, Peter A. Groblewski1 and Charlene M. Voorhees1
(1)
Department of Behavioral Neuroscience and Portland Alcohol Research Center, Oregon Health & Science University, Portland, OR, USA
Abstract
Place conditioning is a form of stimulus–outcome learning that is commonly used to draw inferences about the rewarding and aversive effects of psychoactive drugs. This chapter focuses primarily on methodological issues that arise in the implementation and interpretation of place-conditioning studies. A description of the basic procedure is followed by a discussion of several key methodological issues, including compartment configuration, apparatus bias, stimulus selection, temporal parameters (interstimulus interval, trial duration, intertrial interval), experimental design and controls, dependent variables, and locomotor activity. Consideration is then given to methodological and interpretative issues that arise when using the place-conditioning procedure to study acquisition versus expression, extinction, and reinstatement of place conditioning. The chapter concludes with a brief discussion of the potential relevance of the place-conditioning procedure for understanding drug seeking and addiction in humans.
Key words
Conditioned place preference (CPP)Conditioned place aversion (CPA)ReinstatementRelapseExtinctionLocomotionStimulus selectionConfigural cuesConditioningDrug rewardDrug aversionConditioned reinforcementDrug seeking behaviorUnbiased procedurePreference test1 Introduction
Place conditioning is a form of stimulus–outcome learning that is commonly used to draw inferences about the motivational effects of psychoactive drugs. It is based on the observation that animals will learn to approach or avoid spatially distinct environmental cues that have previously been associated with rewarding or aversive drug effects, respectively. In its simplest form, place conditioning is implemented as a two-phase procedure. During the first phase, drug administration is paired with exposure to a unique set of environmental cues on one or more occasions. Exposure to a different set of environmental cues is paired with vehicle administration. During the second (test) phase, animals are given the opportunity to choose between the two sets of environmental cues by moving between adjacent spatial locations that contain those cues, typically in the absence of the conditioning drug. Greater approach and contact with the drug-paired cue are interpreted as evidence for a rewarding drug effect, whereas withdrawal and avoidance of the drug-paired cue are seen as evidence for an aversive drug effect. Because animals display a preference or aversion for drug-related cues, the place-conditioning procedure has relevance for understanding the general phenomenon of “drug seeking.” Moreover, examination of the learning and motivational processes that underlie place conditioning might improve our understanding of how those processes are involved in the development, maintenance, and elimination of human drug addiction.
There are two major theoretical explanations for place conditioning. Early investigators (1) adopted a two-process theory framework, viewing drug-paired stimuli as conditioned reinforcers (when using rewarding drugs) or conditioned punishers (when using aversive drugs). It was assumed that drug-paired environmental cues acquired conditioned motivational value through a Pavlovian process during the first phase and that choice of a spatial location during the test phase was secondarily reinforced (or punished) by response-contingent exposure to the previously drug-paired cue during the test phase (2). More recently, some investigators have suggested that behavior during a place-conditioning choice test can also be viewed as an instance of “sign-tracking” behavior (3–5). That is, the drug-paired cue might directly elicit a Pavlovian conditioned approach (or conditioned withdrawal) response that does not depend on an instrumental (i.e., action-outcome) contingency. It is very difficult to distinguish between these alternative interpretations experimentally, and it is reasonable to suppose that both of these types of learning might affect performance during place-conditioning tests (6).
This chapter will focus primarily on methodological issues that should be considered in the implementation and interpretation of place-conditioning studies. Detailed discussion of many of these issues as well as summaries of empirical findings can be found in several previously published descriptions and reviews of the place-conditioning technique (7–18).
