and Richard A. Meisch2
(1)
Department of Psychiatry, MMC 392, University of Minnesota, Minneapolis, MN, USA
(2)
Department of Psychiatry and Behavioral Sciences, University of Texas Health Science Center at Houston, Houston, TX, USA
Abstract
This review provides an overview of animal models of acquisition of drug reinforcement by discussing research findings from studies that used drugs such as cocaine, methamphetamine (METH), phencyclidine, and nicotine, as well as several routes of drug self-administration (SA). Theoretical perspectives are given for the acquisition models indicating that the animal models are valid predictors of human behavior. Common organismic factors that contribute to the acquisition of drug abuse are also discussed, such as sex, hormonal influences, innate preference for sweet substances, impulsivity of choice, impaired inhibition, and avidity for physical activity. Differential rates of acquisition of drug SA in rats selectively bred for high and low sweet intake, ethanol intake, or avoidance responding are also discussed. Environmental factors such as enriched versus impoverished conditions, and the effect of behavioral economic factors related to drug abuse (e.g., effort, cost/reinforcement) are also considered. Pharmacological factors have also been found to influence acquisition, such as prenatal exposure to drugs, and potential treatment drug can reduce the rates of acquisition in animal models. Interrelations among factors are described, and their implications are summarized. This review adds to previous accounts of acquisition by shifting the emphasis from analysis of the process of acquisition of drug-taking to an assessment of the major factors that are influential in the initiation and acceleration of this process. The goal is to present translatable findings from animal research that are useful for informing prevention of drug abuse in humans.
Key words
Acquisition/initiationDrug self-administrationReinforcing effectsAnimal modelsRoute of administrationBiological determinantsEnvironmental determinantsPharmacological influences1 Introduction
Acquisition of drug self-administration (SA) is defined as initial use of a drug, a transition from early sporadic sampling to an increase over a period of hours, days, or weeks to a steady rate of intake over time. The use of animal models to represent this phase of drug abuse in humans has many advantages, such as the ability to study (1) behavioral and biological processes underlying acquisition of drug SA; (2) factors that control acquisition, either alone or in combination, over the lifespan of an animal, and (3) behavioral and pharmacological approaches to preventing or reversing acquisition. These studies may then provide information regarding the initiation of human drug abuse on factors regulating the initial occurrence of drug addiction, the underlying neurobiology, and how drug addiction can be prevented. This prospective approach is in contrast to the longitudinal epidemiological studies that can be done in humans and to the retrospective reports that can be analyzed over a longer addiction cycle in humans. The methods used to accomplish these objectives are described below.
The focus of this review will be on newer developments in the last decade since acquisition of drug SA was last reviewed (e.g., (1–3)). The main acquisition procedures that have been used, with the oral, pulmonary, and intravenous (i.v.) routes, as well as parenteral and intracranial methods will be discussed in terms of traditional and updated methods and the findings that have been generated. One major finding is that there has been less emphasis on methodology and more emphasis on application of these models to better understand the increasing prevalence of drug abuse. Thus, the majority of this review will emphasize current findings on the main factors influencing acquisition, such as biological/genetic, environmental, developmental, and pharmacological.
2 Acquisition Procedures
The routes of drug intake most commonly studied in the preclinical laboratory are those most commonly used by humans, i.v. and oral; however, other routes have been used, and they are close approximations of the routes of administration that are used in human drug abusers. Drug reinforcement in laboratory animals was first convincingly demonstrated with the i.v. route (4). However, the successful use of other routes extends the generality of drug-reinforced behavior and permits examination of the role of route of administration. That drugs can be established as reinforcers by these multiple routes extends the parallel with human behavior. Moreover, the successful establishment of many drugs as reinforcers by multiple routes also broadens the domain of drugs as reinforcers and the conditions under which drug reinforcement can be studied.
2.1 Intravenous (i.v.)
A study of intracranial electrical stimulation demonstrated that delays of even 1 s markedly impair acquisition of brain self-stimulation (5). Nevertheless, it takes approximately 10 s for intravenously delivered cocaine to affect the brain (6). How can acquisition of intravenous cocaine reinforcement develop and be effective under such conditions of delay? The answer probably lies in the initial peripheral stimulation that immediately follows cocaine infusion (6). This interpretation is supported by the use of a peripherally, but not centrally, acting cocaine derivative, cocaine methiodide. In rats with experience with cocaine SA, but not in naïve rats, the intraperitoneal (i.p.) administration of cocaine methiodide resulted in ventral tegmental glutamate release and reinstatement of responding. These results are important for they suggest a mechanism for bridging the delay between onset of drug infusion and onset of CNS effects, namely rapid onset of peripheral drug effects that come to function as conditioned reinforcers. The methods used to establish i.v. SA attempt to capture this process.
