Fig. 1.
Relationship between the development of the CST and the emergence of fine motor control abilities. In rodents, there are no direct connections between CS neurons and the cervical motoneurons which innervate forelimb muscles–brainstem pathways and spinal interneurons relay cortical input to motor neurons. Most of the CST fibres in rodents travel in the dorsal columns. In non-human primates and humans, direct CS connections with motoneurons have evolved, together with an increase in the size and number of the CS fibres. This is reflected in an increase in the size of the excitatory postsynaptic potential (EPSP) elicited by cortical neurons in hand motoneurons. The primate CST is located mostly in the lateral columns, and a significant proportion of CS fibres (∼10%) descend ipsilaterally (but actually many of these cross and still terminate contralaterally; see (44)). Development of the CST correlates with the improvement in the index of dexterity, particularly in the ability to perform finger-thumb precision grip (with permission from Courtine et al. (4)).
There is evidence that these neuroanatomical changes are associated with important behavioural adaptations in the control of the hand. The “index of dexterity” is a measure of the degree of functional adaptation of the hand that allows relatively independent use of the digits and the employment of different types of grasp (5, 6). In the dexterity index, a true precision grip involving pad-to-pad opposition between index finger and thumb is the highest form of dextrous capacity (Figs. 2a and 3a). It is found in humans and in great apes, and is given an index of 7. An opposable thumb, allowing a somewhat more limited form of precision grip is found in dextrous monkeys such as the Old World macaque (Rhesus monkey) and the New World capuchin (Cebus monkey), and has an index of 6. Monkeys with prehensile digits but only capable of a side grip between thumb and index finger (pseudo-opposition) (index 5) include the New World squirrel monkey, while the marmoset, the most widely used non-human primate in modern research, has prehensile digits but a non-opposable thumb, and has a dexterity index of 4.
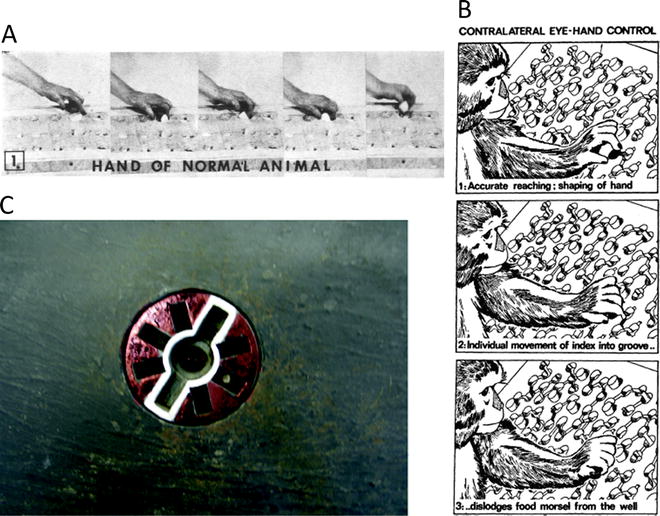
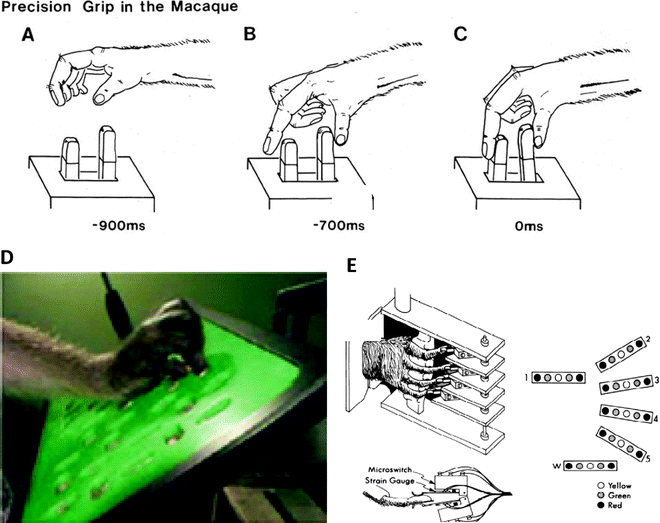