2 Basic Procedure
2.1 Habituation or Pretest
Many place-conditioning protocols begin either with a habituation session or with a pretest session. The general purpose of a habituation session is to familiarize animals with nonspecific, possibly stressful aspects of the procedure such as handling, transport to the test room and (vehicle) injection in order to reduce the likelihood that such events will interfere with later learning about the cue–drug relationship. Habituation might also involve exposure to the test apparatus. In some cases, investigators will begin with one or more pretest sessions in which animals have access to the entire apparatus with the stimulus alternatives configured as in the final choice test. The general purpose of such pretests is twofold. First, a pretest familiarizes animals with the test configuration of the apparatus, reducing the possibility that novelty will interfere with the later expression of a conditioned preference or aversion. Second, it provides a basis for assessing each animal’s initial “bias” for the to-be-conditioned stimuli (CSs). Although such pretesting can sometimes be useful, there are reasons for carefully considering how pretests might impact results and their interpretation. One concern is that preexposure to CSs can interfere with later conditioning, a phenomenon known as latent inhibition (9). Another concern is that an animal’s bias for a particular CS might not be apparent on an initial test, or it may change over time (8), raising questions about the value of using pretest scores when assigning the cue to be paired with drug. These problems and the need for pretesting can be avoided by constructing an apparatus that can be shown to be “unbiased” for the target animal population (see later Sect. 3.2).
2.2 Conditioning Trials
During the conditioning phase, animals are given one or more exposures to each of the stimulus alternatives, preferably in a counterbalanced order. One of the stimulus alternatives (labeled the CS+) is consistently paired with drug whereas the other stimulus is consistently paired with vehicle (labeled the CS−). Because relative novelty can influence approach toward a stimulus during choice tests (12), animals should receive an equal number of exposures to each CS during the acquisition phase of the experiment. Consistent with the principles of Pavlovian conditioning, the strength of place conditioning is positively related to the total number of CS+ conditioning trials (19). When conditioning parameters are optimized, learning can be quite rapid. Four to six conditioning trials of each type are usually sufficient to produce reliable place conditioning, but significant conditioning has also been reported with as few as one (20) or two (19) conditioning trials. Temporal and other parameters that influence the strength of place conditioning are discussed in more detail in Sect. 3.
As an alternative to the standard approach for conducting place-conditioning studies, some investigators have recommended the “reference-dose” procedure (21, 22). Whereas the standard approach typically involves a comparison between drug- and vehicle-paired cues, the reference-dose procedure involves a comparison between cues that have both been paired with drug. For example, the two cues might be paired with different doses of the same drug in order to test whether animals can distinguish between their rewarding effects. It has been suggested that this approach might be especially useful for determining dose–effect curves and for revealing dose effects that are difficult to detect using the standard procedure (22, 23). The reference-dose procedure has also been used successfully to test effects of a pharmacological treatment on the acquisition of conditioned place preference (CPP) (24). A recent empirical and theoretical analysis of the reference-dose procedure can be found elsewhere (23).
2.3 Post-conditioning Preference Test(s)
In most place-conditioning studies, the preference test is conducted 24 h or later after the last conditioning trial by offering the animal a choice between compartments that contain the drug-paired and vehicle-paired CSs. The exact configuration of these compartments varies across studies (see Sect. 3.1) and the transition between compartments might involve traversing a “neutral” compartment. Preference tests are most often conducted under drug-free conditions (or after a vehicle injection) in order to avoid interpretative complications created by sensory–motor drug effects. In some cases, however, the purpose of the study might be to examine effects of a specific drug pretreatment manipulation (e.g., injection of a drug antagonist or agonist). Test session durations typically range between 10 and 30 min, though longer durations are sometimes used. Long test durations are typically less useful when working with rats because they tend to become relatively inactive after 20–30 min. Mice, however, remain active over longer periods of time and long-duration test sessions (i.e., 60 min) have been found to be informative in some cases (2, 25).
3 Key Methodological Issues
3.1 Compartment Configuration
Place-conditioning chambers have been constructed in many different configurations using a wide variety of cues. Most often, place conditioning has been successfully reported using apparatuses consisting of one, two, or three compartments. Although these apparatuses differ somewhat in their configuration during training and testing, they all offer a choice between two stimulus environments (i.e., CS+ vs. CS−) during the test. One-compartment apparatuses typically have a set of interchangeable tactile cues (flooring) such as the apparatus described in detail by Cunningham et al. (13). During conditioning, animals are free to explore the entire apparatus with either the drug- or vehicle-paired cues present throughout. During testing, however, each half of the compartment contains a different cue (counterbalanced). Two-compartment apparatuses consist of two equal sized chambers separated by either a wall or guillotine door that is removed or opened during the preference tests. These apparatuses typically contain a combination of visual (e.g., white, black, gray, or striped walls) and tactile (e.g., metal bars, sandpaper, or wood shavings) cues that differentiate the two compartments, but olfactory cues have sometimes been used as well (e.g., almond, lemon). During conditioning, the animal is confined to one of the two chambers, but is allowed to freely explore both chambers during preference testing. Three-compartment apparatuses typically include two conditioning chambers similar to those in a two-compartment apparatus but are separated by a third, usually smaller, “neutral” chamber. This center compartment is not used during the conditioning phase of the experiment, but serves as a starting chamber during the preference test when all three compartments are available.