2.1.1 Procedures to Facilitate i.v. Acquisition
Drug SA studies have increased dramatically in the last 10 years, and a high proportion of them have used the intravenous route. In the majority of these studies, rapid acquisition was used as a means to subsequently study other phases of drug abuse, such as escalation or reinstatement, and two methods were commonly used. First, experimenter-administered priming injections of the SA drug are given at the start of the sessions to stimulate exploratory behavior on the response manipulandum. A second approach is to train a response for food reward, and then substitute drug. Another form of drug substitution has been used occasionally, whereby a new drug of interest replaces one that is strongly maintaining SA. The substituted drug is given for several days, and doses are varied. The original drug may be reinstated between doses of the new drug. A disadvantage of this method is that extinction can take 2 weeks or more; thus it is not clear whether the behavior is being reinforced by the substituted drug or is the result of extinction from the first drug.
2.1.2 Autoshaping
An autoshaping method has been used to establish i.v. drug SA, as it allows for a gradual and controllable acquisition process that can be standardized across animals, and it permits a quantitative analysis of the process (7). During autoshaping, 10 drug infusions are delivered under a random-interval (90 s) schedule during each 1-h interval for 6 h. For each of the 10 noncontingent infusions, a retractable lever is extended, the lights over the lever are illuminated, and an infusion is delivered after 15 s, and then the lever retracts. If there is a response on the lever before the 15 s elapses, the lever immediately retracts and an infusion is delivered. During a subsequent 6-h SA component, the lever remains extended, and infusions are available contingent upon a fixed-ratio 1 (FR 1) schedule. Autoshaping produces a gradual, negatively accelerating function for the percent of animals in the group that meet the acquisition criteria (8). It is sensitive to many manipulations (e.g., dose, schedule, feeding condition, sex, hormonal differences, treatment medications). Measures of acquisition are percent of group and number of days to meet acquisition criteria, which was defined as a mean of 100 infusions per 6-h session for 5 days for a 0.2 mg/kg dose, or 50 infusions/day for 0.4 mg/kg. The use of autoshaping as an animal model of the acquisition of drug SA has face validity because initial exposure to drugs and associated cues in humans is often without response cost. For example, in humans, the initial exposure to drugs may be from a dealer requiring little or no cost, and then the price is increased with frequency of subsequent use. Both the establishment of stimulus-reinforcer associations and initial low response cost are important in the establishment of the reinforcing effects of drugs.
2.1.3 Growth Curve Analysis
Growth curve modeling has been used to capture the process of acquisition and to add more quantitative indices than those provided by the time to acquire and number in the group meeting criteria (9, 10). The growth curve, modeled after human growth curves, describes individual patterns of increasing drug intake over time with a quadratic equation that yields several measures: y-intercept, slope, and an acceleration parameter. While the model is a promising approach and offers several dependent measures, it was not sensitive to differences in dose or FR requirement. (11). Use of the model with i.v. nicotine SA described acquisition as an initial rapid acceleration in drug consumption, with an increase that waned over time (9, 10). Although the Donny et al. (9, 10) method has not been applied to other drugs or the oral route, descriptions of individual acquisition patterns from studies in other laboratories using oral (12) or i.v. (8) SA demonstrate similar acquisition functions.
2.2 Oral Methods
In humans, the oral route (and absorption in the stomach) is used with ethanol drinking and with the nonmedical use of prescription drugs; oral ingestion of nicotine products (e.g., chewing tobacco, gum) is through the mucosal lining of the mouth. Drugs such as phencyclidine (PCP), cocaine, marijuana, and others can also be consumed orally. In studies with animals, there are two impediments to establishing orally delivered drugs as reinforcers. One is the delay between ingestion and the onset of CNS effects, and the other is the aversive taste of most drug solutions when they are within the range of concentrations that can be reinforcing. Procedures have been developed to deal with these problems (for detailed reviews see (3, 13)).
With the i.v. route, the immediate onset of peripheral drug effects mediates the delay between the infusion and the onset of CNS effects. However, with the oral route, the delay between drug delivery and CNS actions is substantially longer. Therefore, how is it possible to establish orally delivered drugs as reinforcers? Exteroceptive stimuli that accompany drug SA (e.g., physical properties of the drug and drug seeking and taking stimuli associated with the environment) serve as conditioned cues that maintain the behavior. Taste of drug preparations can come to serve as a conditioned reinforcer, and this has been evident in animal studies. For example, with pentobarbital, the range of unit drug doses that maintain oral drug SA is below the range of i.v. doses (see Table 1). The oral dose is calculated as the amount of drug delivered (mg) upon schedule completion divided by the monkey’s body weight. The amount of drug is the volume delivered (e.g., 0.5 mL) multiplied by the drug concentration (e.g., 1 mg/mL). These low drug doses suggest that it must be the taste of the drug solutions that mediates the delay between drinking and the initiation of CNS effects. A related finding is that a quinine solution, when substituted for PCP, maintained responding for 7 to over 30 sessions before values fell to control levels (21). Thus, the quinine solution was serving as a conditioned reinforcer. Support for the role of taste comes from studies of taste aversion conditioning that have demonstrated learning can occur when there are long intervals between ingestion of a substance and subsequent effects.