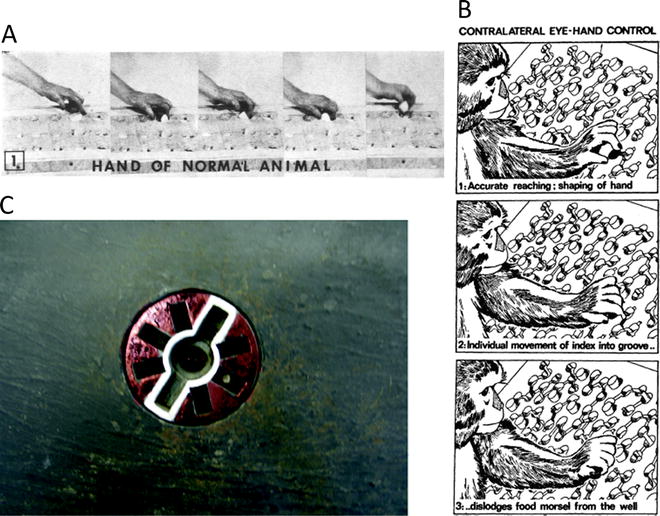
Fig. 2.
(a) Sequence of photographs showing macaque monkey using thumb and index finger to retrieve food from a small well on a Klüver board (with permission from (9)). (b) Brinkman board that requires macaque monkeys to use eye–hand control to retrieve food pellets (black) from the board. Access to pellet is via angled slots in the board. Protruding pegs prevent use of purely tactile cues to locate pellet (with permission from (19)). (c) Rosette device designed to test visuo–motor coordination of precision grip in macaque monkeys. The central food well is accessed by slots on either side, which are highlighted by a white outline. Blind slots located at other orientations do not give access to the reward. The rosette can be rotated on each trial. The monkey has to orient its hands and fingers correctly to gain access to the reward (see (28)).
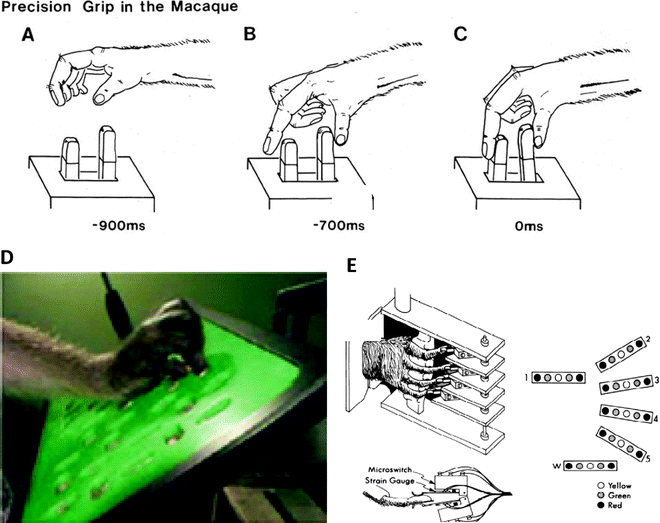
Fig. 3.
(a–c) Sequences showing a macaque performing a trained precision grip movement which involved displacing two spring-loaded levers, one with the index finger and one with the thumb. A plastic cover with two slots above the lever (not shown) restricted access to these two digits and prevented digits 3–5 from contributing; these digits were flexed out of the way. (d) Modified Brinkman board used to assess skilled hand function in monkeys subjected to incomplete spinal lesions and treatment with anti-NoGo antibody (21). Food pellets were placed in wells accessed via slots cut in the board, some horizontal, some vertical. Monkeys used a precision grip (opposition of thumb and index finger) to retrieve food pellets from the board, and monkeys were scored for the number of pellets retrieved in 30 s. (e) Manipulandum designed to test “individuation” of digit movements in the macaque. A pistol-grip device is shown with a monkey’s hand in place, with each digit positioned between two microswitches. An overhead view of a monkey’s finger between two microswitches is shown in detail below. The LED display used to cue different movements (flexion or extension) is shown on the right. 1 = thumb 2–5 = index, middle, ring and little finger, respectively, W = wrist (with permission from (46)).
