Chapter 4 Stem cells hold tremendous promise for the treatment of many ailments regardless of the inciting cause, including traumatic lesions and degenerative or heritable disorders. Stem cell therapy has been investigated in every body system, including musculoskeletal,2,23 cardiovascular,20 endocrine (such as for diabetes or liver failure),7 integumentary,28 and peripheral and central nervous systems.19,36 Although many of these studies have been carried out in laboratory animals with iatrogenic rather than naturally occurring disease, the data appear promising for clinical application.15 In humans, phase I clinical trials have demonstrated safety while investigating the therapeutic potential of stem cells in large bone defects, osteogenesis imperfecta, and hematopoietic disease, and in the treatment of graft-versus-host disease.12,18,28 However, much remains to be learned about stem cells, including the precise mechanisms by which they provide a therapeutic effect. In fact, it should be emphasized that the field of stem cells is still a relatively new research discipline, with continuing and developing paradigm shifts. Three broad categories of stem cells have been identified: embryonic stem cells, fetal or perinatal stem cells, and adult stem cells (including mesenchymal/stromal stem cells, hematopoietic stem cells, and neural stem cells). A recent addition is the induced pluripotent stem cell (iPS cell), produced by reprogramming of adult somatic cells. Stem cells are defined as undifferentiated cells that possess the ability to divide for indefinite or prolonged periods in culture and may give rise to highly specialized cells characteristic of each embryonic germ layer (mesoderm, ectoderm, endoderm).30 Although the fertilized zygote is characterized as totipotent, embryonic stem cells are considered pluripotent, and adult stem cells are multipotent, referring to the ability of each stem cell type to differentiate into one or more germ layer lineages. Because the types of test(s) used to define stemness differ between the various types of stem cells and often are not standardized, considerable debate and confusion have arisen in the literature concerning the identity of a stem cell. Organizations such as the U.S. National Institutes of Health Stem Cell Registry (http://stemcells.nih.gov/research/registry/pluripotentcriteria.asp) and the International Society for Cellular Therapy have attempted to generate a “gold-standard” definition and a set of criteria by which stem cells should be defined.6,30 Such criteria include the ability of the stem cell to differentiate in vitro into multiple cell lineages, such as osteoblasts, chondrocytes, and adipocytes, and for embryonic stem cells, the ability to form teratomas when injected into immunodeficient mice. The ultimate validation of pluripotency, applicable to embryonic stem and iPS cells only, is germline transmission (i.e., introduction into blastocysts will result in the production of chimeric organisms that are germ line competent), which is testable only for nonhuman animals for obvious ethical and legal reasons. Embryonic stem cells are derived from the inner cell mass of preimplantation blastocysts. They were isolated first from mice in 19818 and then from humans in 1998.33 Canine embryonic stem cells capable of in vitro and in vivo (teratoma formation) differentiation were described in 2009.35 To date, feline38 and equine16 embryonic stem-like cells have been described, but neither type has demonstrated in vivo differentiation capabilities. Embryonic stem cells from these companion animals can be used for many purposes, the most obvious of which is for embryonic stem cell–based therapy to treat animals suffering from a variety of diseases. In addition, embryonic stem cell technology is being used in conservation of endangered species of carnivores and in biomedical research, because many of the diseases and musculoskeletal injuries of cats, dogs, and horses are similar to those seen in human beings.32,34 Many rodent model studies have demonstrated the ability of embryonic stem cells to augment nerve injury repair,36 but no clinical reports have described embryonic stem cell use in animals. Open-label prospective studies on the use of embryonic stem-like cells in canine osteoarthritis and in equine tendon injuries are under way, and a double-blind randomized prospective study on the use of fetal stem cells in horses is being conducted (in 2010), but no data are currently available (Celavet; www.celavet.com). In early 2009, the U.S. Food and Drug Administration (FDA) gave approval for the first phase I clinical trial (to demonstrate safety in human beings) for transplantation of human embryonic stem cells into patients with spinal cord injury. The trial has been on hold but is expected to resume in mid-2010. Hematopoietic stem cells capable of forming all types of blood cells reside in the bone marrow and are the target cells in bone marrow transplantation. These stem cells display the cell surface marker CD34 and can be recovered from bone marrow aspirate or blood for clinical application and engraftment to a recipient who has been immunodepleted with total body irradiation for diseases such as leukemia. Hematopoietic stem cell transplantations can be autogenous or allogeneic with major histocompatibility complex donor–recipient matching. Some of the current research efforts in the hematopoietic stem cell field are directed toward gene therapy approaches for genetically based diseases, such as canine leukocyte adhesion deficiency, which is similar to leukocyte adhesion deficiency in people.20 The similarities between canine and human leukocyte adhesion deficiency exemplify the use of naturally occurring animal diseases to develop stem cell–based therapies that ultimately will benefit both animal and human patients. When mesenchymal/stromal stem cells are considered as a candidate cell type for tissue engineering and regeneration, they should not be thought of simply as a replacement cell population, for example, to replenish matrix-producing cells within a tissue. Convincing and reproducible evidence indicates that mesenchymal/stromal stem cells also function to secrete trophic factors that affect surrounding cells, as well as cytokines