The advantages and disadvantages of each configuration continue to be the subject of much debate. For instance, one advantage of a one-compartment apparatus is that it reduces the potential confounding effects of novelty on preference expression because the animal has experienced the entire apparatus during both drug and saline conditioning sessions (26). However, although a one-compartment apparatus works well with tactile CSs, it has not been effective for conditioning visual cues that are readily conditioned in a two-compartment apparatus (3). A three-compartment apparatus has the advantage of possibly reducing unintentional bias related to the animal’s initial placement in the apparatus (10), but also introduces the complication of interpreting potential treatment effects on the amount of time spent in the neutral chamber during choice tests. A broader disadvantage of all two-choice apparatuses is that they do not permit the experimenter to differentiate between an animal’s approach to (or preference for) the drug-paired chamber versus withdrawal from (or aversion to) the saline-paired chamber. One solution to this problem is to use a three-choice apparatus in which three distinct compartments are connected via a neutral transition compartment (27). This approach allows the experimenter to include a third, novel chamber during the choice test in addition to drug-paired and vehicle-paired chambers. In an elegant series of experiments using a three-choice apparatus, Parker (27) showed that rats preferred a morphine-, amphetamine-, or apomorphine-paired chamber over a saline-paired or novel chamber, suggesting that the ability of a drug to induce place preference is not due simply to its ability to reduce habituation to (i.e., maintain novelty of) the drug-paired chamber. Rather, choice of the drug-paired compartment appears to reflect the animal’s preference for the conditioned rewarding effects of drug-paired cues. Given these findings, use of a simpler two-choice apparatus is reasonable and sufficient in most cases.
3.2 Apparatus Bias
A place-conditioning apparatus is considered to be “unbiased” when a group of untrained animals, on average, shows no preference for one stimulus alternative compared to another. In contrast, a place-conditioning apparatus is labeled as “biased” when the average response of a group of untrained animals shows a consistent preference (or aversion) for one of the stimulus alternatives. For example, rodents generally tend to prefer a darkened compartment with black walls over a brightly illuminated compartment with white walls (28). Although it is convenient to apply these labels to the apparatus itself, they are more properly described as characteristics of the subject population. That is, the same apparatus might be “unbiased” for one particular mouse strain, but be “biased” for a different mouse strain (29). Therefore, it is essential that apparatus bias be carefully determined for every subject population under study.
Determination of apparatus bias is important because it can impact the ability to detect effects of drug-induced preference or aversion. For example, if a rewarding drug is paired with an already preferred cue, it may be difficult to detect conditioning-related increments in preference due to a ceiling effect (30). Furthermore, use of a biased apparatus raises problems in the interpretation of place-conditioning studies. For instance, if conditioning involves pairing the non-preferred chamber with a putative rewarding drug, it will be unclear whether the response shown during a post-conditioning choice test is due to direct rewarding effects of the drug or is due instead to drug-induced alleviation of whatever motivational processes underlie the unlearned aversion for the non-preferred chamber (i.e., an anti-aversive or anti-anxiety drug effect; see (12, 15, 18)). Using a biased black-versus-white apparatus, for example, drug pairing might increase preference for the less-preferred white compartment because the drug reduces the stress or anxiety normally elicited by exposure to a brightly illuminated place, but not because the drug has a direct rewarding effect. For these and other reasons, use of an unbiased apparatus is strongly recommended, as is use of an unbiased procedure for assigning the specific cue to be paired with drug (i.e., a random, counterbalanced assignment; see (30)).