There is a correlation between the number of direct connections between cortex and motoneurons and the level of manual dexterity of different non-human primate species (Fig. 1; (6–8)). Accordingly, interruption of the cortical projections to the spinal cord in humans and non-human primates causes a major impairment in fine motor function of the hands and feet (9–12) and this is most severe in humans (13). Corticospinal lesions in rats do not affect overground locomotion and induce sensorimotor rather than motor impairments in forelimb function (3, 14), in keeping with the pattern of corticospinal termination in this species.
So we can conclude that if we want to measure the effect of treatments or therapies that might help to recover loss of hand function, a non-human primate model will be needed. The macaque monkey is much more dextrous than the marmoset, and of course its ability to perform dextrous movements, including the use of tools, is supported by an enlarged frontal lobe and a highly developed cognitive system.
3 Biomechanical Considerations
Most of the measures to be described in this article are in some way designed to test and challenge the capacity for non-human primates to perform skilled manipulation of a device or challenging retrieval of food rewards. These functional tests are to some extent all dependent on the capacity to make what Lawrence and Hopkins (15) called “relatively independent finger movements” (RIFM). That is, the capacity to execute “fractionated” digit movements, best exemplified by the control of index finger and thumb.
These authors were careful to use the term “relative”: in fact, even in humans, there are limits to the extent to which the different digits can be moved in a completely independent manner (16). The thumb is the exception to this rule. Studies have shown that there are both biomechanical and neural constraints on independent finger movements (17, 18). The biomechanical constraints arise because of the complex interactions between the many different muscles acting on the digits. Some muscles (such as the extensor digitorum communis and the flexor digitorum profundus, FDP) act on more than one digit. Their motor units are partitioned in such a way that when one digit is moved, it allows associated movements of neighbouring digits. Compared with humans, macaque monkeys have a somewhat restricted repertoire of grasps, partly due to the fact that the monkey has a relatively shorter thumb length compared with finger length (18). Macaques also have more interconnections between the tendons of the FDP and the origins of the lumbrical muscles (18), which increases the mechanical constraints on the independence of individual digits.
Although a small number of muscles actually control the forces exerted at the fingertip (such as FDP), many others are needed to stabilise the articulated bony chain that extends from the shoulder through to the elbow, wrist, hand and phalanges. Only with such stability can accurate tip-to-tip forces be developed. The hand has a very large number of degrees of freedom, and it is very difficult to monitor movements and forces at every single joint, let alone the activity in all the different muscles that underpins this movement.
4 Measuring RIFM
The early studies of Lawrence and Kuypers (9) on the effects of pyramidotomy on fine finger movements in the macaque monkey adapted the original Klüver board (Fig. 2a). This board contains food wells of varying diameter and depth; the smallest wells required precise co-ordination of thumb and index finger to “winkle out” the reward. After section of the pyramidal tract, monkeys were unable to retrieve food, especially from the smallest wells. Very young monkeys, who lack the capacity for RIFM and in whom the CST is immature, also fail on these tests (15).
Monkeys use visual cues to direct their reach to the appropriate well and to orientate their hand and digits to grasp the reward; once contact with the well and reward is made, additional tactile/haptic cues help the monkey to retrieve the reward. Brinkman and Kuypers (19, 20) developed a board which forced monkeys to use visual rather than tactile cues to retrieve food pellets (Fig. 2b). In this board, protruding pegs, of the same diameter and shape as the food pellets made it difficult to use touch alone to find the real pellets; in addition, slots which gave access to the real pellets were cut into the board at a variety of different angles, again forcing the monkey to use vision to guide its approach to the reward. Only monkeys with intact eye–hand control could retrieve food pellets with normal speed and accuracy (Fig. 2b).
