Chapter 97 The pancreas is made up of the right and left lobes, which join to form a small, central body (Figure 97-1, A). The right lobe is closely associated with the proximal duodenum and contained within the mesoduodenum. It is the most readily accessible portion of the pancreas and is easily exposed by retraction of the descending duodenum. The left lobe of the pancreas begins at the pylorus, extends along the greater curvature of the stomach, and is contained within the dorsal leaf of the greater omentum. Exposure of the left lobe is achieved by retraction of the omentum and stomach ventrally (Figure 97-1, B). The body of the pancreas is closely associated with the proximal duodenum. The pancreas consists of lobules of glandular tissue surrounded by fine connective tissue. Connective tissue septa joining the lobules contain blood vessels, ducts, nerves, and lymphatics. The pancreas has exocrine and endocrine components (Figure 97-2), with 98% total pancreatic mass formed by the exocrine portion and associated vessels, nerves, and ducts. Pancreatic lobules are composed of acinar cells, which are responsible for synthesizing digestive enzymes, and cells that make up the duct system within that lobule. The endocrine pancreas is composed of islands of polygonal cells, known as islets of Langerhans, that form anastomosing cords nestled in the pancreatic lobules and intimately associated with acinar cells. Within the islets are four distinct polypeptide-secreting islet cell types: α-cells that produce glucagon, β-cells that produce insulin, δ-cells that produce somatostatin, and F or PP cells that produce pancreatic polypeptide. The majority of the pancreatic blood supply originates from the celiac artery and is supplied via the splenic and hepatic arteries (Figure 97-3). The splenic artery is the primary blood supply to the left limb of the pancreas. The hepatic artery terminates as the cranial pancreaticoduodenal artery, which enters the body of the pancreas and courses through the proximal portion of the right limb of the pancreas. Branches from the cranial pancreaticoduodenal artery exit the pancreatic tissue and supply the closely associated duodenum. The caudal pancreaticoduodenal artery, a branch of the cranial mesenteric artery, supplies and courses through the distal portion of the right limb of the pancreas. The cranial and caudal pancreaticoduodenal arteries anastomose within the right limb of the pancreas. The anatomy of the pancreatic duct system varies among species. In 68% of dogs, transport of pancreatic secretions to the duodenum is achieved through a single duct from each limb of the pancreas.48 These ducts come together to form a Y (Figure 97-4). The tail of the Y forms the accessory pancreatic duct, or duct of Santorini, which empties into the duodenum at the minor duodenal papilla. A second duct, the pancreatic duct or duct of Wirsung, emerges from the main duct of either the right or left lobe and enters the duodenum adjacent to the bile duct at the major duodenal papilla (Figures 97-5 and 97-6). The accessory pancreatic duct is the larger of the two ducts and transports the majority of pancreatic secretions to the duodenum. Anatomic variations in the canine pancreatic duct include the presence of an accessory pancreatic duct alone and the presence of three duodenal openings. Eighty percent of cats have a single pancreatic duct that fuses with the bile duct before entering the duodenum at the major duodenal papilla.33 Figure 97-4 Anatomy of the canine pancreatic duct system. (© Copyright University of Georgia, 2001.) The two pancreatic functional units, acinar cells and islet cells,74 regulate two major physiologic processes: glucose metabolism and digestion. Inactive zymogens, including trypsinogens, chymotrypsinogens, proelastases, procarboxypeptidases, and prophospholipases, are produced and stored in the acinar cells. These inactive zymogens, along with the coenzymes, enzymes (lipase and α-amylase), and inhibitors produced in the pancreas facilitate digestion of proteins, lipids, and polysaccharides and prevent autodigestion of the pancreas (Table 97-1). In the duodenum, the