The impact of apparatus and design bias on the outcome and interpretation of place-conditioning results has recently been discussed in detail by Cunningham et al. (30). These investigators reported that mice showed significant preference for an ethanol-paired tactile cue only when ethanol was paired with the initially non-preferred tactile cue in a biased apparatus. On the other hand, in an unbiased apparatus, mice showed significant conditioned preference, regardless of which tactile cue was paired with ethanol. Overall, these studies suggest that it is especially important to consider each animal’s initial bias when assigning the drug-paired chamber in a biased apparatus (i.e., whether the preferred or non-preferred chamber is paired with drug), but that this issue may be less important when using an unbiased apparatus.
Interestingly, there are cases where use of a biased animal assignment procedure may be necessary for detecting conditioned place preference, even when an apparatus is relatively unbiased. For example, Le Foll and Goldberg (31) concluded, both from an extensive review of the literature as well as from empirical evidence, that a biased procedure in which drug was paired with the animal’s non-preferred chamber was more sensitive for detecting nicotine-induced place preference in rats. No significant preference was detected in rats that received nicotine paired with their initially preferred chamber. The authors noted that one possible interpretation of this finding is that nicotine was able to induce conditioned preference by alleviating stress produced by exposure to the non-preferred chamber.
3.3 Stimulus Selection
Although some apparatuses use CSs from only one sensory modality (e.g., tactile floor cues), most place-conditioning apparatuses use compound CSs composed of several elements from different sensory modalities. For example, visual (e.g., brightness, patterns), olfactory (e.g., scented bedding, wood chips), and tactile cues (e.g., floor textures, metal grids, sandpaper) are often used in various combinations. Some researchers have suggested that tactile floor cues presented in the dark may be more effective than visual or olfactory cues for eliciting approach responses because the animal must actually touch the drug-paired cue in order to elicit a conditioned motivational response (10, 26). In recent studies that compared the efficacy of tactile and visual CSs, we found that tactile cues were able to support development of ethanol-induced conditioned place preference in mice trained in the dark or in the light, using both one- and two-compartment procedures. However, visual cues (presented in the light) were effective only when the cues were presented in a consistent spatial location using a two-compartment procedure (3). Similar assessments of olfactory cues have not yet been reported. Although some investigators have suggested that place conditioning is stronger when multiple-element cues are used (12), there have been no rigorous empirical assessments of this hypothesis.
3.4 Temporal Parameters
Like other forms of Pavlovian learning, place conditioning is sensitive to several important temporal parameters, including: (1) the time delay between exposure to the CS and exposure to the drug (interstimulus interval or ISI), (2) the duration of CS exposure, and (3) the time delay between consecutive conditioning trials (intertrial interval or ITI). Unfortunately, there is no single optimum combination of temporal parameters. Rather, it appears that the optimal parameters vary depending on the nature of the drug, the route of administration, the animal’s species/genotype and static features of the apparatus (e.g., cue modality/intensity, apparatus bias). Thus, investigators must determine optimal parameters empirically for their target population, drug, and apparatus.
3.4.1 Interstimulus Interval
Most place-conditioning procedures attempt to overlap onset of a drug’s central nervous system effects with cue exposure by injecting the drug immediately or several minutes before exposure to the CS+. Injection of drug at a long delay before or after exposure to the CS will not produce conditioning (32). Selection of an appropriate delay interval presumably depends on the specific drug’s pharmacokinetics and may be influenced by route of administration. For example, routes that require longer periods of time for absorption and distribution (oral, intragastric, subcutaneous) may require somewhat longer delays than routes that produce a relatively rapid onset of drug effects (intraperitoneal, intravenous). Also, drugs with relatively slow rates of elimination may be less sensitive to the detrimental effects on conditioning of longer delays between drug and cue exposure than drugs that are rapidly eliminated. The timing and order of exposure to a CS and drug can have profound effects on the direction of place conditioning. In one study, intragastric infusion of ethanol immediately before cue exposure produced conditioned place aversion (CPA) whereas infusion of the same dose 5 min before cue exposure produced conditioned place preference (33). In other studies involving nicotine, ethanol, or amphetamine, injection immediately before CS exposure produced conditioned place preference while injection immediately after CS exposure produced conditioned place aversion (32, 34–36).