Monkeys show hand preference for different tasks, so it may be important to determine this for the particular task that is adopted.
5 Timed Tests of RIFM
A modified Brinkman board was used by Freund et al. (21) to test macaque monkeys with incomplete spinal lesions (Fig. 3d), in which some subjects were treated with the neurite growth inhibitor, anti-Nogo A, to promote recovery. In studies of this kind, there are inevitably large variations in the size and type of lesion, so the methods used have to be very sensitive to detect any consistent changes in control vs. treated animals. The board used by Freund et al. (21) had both vertical and horizontal slots (Fig. 3d). Intact, control monkeys find it more difficult to retrieve pellets from the horizontal slots compared with the vertical slots, probably because they have to adopt a rather unusual hand orientation to do so. Lesioned monkeys often showed much bigger impairments on the horizontal slots (as measured by the number of pellets retrieved in a fixed test time of 30 s) than on the vertical slots. In a further examination of these data, Freund et al. (22) measured the “contact time.” This was defined as the time interval between the first contact of the index finger with the food pellet and the final successful grasp of the pellet utilising pad-to-pad opposition of the index finger and thumb. This contact time is a useful additional measure because it focuses on the dextrous grasp and excludes other components of the retrieval score in the timed period, such as the reaching time and any delay in the monkey eating or chewing the reward before starting a new retrieval.
Nudo et al. (23) used a modified Klüver board to examine the prehensile performance of squirrel monkeys. These authors counted the number of attempts (“flexions per retrieval”) made by the monkey to retrieve the reward (a banana flavoured pellet) from the board. This approach was important in demonstrating the plasticity of the motor system after an experimental cortical lesion, and in demonstrating the power of intensive rehabilitation in promoting plasticity and functional recovery (24). Moore et al. (25) used timed tests on a Klüver-type board to assess changes in dexterity in aged macaques. Owl and squirrel presented with a board of varying well size rapidly adapt a particular two or three digit strategy to optimise rapid food retrieval, and Xerri et al (26) showed that this caused long-term plastic expansion of the representations of these digits in the somatosensory cortex. Timed tests on a Klüver board were used by Belhaj-Saif and Cheney (27) to assess hand function after unilateral pyramidal tract lesions in macaques, and to show the compensatory function of the rubrospinal tract.
6 Visual Guidance of Food Retrieval from the Brinkman Board
In a further development of the Brinkman board, Haaxma and Kuypers (28) designed a “rosette” device that presented the food in a narrow slot, allowing the monkey to insert its index and thumb on either side of the food pellet (Fig. 2c). The slot was highlighted by a white line which cued the monkey to orientate its hand and digits in such a way as to retrieve the reward. Additional “blind” slots were present: these did not give access to the pellet, and were not highlighted. The device could be rotated between trials, so that the highlighted slot was presented at different orientations on every trial. Monkeys with normal eye–hand coordination and able to correctly use the visual cue to appropriately plan its grasp rarely make errors on this task; those rendered apraxic by lesions to the occipito-frontal white matter lesions were at chance as to which orientation they chose, often inserting their digits into the blind slots (28).
Rotating the Klüver board containing the rewards makes additional demands on the monkey’s ability to plan its reach-to-grasp movement precisely so that it is timed to coincide with a moving target. This test was first introduced by Asanuma and Arissian (29) and subsequently used by Kaeser et al. (30), who used a board rotating at 10 revolutions per minutes, and varying the direction of rotation from clockwise to anticlockwise. They used this test to look for a second phase of recovery in fine hand function after monkeys were treated with autologous stem cells derived from the prefrontal cortex.
Examples of both the static and rotating Brinkman board can be found at: http://www.unifr/neuro/rouiller/motorcontcadre.htm.
