© Springer Japan 2016
Shinichi Ohno, Nobuhiko Ohno and Nobuo Terada (eds.)In Vivo Cryotechnique in Biomedical Research and Application for Bioimaging of Living Animal Organs10.1007/978-4-431-55723-4_3434. Overview on Recent Applications of In Vivo Cryotechnique in Neurosciences
(1)
Department of Anatomy and Molecular Histology, Interdisciplinary Graduate School of Medicine and Engineering, University of Yamanashi, 1110 Shimokato, Chuo City Yamanashi, 409-3898, Japan
(2)
Division of Health Sciences, Shinshu University Graduate School of Medicine, 3-1-1 Asahi, Matsumoto City Nagano, 390-8621, Japan
Abstract
When tissue specimens are prepared for microscopic observation, commonly used methods using chemical fixatives and dehydration can yield artifacts in morphology and molecular distribution. Conventional quick-freezing and high-pressure freezing methods, where specimens are cryofixed after resection, can also cause artifacts due to anoxia and ischemia, and only dead morphological states without blood circulation can be observed with these methods. By contrast, all cells and tissues in animal bodies can be cryofixed in situ by “in vivo cryotechnique” (IVCT), which prevents these artifacts caused following removal of target tissues. In the central nervous system, distribution of small molecules, dynamic changes of signaling proteins, ultrastructures of extracellular space, and blood–brain barrier integrity have been examined using IVCT. To acquire novel information in cryofixed tissues, new imaging methods including Raman microscopy were combined with IVCT. New approaches to observe “living” states in the nervous system will be possible with IVCT.
Keywords
Extracellular spaceBlood–brain barrierSignaling proteins Raman microscopy 34.1 Introduction
Functional aspects are reflected in the structures of cells and tissues in living animal organ s . A widely used method for structural analyses has been the microscopic analyses. Recently, there was dramatic progress in technology of light microscopy . Dynamic signal molecules and their interactions can be visualized in living cells and animals by the fluorescence imaging. “In vivo cryotechnique (IVCT)” has been used to observe dynamic structures and molecular localization in living animal organs at light and electron microscopic levels [1]. Cryobiopsy has been also developed for clinical application. Here, technical aspects of IVCT and recent findings obtained by IVCT in the field of neurosciences will be reviewed.
34.2 Problems of Conventional Preparation Methods
Chemical fixation , dehydration , embedding, sectioning, and dye or metal staining steps have been widely used for animal nervous system to examine morphology at the light and electron microscopic levels. Artifacts affecting the final structures are caused during these tissue preparation steps [2, 3]. Perfusion or immersion with chemical fixative s , such as paraformaldehyde , glutaraldehyde , and osmium tetroxide , is commonly used for chemical fixation steps [4]. Molecular movement and/or structural changes are one of the problems caused during the chemical fixation [3]. In addition, there is a limitation of chemical fixation to preserve transient or dynamic morphology, since there are time intervals before completion of fixation [5]. Subsequent alcohol dehydration steps cause tissue shrinkage [6, 7]. One of the alternative approaches to reduce such artifacts is the cryofixation method.
The cryofixation is one of the physical fixation methods and embeds tissues in vitrified ice [8]. Various methods for cryofixation have been developed to meet the requirement. Major methods include the so-called slamming quick-freezing or the plunging quick-freezing methods, where resected tissues are quickly frozen by slamming onto precooled metal blocks or plunging into precooled liquid cryogen s. In high-pressure freezing, tissues are cryofixed under very high pressures, and vitrification in relatively broad tissue areas can be achieved. After the cryofixation, many preparation methods can be used, including the freeze-substitution (FS) [5, 9]. Although cryofixation methods can reduce the artifacts and preservation of better morphology [8], it is necessary to collect tissues out from living animal organ s in the conventional quick-freezing method s, and morphology and molecular distribution can be influenced by loss of blood supply and ischemia /anoxia [10, 11].