Table 1
Comparison of oral (p.o.) and intravenous (i.v.) pentobarbital doses
Pentobarbital doses (p.o.) | |||
---|---|---|---|
Lightest monkey 7.2 kg | Heaviest monkey 11.8 kg | ||
Pentobarital (mg/mL) | Dose (mg/kg) | Pentobarbital (mg/mL) | Dose (mg/kg) |
0.125 | 0.009 | 0.125 | 0.005 |
0.25 | 0.017 | 0.25 | 0.011 |
0.5 | 0.035 | 0.5 | 0.021 |
1 | 0.069 | 1 | 0.042 |
2 | 0.139 | 2 | 0.085 |
4 | 0.278 | 4 | 0.169 |
References | Pentobarbital doses (i.v.) | ||
(14) | Dose: 3 mg/kg | ||
(15) | Doses: 0.25, 0.5, 1, and 2 mg/kg | ||
(16) | Doses: 0.1 to 3 mg/kg | ||
(17) | Doses: 0.25, 0.5, 1, 2, and 4 mg/kg | ||
(18) | Dose: 4 mg/kg | ||
(19) | Dose: 5 mg/kg | ||
(20) | Dose: 5 mg/kg |
2.2.1 Induction of Drinking with Food
If animals do not drink a drug solution, acquisition of drug-reinforced behavior will not occur. The problem is that often drug solutions have an unpleasant taste; thus, sufficient drinking will not take place to allow the CNS reinforcing effects to occur. One solution is to induce drinking. Several methods have been used. One is to employ schedule-induced drinking, which results in excessive water intake when animals intermittently receive small pellets of food (22). Low drug concentrations are substituted for water, and over time the drug concentrations are gradually increased (23, 24). Once significant drug effects occur, such as changes in the animal’s overt behavior, the pellet deliveries can be discontinued (25). If the drug has been established as a reinforcer, drinking persists at levels that exceed vehicle (usually water) levels (25, 26). By having an initial session component free of inducing conditions, one can monitor the development of reinforcing effects within individual animals across sessions (27). Following the initial component, the inducing conditions can be introduced to insure that drug consumption occurs. A related method to induce drinking is to give the animal food during the session. Drinking of water and drug solutions (postprandial) reliably occurs following eating (12, 28, 29).
2.2.2 Fading: Induction of Drinking with Liquid
A third induction method is to use a liquid such as a low ethanol concentration that is readily consumed, probably due to a positive taste (30–32). Fading procedures are used to introduce the new drug and then remove the original liquid. A low concentration of the drug to be established as a reinforcer is added to the liquid solution (usually 2% ethanol), and across sessions the drug concentration is gradually increased. Subsequently, the ethanol concentration is reduced in steps to zero. If consumption of the drug solution persists above vehicle levels, then the drug has been established as a reinforcer. These induction methods have been successfully used with mice, rats, and rhesus monkeys. An additional strategy is to use animals that have high baseline levels of water intake. Low drug concentrations can be substituted for water and subsequently be increased across sessions (29, 31, 33, 34).
2.2.3 Drug Substitution
Once one drug has been established as an orally effective reinforcer for rhesus monkeys, other drugs can be substituted for the first drug and responding will be maintained (21). Usually, the drugs are from the same pharmacological class, such as PCP like (21, 35), barbiturates (36), and benzodiazepines (37). However, this is not always the case, for d-amphetamine was successfully substituted for PCP (35). The ability to establish orally delivered drugs as reinforcers by simple substitution for another drug expedites research and is theoretically interesting.
2.3 Intragastric (i.g.)
Rats and rhesus monkeys will self-administer a variety of drugs via the i.g. route, which is a variation of the oral route that bypasses taste and orosensory factors (38). However, it has been difficult to establish and maintain drug reinforced behavior using the i.g. route (see (39)). The reasons are probably due both to the delayed onset of drug effects relative to some other routes and to the delayed onset of peripheral cues. For example, with the i.g. route, there are no immediate taste cues such as those that occur with the oral route, and no rapid onset of drug effects found with the i.v. route. Acquisition is much slower than with the i.v. route, and the delayed onset of drug effects increases the possibility of overdose (39). Thus, with the i.g. route, large drug doses are necessary to sustain drug taking, and responding is not maintained under high-value intermittent schedules (39). These findings illustrate the importance of the immediacy of onset of either conditioned or unconditioned drug effects.