7 Other Tests of Precision Grip
A potential drawback of the Klüver board is that it does not allow easy visualisation of individual digit movement, nor of the dynamics and kinematics involved. A pioneering study by Hepp-Reymond and Wiesendanger (31) used a small hand-held device that monitored the precision grip forces exerted by the monkey, and this was combined with simultaneous EMG recordings from hand muscles (32). The force required was indicated to the monkey by a visual display. This approach demonstrated a significant deterioration in the level of precision grip force that follows damage to the pyramidal tract, and also showed that the rate at which grip force increased was also much slower, reflecting the slowness and weakness of movement mentioned in the Introduction.
Another approach was used by Muir and Lemon (33): this required the monkey to exert independent control of the index finger and of the thumb to move two spring-loaded levers into a required target zone, and hold them both there for 1 s in order to earn a food reward (Fig. 3a–c; (34, 35)). The displacement of each digit was in the order of a few mm, and forces required were <1.0 N. A cover mounted above the manipulandum levers restricted access to the thumb and index and prevented other digits from contributing, thereby highlighting the fractionation of digit movement and hand muscle activity (36, 37). Using a robotic device it is possible to simulate spring loads with different properties and these can be changed in either a blocked manner or trial-by-trial.
Other investigators have used a small reward mounted in a device that constrains the monkey to use a precision grip to retrieve it. Darian-Smith (38) reviewed the use of high speed digital video to capture macaques grasping a reward held in a device that required the monkey to develop different precision grip force levels to release the reward; these ranged from 0.5 to 2.0 N (see Fig. 4). This approach allows the precise kinematics of the grasp to be