34.3 Development of In Vivo Cryotechnique and Cryobiopsy
Living animal organs can be directly cryofixed in vivo by the IVCT without tissue resection. Blood circulation of living tissues can be maintained by IVCT until the moment of cryofixation , and the artifacts caused by ischemia or anoxia can be minimized [12]. Additional merit of cryofixation is achievement of high time resolution [8]. After IVCT, many preparation procedures are available, as in the cases of the other cryofixation methods. The antigen retrieval procedures can be omitted in the specimens prepared with cryotechniques [13].
One of a few drawbacks in IVCT is that well-frozen areas are restricted to less than 10 μm depth from the surface of the tissues at an electron microscopic level [11, 14–17]. Due to the low spatial resolution at the light microscopic level, the width of the areas without visible ice crystal s is about a few hundred micrometers deep from the surface [12, 13]. Another drawback of IVCT is that exposure of the target organs in living animals is necessary to pour the cryogen directly onto the organs and minimize formation of observable ice crystals.
Although ultrastructural analyses were the initial application of IVCT, IVCT has been also applied to many light microscopic studies [18]. However, cryogen is poured over the animal bodies; it is difficult to obtain serial specimens from single animals with IVCT. In “cryobiopsy ,” serial tissue samples can be pinched off from single anesthetized animals with precooled cryobiopsy forceps (−196 °C). Most methods for preparation of frozen tissues are possible in the following preparation for these biopsied tissues [18, 19]. In the following section, the four aspects of IVCT will be discussed with particular emphasis on recent application of IVCT to the neuroscience fields, including (1) brain morphology without ischemic/anoxic artifacts , (2) molecular localization in combination with new bioimaging techniques, (3) localization of soluble molecule, and (4) rapidly altering signal proteins.
34.4 Brain Morphology Without Ischemic/Anoxic Artifacts
Asphyxia following tissue resection or during chemical fixation causes the entrance of extracellular water and electrolytes into the intracellular compartment and decreases extracellular space s [20–22]. To overcome this problem, ultrastructures of mouse cerebellar cortex were analyzed with IVCT [23]. With IVCT, large extracellular spaces could be observed, and these spaces in molecular and Purkinje cell layers are distributed among cellular profiles and synaptic clefts in mouse cerebellar cortex. The large extracellular spaces were maintained with IVCT after 30 s ischemia , but mostly diminished with IVCT after 8 min of ischemia or with the conventional quick-freezing method . The extracellular spaces were abundant around some synaptic clefts, whereas other synaptic clefts were totally or partially covered with glial processes [23]. The estimated sizes of extracellular spaces around the open and enclosed synaptic clefts were not significantly different, and such extracellular spaces presumably support translocation of various extracellular components [24]. It was considered that most dendritic spines bearing synapses are ensheathed by Bergmann glia l processes in cerebellar cortex, and more than half of the circumferential length around the synaptic clefts were covered with cytoplasmic sheaths of Bergmann glia [25]. The perisynaptic glial processes are considered critical for the glial functions related to synapses [26–28]. The glial processes around synaptic clefts are considered to extend or retract through the perisynaptic extracellular spaces, and the extracellular spaces support the dynamic alterations of glial processes. Since cellular profiles might be swollen during tissue preparation steps with conventional preparation methods, a careful reevaluation of ultrastructural differences among different areas of molecular layers in the cerebellar cortex may be necessary using IVCT.