activation peptide is cleaved from trypsinogen by enteropeptidase, which is produced by enterocytes of the duodenal mucosa (Figure 97-7). This cleavage produces active trypsin. Trypsin in turn cleaves activation peptides from the other zymogens to produce active digestive enzymes chymotrypsin, elastase, carboxypeptidase, and phospholipase. Trypsin, chymotrypsin, and elastase are endopeptidases that cleave peptide bonds at specific sites within polypeptide chains. Carboxypeptidase is an exopeptidase that cleaves specific carboxyl terminal residues. Phospholipases hydrolyze fatty acids of some membrane phospholipids. Lipase, in the presence of colipase, hydrolyzes ester bonds in triglycerides, and α-amylase hydrolyzes starches. (From Feldman EC: Sleisenger and Fordtran’s gastrointestinal and liver disease, ed 9. Copyright © 2010 Saunders, an imprint of Elsevier. Adapted from Gorelick F, Pandol SJ, Topazian M: Pancreatic physiology, pathophysiology, acute and chronic pancreatitis. Gastrointestinal Teaching Project. American Gastroenterological Association, Bethesda, MD, 2003.) Exocrine pancreatic secretion is regulated through nervous and hormonal mechanisms. Anticipation, smell, or the physical presence of food within the proximal intestinal tract results in pancreatic secretion through vagal stimulation. This neurologic stimulation promotes flow of pancreatic secretions into the duodenum. More importantly, movement of partially digested food and gastric acid into the proximal duodenum initiates hormonal stimulation of pancreatic secretion. Duodenal mucosal cells release secretin and cholecystokinin, which are absorbed into the blood. In the pancreas, secretin stimulates secretion of large volumes of bicarbonate-rich fluid that is important for neutralization of gastric acid within the duodenum. Cholecystokinin stimulates secretion of digestive enzymes. In the absence of food, there is a low basal rate of exocrine pancreatic secretion. Ingestion of a meal induces a biphasic response in pancreatic secretion. The initial phase is rich in pancreatic enzymes and peaks 1 to 2 hours after eating. The second phase is rich in bicarbonate and peaks 8 to 11 hours after eating.9,12 Healing of the pancreas has been studied primarily in experimental models of pancreatitis.6 Injury of acinar cells results in inappropriate protease activation that overcomes endogenous antiprotease defenses. The consequence is an initial cellular response of polymorphonuclear leukocyte infiltration into the perivascular regions of the pancreas followed a few hours later by accumulation of macrophages and lymphocytes. Oxygen radicals derived from phagocytes damage pancreatic capillary endothelial cells and propagate a cycle of migration of inflammatory cells from the leaky vasculature into the pancreatic tissues. This inflammatory cascade is reversible; however, in some cases, it progresses to necrotizing pancreatitis characterized by extensive acinar necrosis, interstitial microabscess formation, peripancreatic fat necrosis, microvascular thrombosis, and local hemorrhage. Significant fluid sequestration can occur with the intense inflammatory response, resulting in hypovolemia in severe cases. A systemic response to the local inflammatory mediator products may result in multiple organ dysfunction and systemic inflammatory response syndrome. In chronic conditions, irreversible parenchymal destruction and fibrosis may occur within and between lobules, as well as around ducts. Fibrosis is believed to be the result of proliferation and differentiation of pancreatic myofibroblasts or “stellate” cells. Interestingly, although rats with induced pancreatitis develop collagen deposits in the interstitial areas of the pancreas, with the greatest amounts seen at 7 days, the changes completely resolve within 18 days after the onset of pancreatitis.77 Proliferation of acinar cells occurs as early as 72 hours after induction of acute pancreatitis in a rat model and persists for longer than 1 week.20 Factors that stimulate acinar cell proliferation are not well characterized; it is believed that the intestinal hormone cholecystokinin is a