex is elicited clinically by tapping the patellar ligament (the continuation of the quadriceps tendon) with a patella hammer, or plexor (see Fig. 4.4 and 13.9).
(c) By activation of γ-LMNs causing intrafusal muscle fibres to contract. This is the mechanism by which many descending UMNs ultimately cause contraction in the muscles – they stimulate the γ-motor neuron, which reflexively causes stimulation of the α-LMNs and amplification of the action.
Within the spinal cord the input from the 1a afferent fibres can link to LMNs in the same spinal cord segment or in different spinal cord segments, ipsi- or contralaterally (see Fig. 4.8). The propriospinal tract is a white matter tract immediately surrounding the grey matter of the spinal cord, and conveys axons connecting between spinal cord segments. Thus input from one muscle can influence other muscles acting around that joint, or other joints in the same limb, or other limbs. For example, in the withdrawal reflex, noxious stimulation of the foot causes limb flexion. If the animal is standing at the time, then the other limbs will extend to compensate for the loss of weight-bearing in the stimulated limb. The withdrawal reflex uses multiple spinal cord segments to activate both multiple flexor muscles within the stimulated limb, and extensor muscles of the other limbs.
Golgi tendon organs and the inverse myotatic reflex
Their sensory innervation is type 1b afferent fibre; this has a higher threshold to stimulation than the muscle spindle. Significant stretching of the tendon stimulates nerve impulses in 1b afferent fibre; these are projected into the spinal cord. This input stimulates interneurons that inhibit the α-LMN of that muscle, thereby decreasing muscle contraction and reducing the tension in the tendon (see Fig. 5.1). It may also stimulate α-LMNs of the antagonist muscle.
α-γ co-activation
When the α-LMNs are stimulated, the extrafusal fibres contract, reducing the stretch of the intrafusal fibres; this decreases activation of the Ia afferent fibre. This decreased sensory input to the CNS would result in loss of proprioceptive input (Chapter 6). But if the γ-LMN is activated simultaneously with the α-LMN, then the intrafusal fibres contract, causing comparable shortening of the muscle spindle. Thus the relative stretch of the muscle spindle is maintained and the Ia firing is sustained. Therefore when a muscle is stimulated to contract, both α- and γ-LMNs are simultaneously stimulated and proprioceptive input from the muscle about its length and tension is maintained. This is called α-γ co-activation. Functionally, this acts to maintain appropriate muscle tone, despite changes in muscle length, and to maintain continual proprioceptive input to brain, which is essential for normal posture and movement.
Posture and the myotatic reflex
The myotatic reflex is reflex activity based on muscle spindle input. It is a major mechanism by which the animal maintains posture and supports itself against gravity. Gravity acts on the animal to cause flexion/collapse of the limb joints, flexion of the cervical vertebral column due to the weight of the head and extension (lordosis) of the thoracolumbar vertebral column due to the weight of the trunk. Thus gravity causes stretching of the muscle spindles in the extensor muscles of the limbs and neck, and flexor muscles of the thoracolumbar vertebral column. The stretching causes firing of the 1a sensory fibres from the spindles, input via the spinal nerves to the spinal cord, stimulation of the α-LMNs and extension of the limbs and neck and flexion of the vertebral column. Simultaneously, the antagonistic muscles (limb and neck flexors and spinal extensors) may be inhibited (Fig. 5.2).