documented in great detail. The time taken to collect a glucose tablet held in a spring clip was used by Galea and Darian-Smith (39) to document the maturation of precision grip in neonatal macaques; while such movements are absent at birth, the speed and fluency of the grip develop rapidly over the first 5–6 months of life, and then show a slow but prolonged improvement into the third year. Fine movements of the digits are essential for social grooming, and young macaques are reported to begin grooming at around 5–6 months old (40).
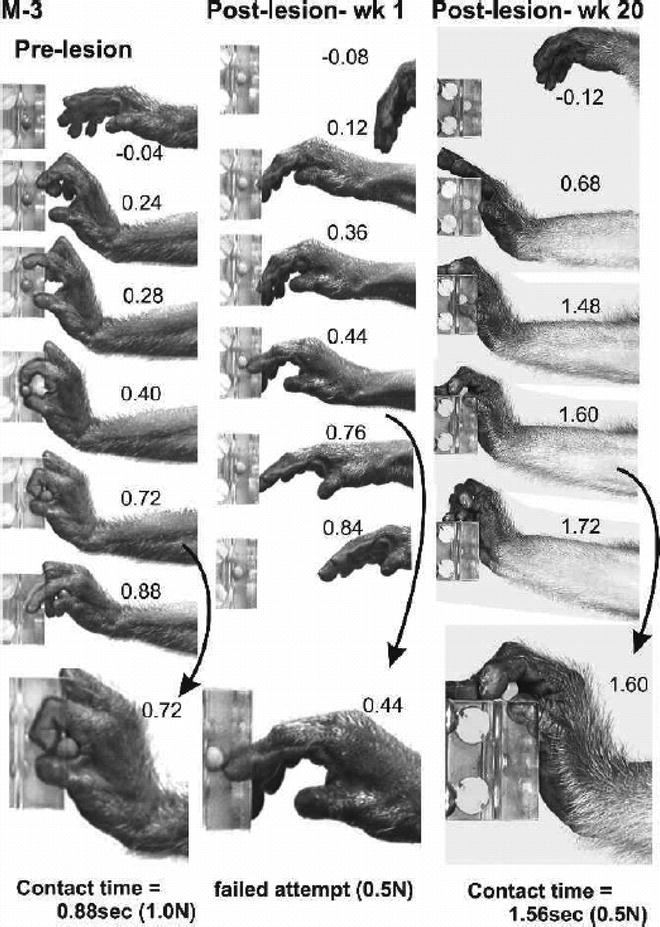
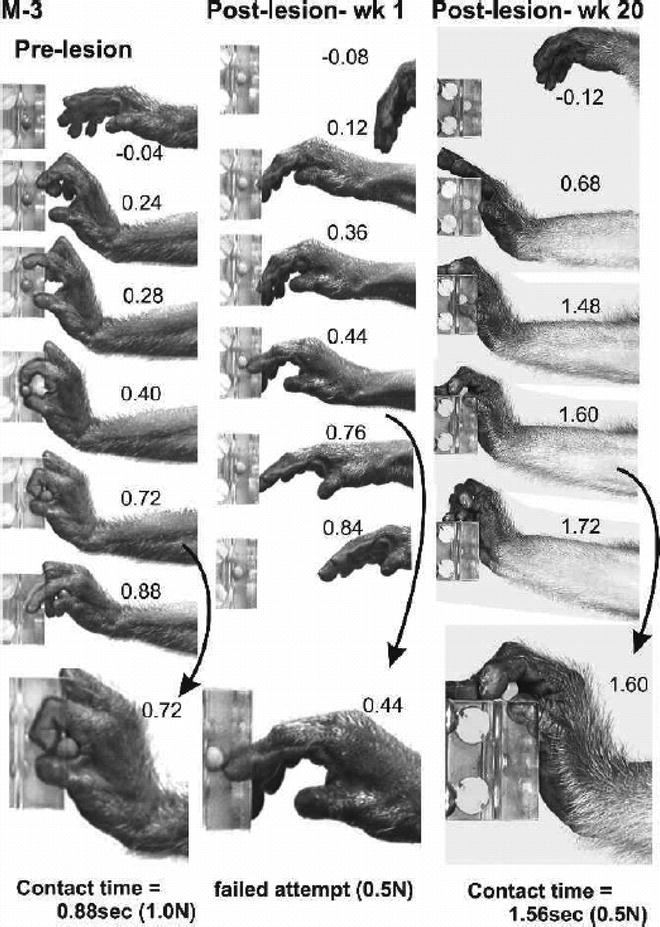
Fig. 4.
Frame sequences from digital video illustrating the recovery of voluntary hand movements and sensorimotor function following a dorsal root lesion that removed all detectable input from digits one to three and the thenar palm. This monkey was unable to perform the reach-retrieval task at all for the first 2 months and then gradually recovered the ability to oppose the digits and to efficiently grasp and remove the target object. Note the failed attempt to retrieve the target 1 week following the lesion and the altered but successful retrieval at 20 postlesion weeks(with permission from (38)).
Digital video was also used by Sasaki et al. (41) to demonstrate that unilateral lesions to the lateral corticospinal tract at the C5 level, which would have interrupted descending corticospinal control of the spinal segment in the cervical enlargement supplying hand and digit muscles, caused an immediate impairment of precision grip. Over the following weeks, the slowness and clumsiness of the grip recovered significantly, and this was again documented by digital video. Recovery may have involved a number of different mechanisms (42), including ipsilateral propriospinal systems and uninjured but slowly conducting corticospinal fibres (43), and possibly a contribution due to collateral sprouting from intact corticospinal fibres descending on the unlesioned side of the cord (44).