potent trophic factor for the pancreas. Healing of the pancreas after biopsy has been evaluated in normal dogs and cats. In one study comparing partial pancreatectomy techniques in dogs, leukocyte infiltration and fibrosis were seen on histopathologic examination of the sites 7 days after biopsy. Clinical and enzymatic indicators of pancreatitis were not different from those in control dogs.1 Although the degree of inflammation incited by surgery of the pancreas in diseased animals has not been studied extensively, it is reasonable to assume that injury of the pancreas occurs with any pancreatic procedure, whether it is routine biopsy or excision of a pancreatic neoplasm. Suture fracture technique (Figure 97-8) or blunt dissection and ligation (Figure 97-9) may also be used to obtain pancreatic tissue for histologic examination. Before performing either technique, the mesoduodenum or the deep leaf of the greater omentum must be incised to gain access to the desired portion of the pancreas. Blunt dissection and ligation can be used in virtually any region of the pancreas as long as care is taken to avoid the duct system and the major vascular supply of the area. If lesions are in the proximal portion of the right lobe or the body of the pancreas, care must be taken to avoid damage to the pancreaticoduodenal vessels to prevent duodenal necrosis. In the blunt dissection and ligation technique, pancreatic tissue to be removed is isolated from the remaining pancreas by blunt dissection between lobules with a Halsted mosquito hemostat or sterile cotton swabs (see Figure 97-9). Associated vessels and ducts are ligated, the pancreatic tissue is excised, and the mesoduodenum is closed. The suture fracture technique is performed by passing nonabsorbable suture through incisions in the mesoduodenum and around the pancreas proximal to the tissue to be removed (see Figure 97-8). The suture is tightened, crushing the pancreatic parenchyma and occluding associated ducts and vessels. Pancreatic tissue distal to the ligature is excised, and the mesoduodenum is closed. The clinical impact of pancreatic biopsy has been evaluated in a relatively small number of dogs and cats, and the studies that have been done have used normal animals, not animals with pancreatic disease.1,40 In experimental comparison of suture fracture and blunt dissection and ligation techniques for pancreatic biopsy in dogs, no differences in clinical signs and serum amylase or serum lipase measurements were seen between groups after biopsy.1 Histopathologic examination of pancreatic biopsy sites revealed a more severe inflammatory reaction in dogs undergoing suture fracture biopsy; however, the increased inflammation did not result in clinical signs one week after surgery, adhesions to the pancreas were noted in 3 of 19 dogs.1 In a study of surgical biopsy of the pancreas in 15 normal cats, mild nonspecific clinical signs after surgery included swelling at the abdominal incision (3 of 15), pyrexia (3 of 15), mild pain upon abdominal palpation(15 of 15), and one episode of vomiting (1 of 15).40 Follow-up evaluation of these cats revealed small (0.5 to 1.0 cm diameter) adhesions in 13 of 15 cats and extensive (3 to 5 cm diameter) adhesions in 2 of 15 cats. Laparoscopic and laparoscopic-assisted pancreatic biopsy have been reported in dogs and cats.3,17,24,44,71 Two or three ports may be used, with placement of the third port based on the ease of identification and isolation of the pancreas, the need for a second instrument, or the need for biopsy or manipulation of other organs. The actual biopsy sample may be obtained by use of a 5-mm cup biopsy forceps, a pretied loop ligature (Figure 97-10), vessel-sealing device, hemostatic clips placed in a V shape around the tissue to be excised, or harmonic scalpel.44 Cosford et al.17 reported the findings of six normal cats that underwent laparoscopic punch biopsy of the left limb of the pancreas. No significant difference was noted in postoperative clinical signs compared with cats undergoing laparoscopy without pancreatic biopsy. After 4 weeks, there was evidence of mild adhesions in two of six cats