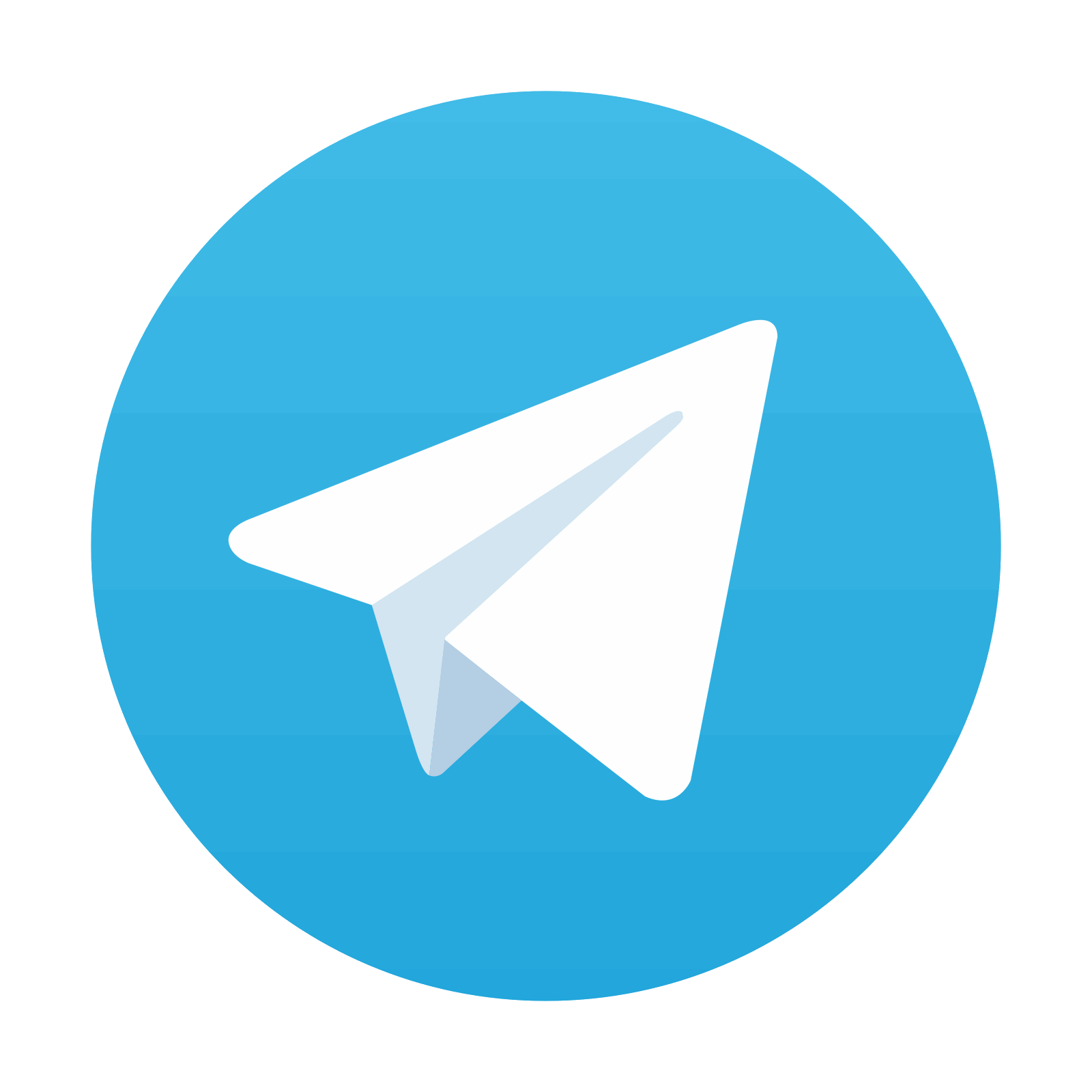
Stay updated, free articles. Join our Telegram channel

Full access? Get Clinical Tree
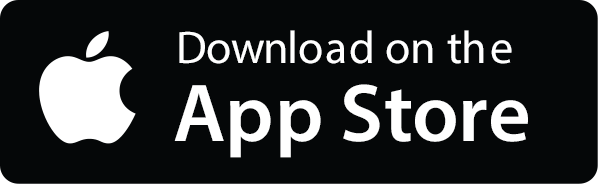
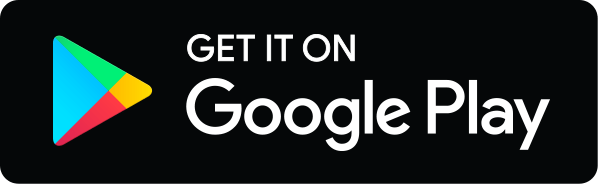