34.5 Molecular Localization in Combination with New Bioimaging Techniques
Novel information about the structures of molecules under normal conditions and diseased states can be obtained with microscopic approaches, including fluorescent, infrared, and Raman microscopy , which can detect the presence of biological molecules and/or drugs [29]. Raman microscopy can visualize different molecular states/structures in biological materials [30]. To preserve and observe the distribution and/or structures of molecules, e.g., proteins, lipids, carbohydrates, and vitamins, in living animal nervous system, the eyeball tissue sections prepared with IVCT followed by freeze-drying were observed under the confocal Raman microscopy, and mapping of the Raman spectra was obtained [31]. The Raman spectra could be largely classified into four patterns, which represented histologically different layers. One Raman spectrum corresponded to melanin and distributed mainly in the choroid and pigment cell layers. Another Raman spectrum represented hemoglobin and was obtained from the blood vessel s in the sclera, whereas another spectrum was similar to the purified rhodopsin and distributed in the photoreceptor layer. The Raman spectra obtained in the freeze-dried samples and control solution samples were similar, presumably due to the less altered structures of biomolecules in the freeze-dried specimens. The IVCT was also combined with confocal cryo-Raman microscopy , where specimens are observed at low temperature (<−150 °C). By this approach, oxygen saturation levels of flowing erythrocyte s in frozen tissue specimens were directly observed [32]. From these studies, Raman microscopy can distinguish multiple components in living bodies, and IVCT can be combined with Raman microscopy to examine distribution and structures of biological and chemical components which are changing quickly in living animal organ s .
34.6 Precise Localization of Soluble Molecule
The central nervous system possesses a selective molecular barrier between blood vessel s and parenchyma, which has been called the blood–brain barrier (BBB). BBB plays critical roles in brain functions and diseases such as neurodegenerative disorders [33]. IVCT was used to examine extravasation of intrinsic serum soluble protein s in the previous study [12]. IVCT followed by FS can minimize diffusion and translocation of soluble serum molecules before fixation and can clarify the precise distribution of IgG and albumin in cerebellar tissues. Under the normal condition, serum IgG and albumin were localized exclusively inside the cerebellar blood vessels. However, the immunostaining for the serum protein s was observed around the blood capillaries in the cerebellar parenchyma when the brain tissues were cryofixed within a minute after removal. The serum protein diffusion through BBB after tissue removal could be attributable to leakage of the serum components. Using IVCT, injected extrinsic molecules could be successfully detected in multiple organs [19, 34, 35]. IVCT followed by FS is useful to examine the extravascular leakage of soluble molecules under pathological conditions of animal brains, given that serum proteins could not be detected outside blood vessels under normal conditions.
IVCT and subsequent FS were also used to examine distribution of glutamate , which had been controversially detected in inner segment s of photoreceptors [36]. With IVCT, the most intense immunostaining for glutamate was observed in the specimens freeze-substituted with acetone containing paraformaldehyde and glutaraldehyde . Glutamate was clearly observed in the inner segments, outer and inner plexiform layers, and ganglion cell layers. In previous studies in the goldfish [37], cats [38], chickens [39, 40], rats [41], and monkeys [41], immersion fixation revealed glutamate immunoreactivity in the inner segment of the eyes. When the animals were perfused with fixatives, the immunostaining for glutamate could not be detected in the inner segment [42, 43]. Thus, it was suggested that postmortem changes caused by ischemia /anoxia might affect distribution of glutamate in the inner segment. Since the effect of ischemia/anoxia can be minimized by IVCT, the clear glutamate immunoreactivity observed with IVCT in the inner segment of photoreceptors suggests that the glutamate immunoreactivity is not caused by ischemia/axoxia. It was reported that the inner segment also contains highly active enzymes related to the glutamate metabolism [44, 45]. IVCT will be a powerful tool to examine living animal nervous system and detect distribution of small molecules including glutamate. In addition, concentrations of fixatives such as glutaraldehyde need to be optimized to cross-link and retain small molecules and not to hinder the antibody binding.