and microscopic evidence of focal pancreatitis or steatitis in five of six cats. These findings were interpreted as normal healing of the pancreatic biopsy sites.17 Laparoscopic pancreatic biopsy in normal dogs has also been performed without significant clinical consequences.3,24,71 Suture fracture and blunt dissection and ligation techniques described above for pancreatic biopsy may be used to perform partial pancreatectomy. Stapling techniques for partial pancreatectomy have also been reported.4 Indications for partial pancreatectomy include focal trauma or presence of an isolated mass, pseudocyst, or abscess. Provided that the duct to the remaining portion of pancreas is left intact, 75% to 90% of the pancreas can be resected without impairment of exocrine or endocrine function.46 The canine pancreas has substantial regenerative capacity. In one study, pancreatic weight 6 weeks after a 95% partial pancreatectomy had increased 50% above the original weight of the 5% remnant pancreas.25 The authors suggested that expression of insulin-like growth factor-1, which was induced within 24 hours of surgery and reached maximal levels 3 days after surgery, was partly responsible for the regeneration rate seen in this study. Multiple techniques have been described for total pancreatectomy.14,19,38,53 For each technique, the method for removal of the left lobe of the pancreas is the same. The ventral leaf of the omentum is torn to expose the pancreas. Branches of the splenic artery are identified and ligated as the left lobe of the pancreas is gradually dissected free from the deep leaf of the omentum. Obstruction of the splenic artery requires splenectomy. As the dissection continues in the direction of the body of the pancreas, branches of the common hepatic and gastroduodenal arteries that enter the pancreas are identified, ligated, and transected. At the angle of the pancreas, care is taken to identify and preserve the gastroduodenal artery and its terminal branch, the cranial pancreaticoduodenal artery, to maintain blood flow to the proximal duodenum. There are three possible methods for removal of the remainder of the right limb of the pancreas. The avulsion technique integrates blunt dissection of the pancreatic tissue from the pancreaticoduodenal vessels while maintaining blood supply to the duodenum. A second technique, originally described as a method to facilitate experimental pancreatic transplantation, preserves blood supply to the duodenum through preservation of the recurrent duodenal branch of the right gastroepiploic artery.14 Preservation of this recurrent duodenal artery reportedly provides adequate blood flow to the duodenum after ligation and transection of the cranial pancreaticoduodenal artery where it enters the pancreas. A third technique is to maintain as much duodenal blood supply as possible while ligating and transecting the communication between the cranial and caudal pancreaticoduodenal arteries near the caudal duodenal flexure.50 Although duodenal necrosis did not occur with the latter technique, cyanosis of the duodenum was routinely evident. Pancreaticoduodenectomy combines the techniques for total pancreatectomy (described above) with excision of the pylorus and duodenum (described in Chapters 91 and 92, respectively). The duodenum is resected to the level of the distal end of the right lobe of the pancreas. The bile duct is ligated and transected as it enters the duodenum. Cholecystoenterostomy (described in Chapter 95) is required to establish biliary drainage. The patency of the gastrointestinal tract is reestablished through a gastroenterostomy procedure.
Pancreas
Anatomy
Vascular Supply
Pancreatic Ducts
Physiology
Digestion
Regulation of Exocrine Pancreatic Secretion
Healing of the Pancreas
Specific Surgical Procedures
Blunt Dissection Technique
Suture Fracture Technique
Effects of Open Surgical Pancreatic Biopsy
Laparoscopic Pancreatic Biopsy
Partial Pancreatectomy
Technique
Pancreaticoduodenectomy
Stay updated, free articles. Join our Telegram channel

Full access? Get Clinical Tree
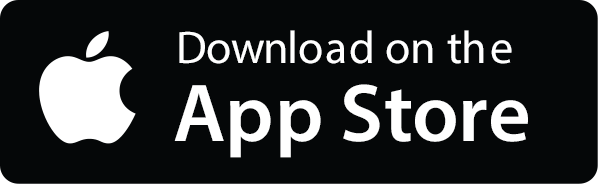
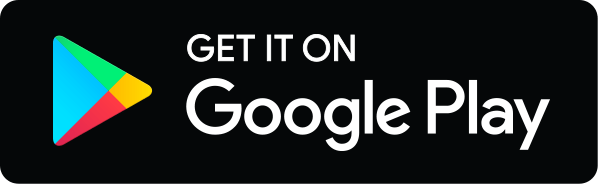