2.4 Smoking
A laboratory model of cocaine-base smoking is another oral procedure that was developed around 1990, and this laboratory method has been used to conduct parallel studies with rhesus monkeys (40) and humans (41). Monkeys were first trained to self-administer PCP and water, as described in the oral methods. They were then trained to make sucking responses on a hollow steel tube with a replacable, coiled nichrome wire that fits into the inside of the tube. The drug was dissolved in 95% alcohol, placed on the coiled wire with a syringe, then alcohol was allowed to evaporate. Before each smoking trial, a drug-coated coil was placed into the smoking tube and connected with the circuitry on the outside of the cage. After the response requirement (five sucking responses) on the smoking spout is completed, the coil was heated, the cocaine was volatilized, and the monkey inhaled the drug. A similar apparatus (and verbal instructions) was used with humans, and results indicated that nearly all of the drug was inhaled and absorbed by both monkeys and humans, and similar physiological measures (heart rate, blood pressure, drug level in plasma), and time courses resulted (41).
The smoking procedure has continued to provide valuable information over the last 18 to 20 years. Studies have shown that nondrug incentives and medications reduce cocaine smoking, and when both treatment approaches were combined, cocaine smoking nearly stopped (42). Other drugs such as heroin, heroin–cocaine (speedball) combinations, and THC have been studied, and extensive behavioral economic analyses of smoking-rewarded behavior have been conducted regarding the effect of sex and availability of nondrug reinforcers on the acquisition of smoking cocaine (43). The smoking model is labor intensive, but the results are highly consistent with human behavior, and tests of treatment attempts are predictive of clinical results (see (41)).
2.5 Intramuscular, Intraperitoneal, and Subcutaneous
In addition to the i.v., oral, and inhalation routes, acquisition of drug-reinforced behavior can occur with other routes such as intramuscular (i.m.), i.p., and subcutaneous (s.c.) (see Table 2). For example, lever pressing by rhesus monkeys can be maintained by response-contingent i.m. injections of morphine or cocaine (44). The injection is administered by the experimenter upon completion of the schedule requirement. The advantages of this procedure are the lack of need for surgery, catheters, and infusion pumps. In addition, the i.m. route can be used over long periods of time. The disadvantages are the need for the experimenter to give the injection when the schedule is completed and the training required to establish i.m.-injected drugs as reinforcers.
Table 2
Additional routes of drug self-administration
Route | Species | Reference |
---|---|---|
Intramuscular (i.m.) | Rhesus monkeys | (44) |
Squirrel monkeys | (45) | |
Intragastric (i.g.) | Rats | (46) |
Rhesus monkeys | (20) | |
Intracerebroventricular | Rats | |
Intracranial | Rats | |
Rhesus monkeys | (51) | |
Mice | (52) | |
Intrathecal | Rats | (53) |
Intraperitoneal (i.p.) | Rats | (54) |
Subcutaneous (s.c.) | Rats |
A similar procedure has been developed for rats (54, 56). Initially the rats were trained to respond under a fixed-interval (FI) schedule with food as the reinforcer. Next, a fading procedure was used. After food was obtained, the rat was given either an i.p. or s.c. injection of the potent opioid, etonitazene. The dose was gradually increased across sessions, and subsequently the amount of food was systematically decreased. Responding persisted in the absence of food, and the substitution of saline for drug resulted in a drop in response rate below drug values. Reintroduction of the drug produced a return to the previous levels of responding. Thus, the drug clearly functioned as a reinforcer. The advantages and disadvantages are the same as with the i.m. route. With all three routes, all behavior leading up to the initial injection is reinforced by the drug without the behavior being influenced by direct drug effects. These experimental methods make possible the study of drug-reinforced behavior over long time periods and in laboratories that lack the equipment and facilities for intravenous studies.
3 Factors Affecting Acquisition
A common goal of drug addiction research is to identify factors that contribute to the acquisition of a habitual drug-abuse pattern in humans, so that at-risk individuals can be targeted for prevention or treatment attempts that are focused on their specific vulnerabilities. Phenotypic variations in acquisition of drug SA are strongly influenced by individual differences in genotype, physiological state, drug history, interactions with the environment, as well as the pharmacological and neurobiological aspects of the drugs and the SA process. In the following section, these factors are divided into biological, environmental, and pharmacological/neurobiological determinants, and each is briefly discussed; however, these factors do not influence acquisition of drug SA independently, and many interactions exist among them (see Table 3; (57)).