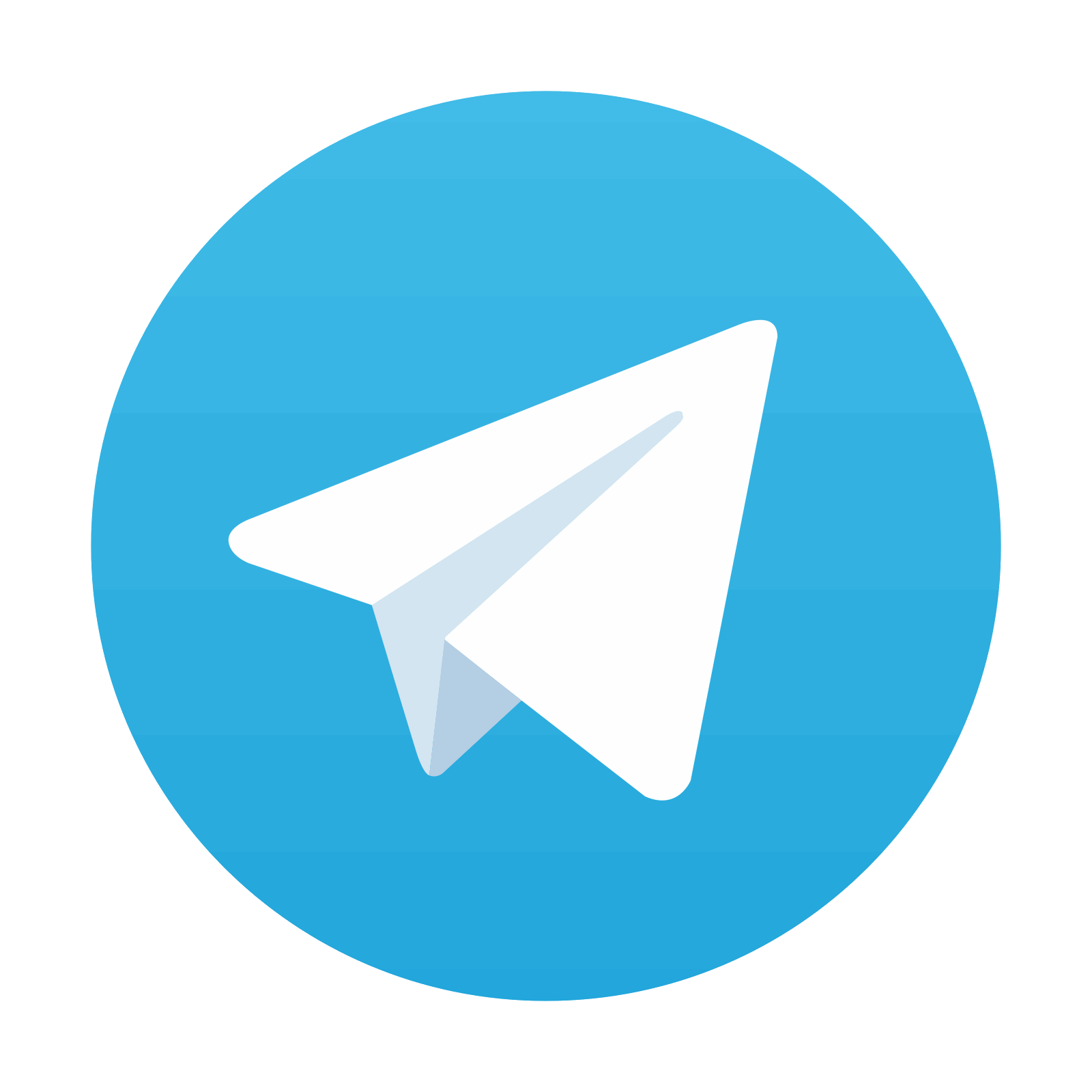
Stay updated, free articles. Join our Telegram channel

Full access? Get Clinical Tree
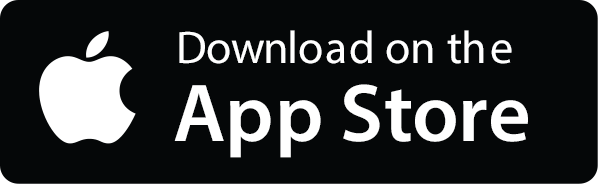
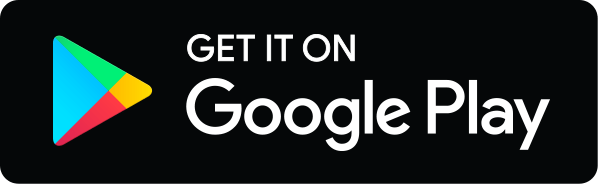