and chemokines that modulate local immune and inflammatory reactions.2,24,27 It is currently thought that mesenchymal/stromal stem cells are immunotolerant, and that the clinical use of allogeneic stem cell therapy is likely to be safe. However, conflicting data have come from various laboratories regarding immune tolerance of allogeneic mesenchymal/stromal stem cells. Caution should thus be exercised when their application is considered, until further research has been conducted. In bone marrow transplantation, a commonly accepted paradigm is that recipients of allogeneic hematopoietic stem cells develop transplantation tolerance, and that only a short course of immunosuppressive therapy is needed. Existing data for mesenchymal/stromal stem cells are entirely supportive of this concept.25 Clear deficiencies exist in the current understanding of transplantation tolerance after allogeneic hematopoietic stem cell therapy, and current methods of detecting graft-versus-host disease are deficient. For example, mesenchymal/stromal stem cells inhibit the T-cell alloresponse in a dose-dependent manner, and they inhibit T-cell proliferation in response to allogeneic stimuli.13 The effects of mesenchymal/stromal stem cells on T-cell proliferation appear to be contextual where mesenchymal/stromal stem cells inhibit T-cell proliferation in proinflammatory conditions, but these effects are lost in the presence of antiinflammatory cytokines.26 These beneficial effects have led to the use of mesenchymal/stromal stem cells for immunomodulatory therapy for graft-versus-host disease, ischemic heart disease, and stroke.3 The trophic effects of mesenchymal/stromal stem cells are mediated via their release of soluble factors such as cytokines and growth factors that diminish tissue injury, promote neovascularization, recruit and induce proliferation of resident tissue stem cells, and inhibit fibrosis.9 In a hamster model of heart failure, administration of mesenchymal/stromal stem cells into cardiac muscle increased circulating levels of hepatocyte growth factor and leukemia inhibitory factor, which were associated with mobilization of myocardial progenitor cells.29 In the myocardium surrounding the injected mesenchymal/stromal stem cells, expression of reparative cytokines such as insulin-like growth factor-2 and vascular endothelial growth factor was also increased. Furthermore, mesenchymal/stromal stem cells can function as carriers for therapeutic genes. For example, bone marrow–derived mesenchymal/stromal stem cells carrying the human insulin gene were injected intrahepatically to diabetic beagles. The diabetes was effectively relieved for up to 16 weeks following injection, suggesting that mesenchymal/stromal stem cells could be used in a gene therapy approach to type 1 diabetes.39 Recently, iPS cells have been added to the arsenal of stem cells. iPS cells are generated by the reprogramming of adult or embryonic somatic cells, such as skin fibroblasts, via transduction of a specific set of transcription factor genes.17,22,31,37 The reprogrammed iPS cells exhibit most, if not all, of the characteristics of embryonic stem cells, including embryoid body formation in vitro, chimeric integration, germ-like transmission and teratoma formation in vivo, and expression of embryonic stem cell–specific gene markers. iPS cells exhibit similar levels of pluripotency as embryonic stem cells but can be derived from the individual patient, and therefore may be without associated ethical or allogeneic complications and possible immune rejection issues. The capacity for iPS cells to undergo induced differentiation into multiple cell lineages, including neuronal cells, adds to the excitement surrounding their therapeutic potential. Ideally, each animal or person would have iPS cells generated from his or her own cells and stored for application at the time of need. This would generate a source of autogenous cells for each animal or person and would obviate the need for culture expansion of mesenchymal/stromal stem cells. Generation of iPS cells has clearly demonstrated the ability of lineage-specific cells such as fibroblasts to convert/revert back to stem cells. This contrasts with the original stem cell paradigm, in which it was thought that differentiation from a stem cell into a lineage-specific cell was unidirectional and linear. It is now known that specialized, lineage-specific cells can be directly converted from one lineage to another, including mesenchymal/stromal stem cells that have previously been differentiated along specific pathways (Figure 4-1).10 iPS cell biology is a rapidly evolving field of stem cell research that has tremendous promise because of the mitigation of ethical concerns surrounding embryonic stem cells and potential for immunorejection associated with allogeneic transplantation of mesenchymal/stromal stem cells. Presently, iPS cells are most efficiently generated using viral transduction of the genes required for nuclear reprogramming. Clear health concerns surround the use of virus-based gene transduction, but this approach is likely temporary with rapid advancements in the field of iPS cell research. The clinical promise of this nuclear reprogramming technology was underscored by a recent report in which sickle cell anemia in a humanized mouse model was rescued by creating autologous iPS cells, correcting the globin locus by gene targeting, differentiating iPS cells into hematopoietic precursors, and transplanting them into mutant mice.11 Use of iPS cells also avoids ethical concerns regarding destruction of embryos to generate embryonic stem cells. However, significant hurdles and complications may be associated with clinical applications of human iPS cells, including their safety and potential tumorigenicity, and the costs of developing and testing iPS cells from individual patients. Much research is thus needed to realize the practical application of iPS cells by overcoming these hurdles and complications.
Stem Cells and Regenerative Therapy
Stem Cells in Regenerative Surgical Strategy
< div class='tao-gold-member'>
Stay updated, free articles. Join our Telegram channel

Full access? Get Clinical Tree
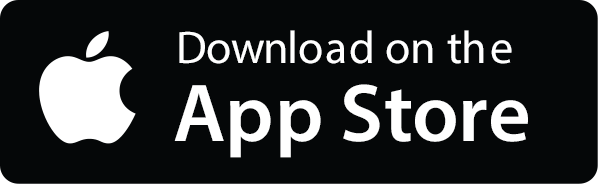
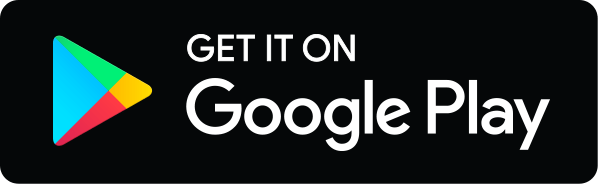