Table 3
Interaction of risk factors for drug abuse
Risk factors | Adolescents > adults | |||||
---|---|---|---|---|---|---|
Sex/hormonal | + | Sex/hormonal | ||||
F > M | ||||||
Impulsivity | + | + | Impulsivity | |||
HiI > LoI | ||||||
Sweet liking | + | + | + | Sweet liking | ||
HiS > LoS | ||||||
Novelty reactivity | + | + | O | + | Novelty reactivity | |
HR > LR | ||||||
Exercise | + | + | − | − | O | Exercise |
HiR > LoR | ||||||
Environment | + | + | − | ? | + | ? |
Poor > rich | ||||||
Stress reactivity | + | + | + | − | + | ? |
High > low |
3.1 Biological Factors
3.1.1 Sex/Hormonal
Research with animal models over the last decade has shown that female rodents and nonhuman primates acquire drug SA faster, in greater numbers per group, and at lower doses than males (58). This finding has consistently been reported with different routes of administration, several species, and with a wide range of drugs including amphetamine, caffeine, a cannabinoid receptor-1 agonist (59), cocaine, ethanol, fentanyl, heroin, methamphetamine (METH), morphine, nicotine, and phencyclidine (see reviews by (59, 60)). Most acquisition studies were conducted with rats; however, female monkeys exceeded males on acquisition measures (58). The sex differences (females > males) in acquisition also extend to other critical transition phases of drug abuse such as escalation, binge use, dysregulation, compulsive intake (61, 62), and reinstatement of behavior previously reinforced by cocaine (63). Also, chronic exposure to a cannabinoid agonist (CP 55,940) during adolescence increased acquisition of cocaine SA in females but not males (64). Females also respond better than males to behavioral and pharmacological treatment for drug SA (58); however, males exceed females in withdrawal effects (e.g., (65–67)).
The underlying basis for the most consistent sex differences (females > males) in various aspects of drug abuse is the ovarian hormones. Specifically, estrogen increases and progesterone decreases the rewarding effects of drugs. Studies with ovariectomized (OVX) estrogen-replaced animals indicate that drug SA occurs more rapidly and in greater numbers of rats than vehicle-treated OVX controls or sham-operated rats treated with the estradiol antagonist, tamoxifen. The facilitating effects of estrogen on acquisition have been shown with cocaine (60) and heroin (68). In contrast, progesterone counteracts the facilitating effects of estrogen in female rats, and male rats are not affected by these ovarian hormones (60). Estrogen and progesterone have similar opposite effects during other phases of the addiction process (69–71). Results of the animal studies are in close agreement with reports in humans regarding positive subjective effects of drugs when estrogen peaks (follicular phase) and negative effects when estrogen is opposed by high levels of progesterone (72). Thus, sex and hormones associated with the phase of the menstrual cycle may be important determinants in the initiation of drug abuse in humans.
3.1.2 Age
Animal models are essential for studying the influence of age on the acquisition of drug abuse, as it is difficult to experimentally study human drug abuse at the early age at which it begins. Animal studies have focused on comparisons of adolescent versus adult onset of drug SA to parallel human conditions (57). Two basic questions are most commonly addressed: (1) does drug exposure during adolescence alter acquisition of SA of the same or different drugs in adults, and (2) does the rate of acquisition of drug SA differ in adolescent versus adult animals? Rodents offer an ideal model for the acquisition phase because the length of their adolescence ∼ 30 days allows adequate time to initiate drug SA and to conduct subsequent tests during adulthood. For example, exposure to ethanol during adolescence resulted in greater acquisition of ethanol SA in adults (73). Work with rats also showed that nicotine exposure during adolescence (compare with exposure as adults) increased the acquisition of cocaine SA when both groups were tested as adults (74).
In studies that compared adolescents and adults during acquisition of drug taking, rats exposed as adolescents (vs adults) exhibited faster acquisition of cocaine SA and in greater numbers per group (75, 76). Adolescent rats also acquired SA of nicotine (77) and ethanol (71) faster than adults. Adolescent rats also showed greater intake of saccharin (vs water) than adults (74, 78). These results are consistent with greater sweet preference in younger versus older humans (79), and it is related to drug reinforcement because sweet preference predicts acquisition of drug SA (80). Overall, adolescent drug (and other reward) exposure increases the likelihood and rate of acquisition of drug use as adults.
3.1.3 Sweet Preference
Rats selected for their avidity for sweet tastes rapidly acquire SA of drugs such as amphetamine, ethanol, and morphine compared to animals with lower sweet preferences (see (79–80)). In rats that were selectively bred for a high (HiS) and low (LoS) saccharin intake, HiS rats exceeded LoS rats in free-choice and forced-choice ethanol drinking using a two-bottle choice (81), and in acquisition of i.v. cocaine and heroin SA (see (79–80)). Rats that self-administered cocaine had saccharin preference scores proportional to the speed of acquisition.