3.4.2 CS Duration
Place conditioning has been demonstrated over a wide range of CS (trial) durations, but most often in the range between 10 and 30 min. Some data suggest that the optimal CS duration might depend on both the drug and the animal genotype. For example, in our studies of ethanol-induced place conditioning in DBA/2J mice, a 5-min trial duration produced stronger place preference than 15- or 30-min trials (37). However, when the same strain and apparatus were used with cocaine, place conditioning was more robust with a relatively long, 60-min trial duration than with 15- or 30-min trials. In contrast, a different mouse strain (C57BL/6J) showed similar cocaine-induced place preference at all three trial durations (38). Thus, one cannot assume that the optimal trial duration determined for a particular drug and apparatus will necessarily be optimal when using a different drug, genotype, or species.
3.4.3 Intertrial Interval
In contrast to Pavlovian conditioning procedures that involve short-lived unconditioned stimuli like food pellets or electric shock, ITIs in drug conditioning studies are typically measured in hours or days rather than seconds or minutes. In most place-conditioning studies, CS+ (drug) and CS− (vehicle) trials are typically separated by intervals of 24 h or more, providing more than enough time for effects of most drugs to dissipate between trials. This strategy also allows both types of trial to be conducted at the same time of day, eliminating a possible influence of circadian variables. In some studies, however, investigators administer both types of trial on the same day, raising the possibility that the conditioning of one cue is influenced by its temporal proximity to the trial that precedes or follows it. For example, if the first trial of the day involves injection of a drug with a long half-life, residual drug effects may affect learning on the second trial of the day. Alternatively, if the first trial involves a drug with a relatively short half-life, learning on the second trial might be influenced by acute withdrawal (“hangover”) from those drug effects, which could condition an aversion to the second trial cues. Some investigators have attempted to avoid such problems by always making the CS+ (drug) trial the second trial of the day. However, because trial order is not counterbalanced, this approach confounds drug effects with time-of-day (circadian) effects. Moreover, if the interval between the CS− and CS+ trials is very short, learning on the CS− trial might be affected by drug exposure on the following CS+ trial (38). The best way to avoid all of these potential problems is to separate the drug and vehicle trials by 24 h or more.
3.5 Experimental Design and Controls
The expression of a conditioned place preference or aversion is assumed to depend on the formation of a learned association between contextual stimuli and the drug’s rewarding or aversive effects. Because there are many reasons why exposure to a drug might alter preference for a contextual stimulus, it is important to include proper controls in the experimental design to isolate effects that reflect associative cue–drug learning (39). One common approach is to compare an experimental group (i.e., a group that receives cue–drug pairings) to a vehicle-only control group that receives equivalent exposure to the CSs, but always receives vehicle injections instead of drug. Although a vehicle-only group is useful for detecting unlearned biases or shifts in biases that might occur due to repeated cue exposure or the passage of time, it does not control for any non-associative effects produced by drug exposure. For example, a drug that had no direct rewarding or aversive effects might nevertheless produce changes in cue preference due to chronic changes in sensory–motor function or motivational tone. Thus, a better control is provided by making comparisons between groups that are matched for overall exposure to both the CS and drug (39, 40).
One such strategy involves use of an “unpaired drug” control group. This group receives exposure to the CSs without drug (i.e., with vehicle), but also receives exposure to the drug unpaired with the CSs. For example, drug exposure might occur in the home cage several hours after each exposure to the cue that is normally paired with drug in experimental animals. Conditioning is indexed by the difference between the paired experimental group and the unpaired control group. One problem with an unpaired drug group, however, is that it does not control for possible conditioning of responses to features of the apparatus or test room that are common to both the drug- and vehicle-paired compartments. For example, in contrast to an experimental group that receives drug in the apparatus on half of its trials (i.e., on CS+ trials), an unpaired group is unlikely to acquire a conditioned activity responses to general cues of the apparatus (41), thus providing an alternative interpretation of test differences between paired and unpaired groups. An arguably better control strategy involves using a counterbalanced discrimination control procedure in which one cue is consistently paired with drug (CS+) whereas the other cue is paired with vehicle (CS−) in all animals. The specific CS paired with the drug is counterbalanced across groups, and successful conditioning is indexed by analyzing the difference between the counterbalanced conditioning subgroups. Because these groups are matched for exposure to the apparatus, CSs, and drug, but differ in the paired relation between each cue and drug, group differences are assumed to reflect the underlying cue–drug association (13, 30, 39).