< div class='tao-gold-member'>
Only gold members can continue reading. Log In or Register a > to continue
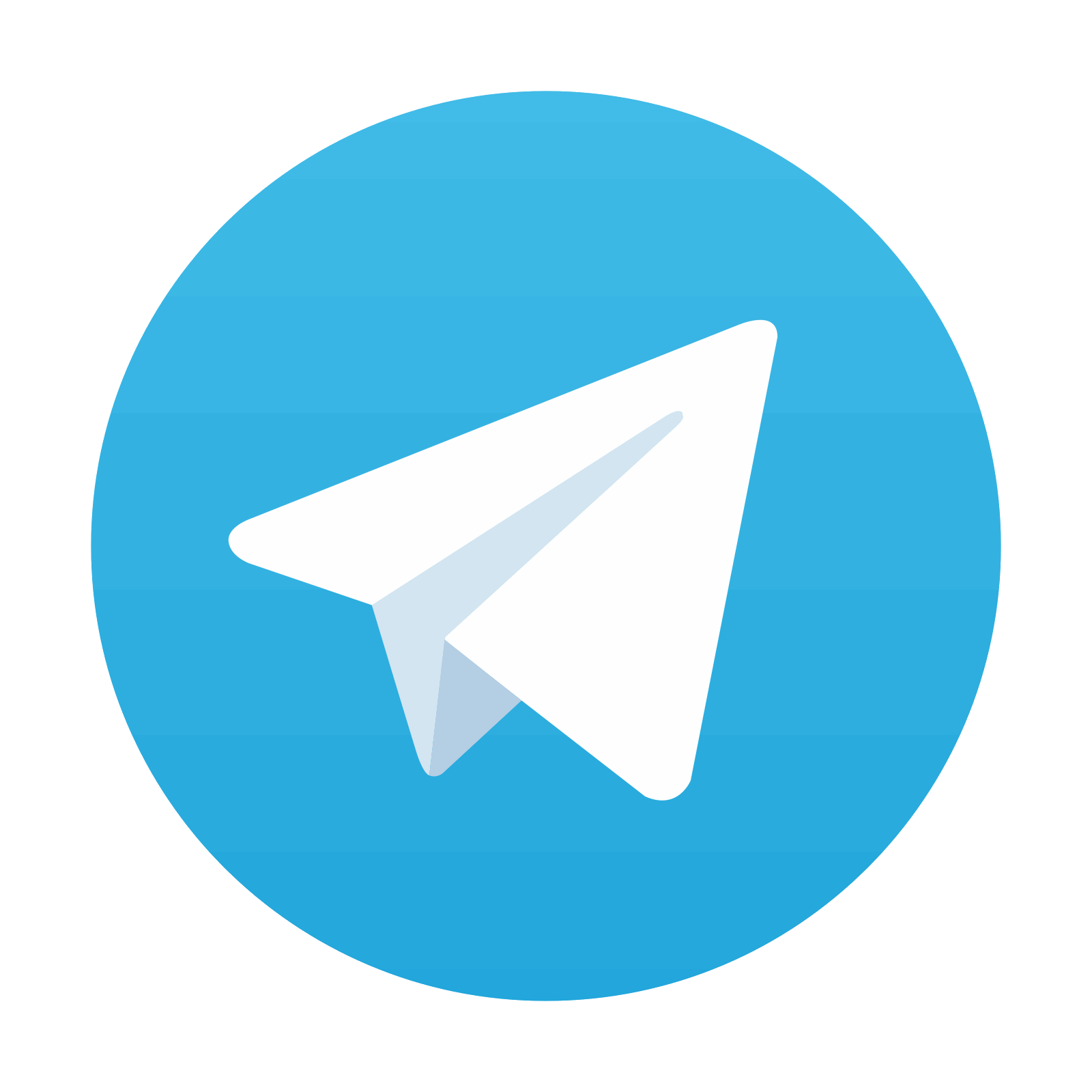
Stay updated, free articles. Join our Telegram channel

Full access? Get Clinical Tree
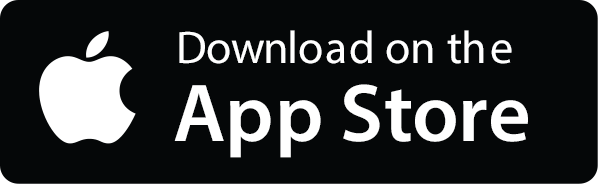
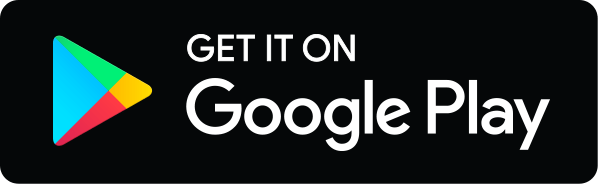