Recent studies indicate that HiS rats are more impulsive than LoS rats, and impulsivity is another behavioral factor that predicts drug SA. For example, HiS rats exceeded LoS rats in the acquisition of i.v. cocaine (0.8 mg/kg) SA under a Go/No-go procedure (69). Drug preference also correlates with sweet intake, as rats that were selectively bred for high or low ethanol intake showed corresponding high or low sweet intake, respectively (82). Thus, saccharin intake is a strong predictor of the acquisition of ethanol and cocaine SA, as well as other phases of drug seeking and taking such as escalation, extinction, and reinstatement (80).
3.1.4 Impulsivity
Impulsive behavior is another major predictor of acquisition of drug abuse (see reviews by (83, 84)). There are several operational definitions of impulsivity, including choosing a smaller-immediate reward over a larger-delayed reward, inability to stop a behavior that has no or negative consequences, and impatience, short attention span, or engaging in risky behaviors. These aspects of behavior and their relationship to drug abuse have been modeled in animals and humans, and mainly two measures of impulsivity have been studied: impulsive choice – choice of a smaller immediate over a larger delayed reward (83–85), and impaired inhibition – inability to withhold a prepotent response (86). Impulsive choice is modeled in animal and human preclinical studies using a delay discounting procedure. These forms of impulsivity will be discussed with respect to their influence on acquisition of drug SA.
Delay discounting. Initial studies in rats using a delay discounting procedure (see (84)) have used this task to identify high (HiI) and low (LoI) impulsive animals that were subsequently evaluated for their rate of acquisition of drug SA. In one of the first studies (87), rats chose between two immediate pellets or 12 delayed (15 s) pellets in a Y-maze. When their subsequent consumption of 12% ethanol was measured, the HiI rats that selected the immediate pellets on at least 75% of trials consumed more ethanol than those that were less impulsive. A second study extended this task to operant conditioning measures of delay discounting for one immediate versus three delayed food pellets and examined subsequent i.v. cocaine SA in HiI and LoI rats (88). Rats selected as HiI showed more rapid acquisition of cocaine SA, and at greater numbers than that of LoI rats. Subsequent work with the HiI and LoI rats indicated that impulsivity was similar in males and females (∼70%, and ∼24% for HiI and LoI respectively) (63). Also, high impulsivity predicted escalation and reinstatement of cocaine-seeking behavior, but LoI rats exceeded HiI rats during extinction.
Impaired inhibition. The Go/No-go (89), stop signal reaction time (SSRT) (90), and 5-choice serial reaction time (5CSRT) tasks (91) have been used to measure impaired inhibition (see (84)). For example, rats selected as HiI based on the 5CSRT task made more nose-poke responses to initiate i.v. nicotine SA than LoI rats (92). In another study using this task, rats screened as HiI subsequently self-administered larger amounts of i.v. cocaine than LoI rats, and HiI rats (vs LoI) also had fewer D2 dopamine receptors in the ventral striatum (neurocircuitry related to reward, novelty response, and movement) (93). Interestingly, both this study using a 5CSRT task and the Perry et al. (88) study using the delay-discounting method demonstrated that impulsivity measures were predictive of cocaine SA, but they were not correlated with locomotor activity in a novel environment that has also been shown to predict acquisition of drug SA (94).
3.1.5 Novelty Reactivity
In humans, there is a correlation between drug abuse and behaviors such as sensation seeking, novelty seeking, and risk taking (95). Similarly in rats showing a propensity to explore a novel environment, such as rats that are high responders (HR) in a novel environment, showed greater drug-induced locomotor activity, and more readily acquired drug SA than low responders (LR) in the novel environment (96–98). For example, HR rats, compared with LR rats, showed faster acquisition of amphetamine (94, 99), cocaine (100), and nicotine (101) SA, particularly when exposure to a novel environment was forced (e.g., (101)) and not a choice (e.g., (99)). Also, rats selectively bred for HR acquired cocaine SA more rapidly than LR-bred animals (102). In these studies, exposure to the novel environment was forced. In contrast, when free-choice novelty procedures were used (96), there were negative findings on the relationship between HR versus LR rats and acquisition of SA.
3.1.6 Physical Exercise
There is also evidence that a proclivity for physical activity predicts drug abuse, and this “exercise preference” is independent of novelty-related locomotor activity (103). For instance, wheel running is a nondrug, noningestive positive reinforcer for rats, as they lever press to gain access to a wheel (104). Rats escalate their revolutions when given unlimited (105) or long (103) access, and wheel running is chosen over food under some conditions (106). Thus, wheel running has several features in common with drug addiction. In a study using female rats, the animals were allowed access to a running wheel for 6 h daily for about 3 weeks until behavior stabilized, then groups were selected for high (HiR) and low (LoR) running based on a median split of revolutions. By the time the groups met the acquisition criteria, the HiR rats had significantly more cocaine infusions than the LoR rats, and that pattern continued for 14 days of maintenance. There were no differences in extinction between groups, but the HiR group exceeded the LoR group in reinstatement responses on the previously active lever after an i.p. cocaine-priming injection (103).