3.6 Dependent Variables
Investigators have used several different dependent variables to index performance during place-conditioning test sessions. The most common measures are: (a) raw time scores (e.g., number of seconds spent in compartment A), (b) percentage time spent in the drug-paired compartment, (c) difference between time spent in the drug-paired compartment and time spent in the vehicle-paired compartment, or (d) the difference between time or percentage time spent in the drug-paired compartment during the post-conditioning test and time or percentage time in that compartment during a pretest. Two critical issues in the selection of a dependent variable are: (a) whether the apparatus and subject assignment procedure are biased or unbiased and (b) the nature of the experimental design and the specific comparisons that are used to draw conclusions about the development of a conditioned place preference or aversion. For example, experiments that use a biased subject assignment procedure will typically include a pretest as part of the procedure and then index place conditioning by calculating a post-conditioning minus pretest difference score. Although such changes might be informative, it is important to note that within-subject preferences might change between a pretest and posttest for reasons that are unrelated to drug-induced conditioning. In cases where a pretest is not required (e.g., when using an unbiased apparatus and unbiased subject assignment procedure), however, the dependent variable can be based entirely on behavior measured during the post-conditioning test. As noted earlier, the most informative comparisons will be between groups that have been matched for their overall exposure to the choice stimuli and drug (e.g., between paired vs. unpaired groups or between the counterbalanced subgroups in a discrimination design, that is, A+, B− vs. A−, B+). When the apparatus is unbiased and an unbiased subject assignment procedure has been used, we recommend a comparison of raw time scores between the counterbalanced subgroups as the most direct way to provide evidence of successful place conditioning. Cunningham et al. (30) have discussed the rationales for all of these dependent variables as well as their advantages and disadvantages. They also provided an empirical comparison of all four measures in experiments involving either a biased or unbiased apparatus.
3.7 Locomotor Activity
Processes unrelated to drug-induced conditioning can disrupt the expression of conditioned-place preference or aversion. The simplest way of disrupting or altering expression is by introducing a manipulation or experimental variable that alters locomotor activity during the preference test session. For example, the design of a place-conditioning study might include administration of a pharmacological agonist or antagonist before the choice test in order to assess whether the treatment drug alters the motivational processes underlying expression of conditioned-place preference or aversion. While such tests might be informative, their interpretation becomes complicated if the drug elicits a significant increase in activity, a behavior that could compete with the behavior of maintaining contact or proximity to the drug-paired cue. Subject variables that are linked to differences in basal activity (e.g., genotype, sex) might also confound interpretation of differences in expression of place conditioning. A recent study highlighted the importance of these concerns by showing an inverse relationship between the strength of conditioned-place preference and the level of test activity induced by a pretest injection of an activating dose of ethanol (42). This relationship was most apparent in the DBA/2J mouse strain, which is strongly activated by ethanol. In fact, the high levels of activity in these mice after ethanol completely interfered with the expression of place preference. The inverse relationship between preference and test activity was also observed in another mouse strain (NBZ/B1NJ) that is much less activated by ethanol. In that case, however, the ethanol-induced increase in activity was not sufficient to interfere with the expression of preference. Overall, these data underscore the importance of measuring locomotor activity during place conditioning tests and considering that information in the interpretation of results.