Animals in this study were initially tested for locomotor behavior in an open field apparatus for novelty reactivity (Day 1) and locomotor activity (Day 2). Neither measure revealed group differences correlated with wheel running. In another study, rats that were experienced with chronic exercise showed a greater conditioned place preference (CPP) for cocaine than a group exposed to sedentary conditions before the CPP procedure (107). Thus, individuals that experience more exercise, either because they are genetically predisposed or they have more opportunities in their environment, show greater sensitivity to the rewarding (SA) and conditioned-rewarding effects (CPP) of cocaine then those inclined toward or exposed to less exercise. Overall, avidity for wheel running (HiR) was a predictor of drug seeking and taking, and this was similar to other high-responding phenotypes such as HiS, HiI, and HR.
3.1.7 Roman Selectively Bred High and Low Avoidance Rats
Roman high- (RHA) and low-avoidance (RLA) rats have been selectively bred respectively for rapid or poor acquisition of a two-way active avoidance in the shuttle box apparatus, initially in Rome and later in Sardinia, Italy (see (108)). When the RHA and RLA rats were compared on acquisition, maintenance, extinction, and reinstatement of cocaine-seeking behavior (108), over 4 weeks of training at doses of 0.1, 0.2, 0.4, and 0.4 mg/kg, respectively, the RHA line showed significantly more infusions than the RLA line in weeks 3 and 4. The RHA line also showed higher extinction responding (resistance to extinction), and cocaine-induced reinstatement responding than the RLA line. This is another example of the effect of genetic differences in selected rat lines on their initial vulnerability to drug SA. Other genetically divergent lines show differences in drug SA (e.g., Lewis > Fischer 344), but acquisition studies are lacking.
3.1.8 Ethanol Intake
In other genetic studies, rats were bred to prefer (AA) or not prefer (ANA) alcohol, and the drug-naive AA rats showed greater intake of cocaine and etonitazene, a potent agonist at the mu opioid receptor, than ANA or outbred Wistar rats (109). The AA rats also acquired i.v. heroin SA more rapidly than the ANA rats, and when i.v. ethanol was substituted for heroin, the AA rats self-administered more than the ANA rats (110). With other lines, selective breeding for ethanol intake has been shown to result in rapid acquisition of ethanol SA (111) as well as acquisition of behavior maintained by other drugs (82) and nondrug substances (112). For example, Le et al. (82) examined the acquisition of i.v. nicotine and oral (10%) sucrose SA in alcohol-preferring (P) and nonpreferring (NP) rats. The P rats’ intake exceeded NP rats’, and there was a vertical upward shift in the dose (nicotine) – and concentration (sucrose) – response functions. There were also changes during extinction and reinstatement in which the P line exceeded the NP line. On the other hand, P versus NP line differences were not observed in cocaine acquisition or maintenance; however, higher doses were used that do not always reveal individual differences during acquisition (8). Rats bred for high and low ethanol intake also showed respective high and low intake of sweet substances (112) indicating that selective breeding may have pleiotropic effects. Genetic influences similar to those shown in animals are also clearly represented in humans (113). The findings suggest that there is a genetic correlation between ethanol preference and acquisition of not only ethanol but other drug and sucrose intake in drug-naïve rats.
3.1.9 Neurobiology of Acquisition
Possible neurobiological mechanisms for the acquisition of drug SA have been discussed extensively (e.g., (114, 115)). There have been few studies investigating the neurobiological basis of the acquisition process, possibly because it is difficult to study behavior that is confounded with the direct effects of the drugs that are self-administered.
One approach would be to examine the role of different neurotransmitters involved with acquisition of SA of specific drugs. For example, evidence suggests a critical role for dopamine in the reinforcing effects of cocaine. Genetic knockout studies would yield useful information, but they are done in mice, and SA models mainly exist in rats and primates, as i.v. SA is difficult to accomplish in mice. Nevertheless, in studies with mice dopamine receptor 1 subtype (D1) knockout mice did not reliably acquire SA of cocaine (and other D1 and D2 agonists) (116), while D2 knockout mice were no different than wild-type controls (117). Thus, D1 receptors are necessary for establishment of the reinforcing effects of cocaine and other dopamine-related drugs. Another option would be to examine neurobiological differences in rats selected or bred to be addiction-prone or -resistant, such as Lewis and Fischer 344, AA and ANA, P and NP, HiS and LoS, HiI, and LoI using drug-naïve representatives of these populations.