< div class='tao-gold-member'>
Only gold members can continue reading. Log In or Register a > to continue
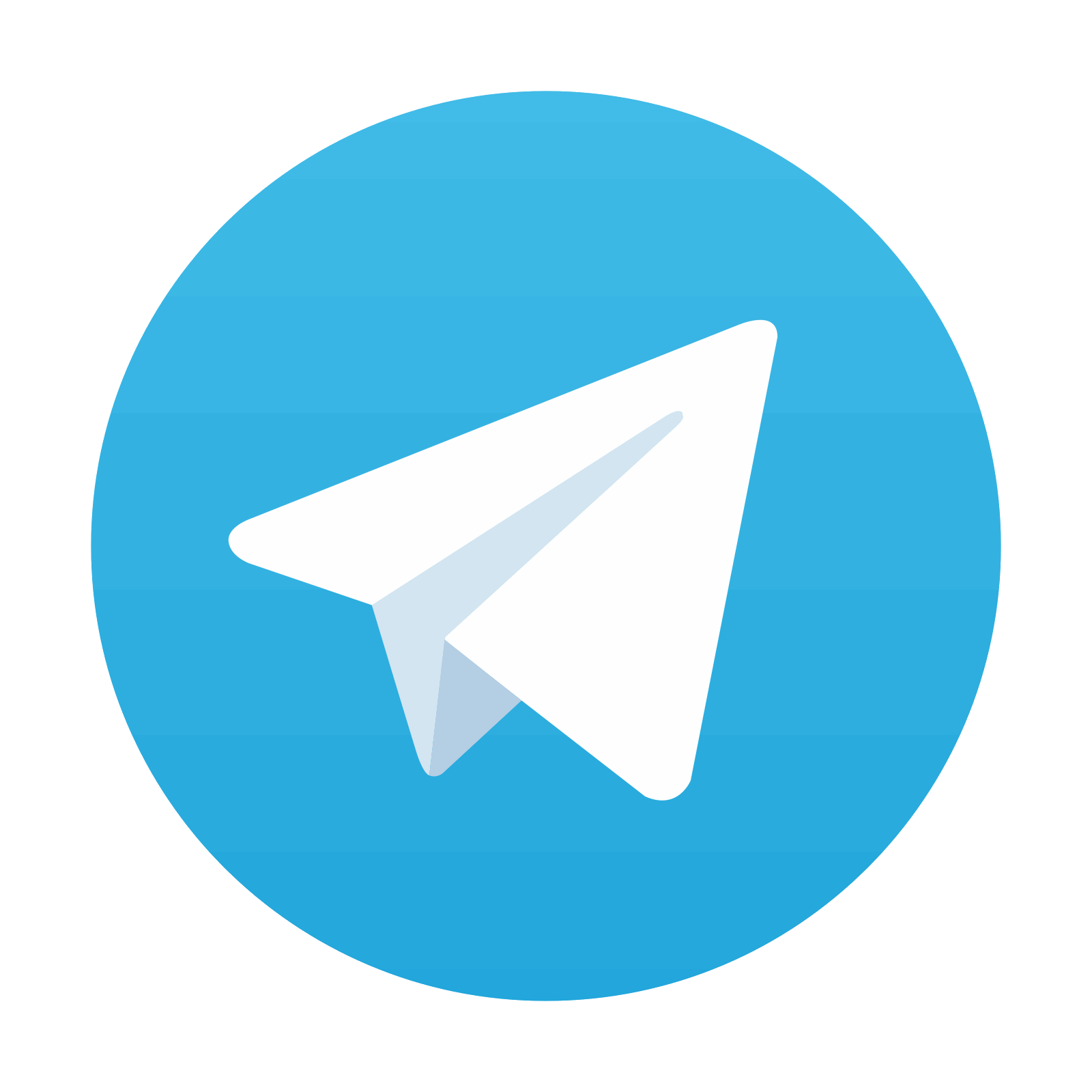
Stay updated, free articles. Join our Telegram channel

Full access? Get Clinical Tree
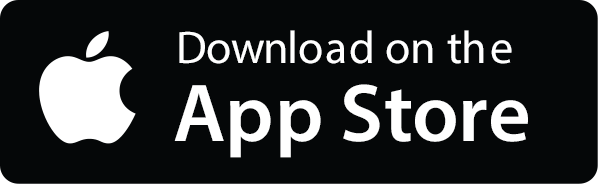
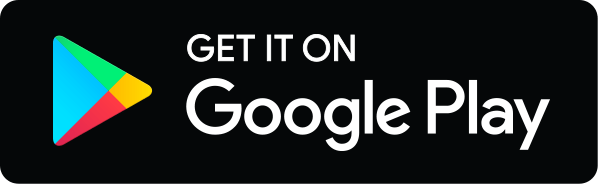