< div class='tao-gold-member'>
Only gold members can continue reading. Log In or Register a > to continue
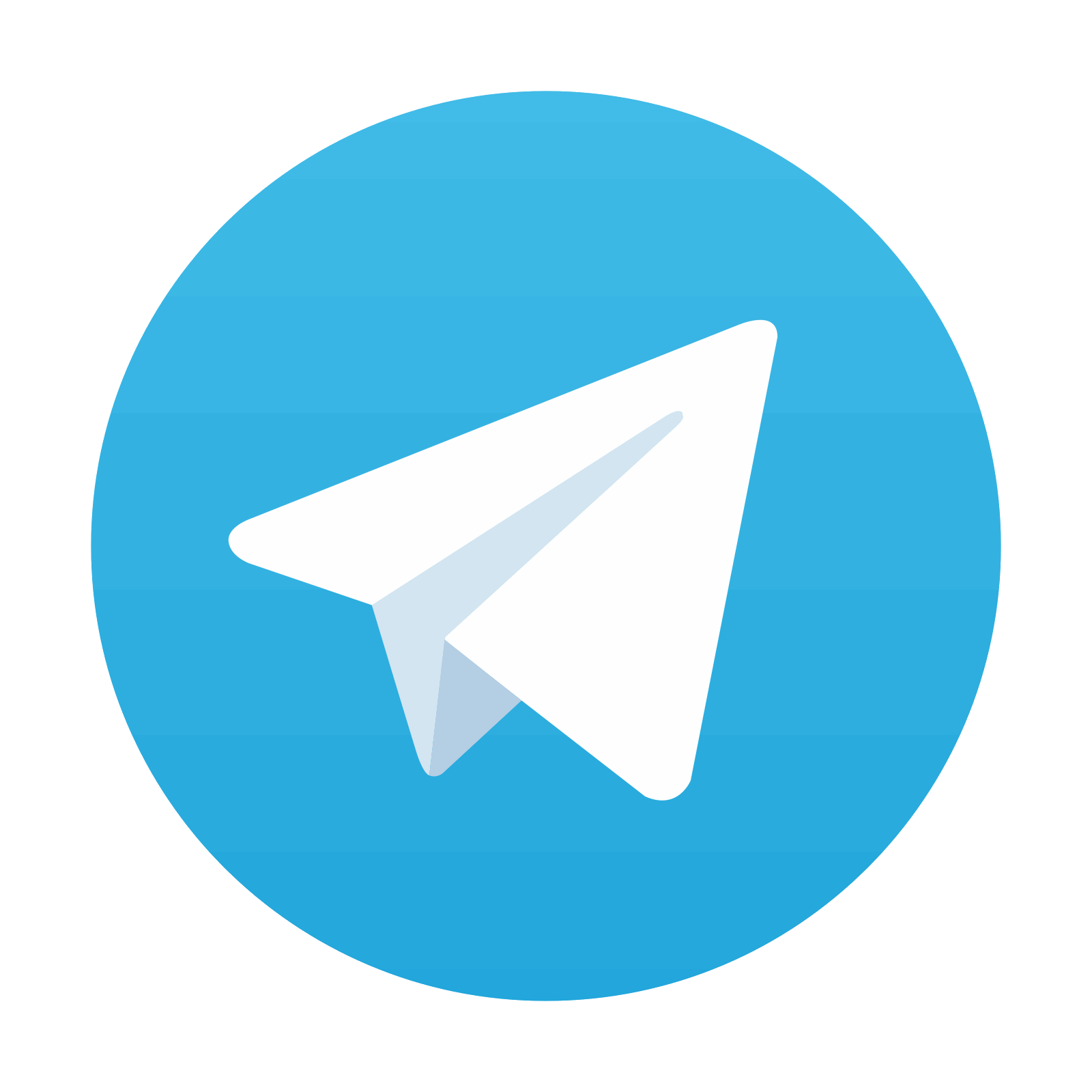
Stay updated, free articles. Join our Telegram channel

Full access? Get Clinical Tree
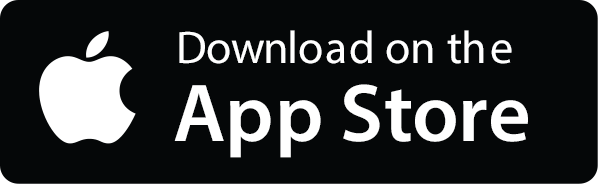
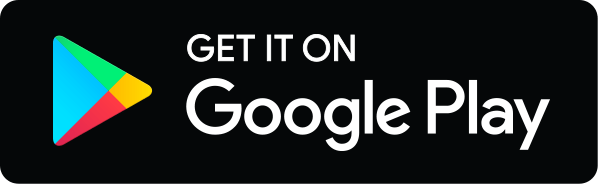