28 Obesity
Definition of obesity
Obesity can be simply defined as an expanded mass of adipose tissue in the body (Figs 28.1 and 28.2). Obesity also can be defined as a disease in which excess body fat has accumulated to such an extent that health may be adversely affected. In human populations, obesity has been defined by either direct (e.g., magnetic resonance imaging (MRI) or dual-energy X-ray absorptiometry (DEXA) imaging) or indirect (e.g., bioelectrical impedance) quantitative assessment of body fat content, or has been based on anthropometric approaches that utilize simple clinical measures such as body mass index (BMI; weight/height2, kg/m2) or waist circumference (WC; Bosy-Westphal et al 2006). At the population level, measurement of body fat mass has no advantage over BMI or WC in the prediction of obesity-related metabolic risk (Bosy-Westphal et al 2006, Tulloch-Reid et al 2003), and BMI is the most widely used index for evaluation of being overweight or obese in human patients (Romero-Corral et al 2008). BMI categorizes people as normal or “healthy” weight (BMI 18.5 to 24.9), overweight (BMI 25 to 29.9), obese (BMI 30 to 34.9), or severely obese (BMI ≥ 35). In large-scale population studies there is a close relationship between BMI and incidence of several chronic conditions associated with excess fat (e.g., diabetes mellitus, hypertension, cardiovascular disease; Kopelman 2000). The underlying assumption is that most variation in weight for persons of the same height is due to fat mass. However, BMI may not reflect the same degree of fatness (adiposity) across different populations due to differences in race/ethnicity, age and body proportions. BMI does not differentiate between elevated body fat content and preserved or increased lean mass (e.g., in athletic individuals). As well, BMI is insensitive to variations in body fat distribution (e.g., central obesity) that are important predictors of metabolic and cardiovascular risk (Romero-Corral et al 2008, Shea et al 2011).
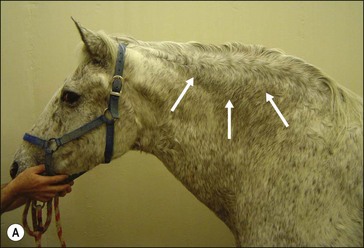
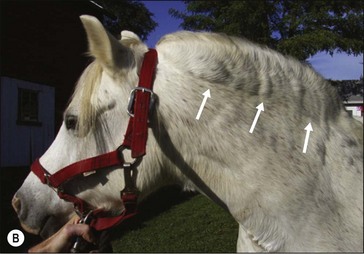
Figure 28.2 Images of a horse A) and pony B) with prominent nuchal crest subcutaneous fat deposition (“cresty neck”).
There is no universal definition of obesity in horses and ponies. As described in Chapter 22, horses or ponies with a body condition score (BCS) 7 (using the Henneke 9-point system; Henneke et al 1983) are considered overweight while animals with BCS of 8 or 9 are obese. Minimal data are available on body fat mass in horses and ponies, although recently the deuterium oxide dilution technique has been validated for the measurement of body fat in the Welsh mountain pony (Dugdale et al 2011a). In general, it appears that body fat is likely to be greater than 20–25% of body mass in animals with BCS ≥ 7, and values of up to 47% have been measured in ponies (Dugdale et al 2011a). It is important, however, to note limitations of BCS for assessment of adiposity (Dugdale et al 2012). Fundamentally, the BCS system provides a measure of “superficial flesh” but it does not assess subcutaneous fat independent of muscle mass. The current BCS systems also do not evaluate fat within the abdominal cavity, nor do they allow differentiation between obese (i.e., Henneke BCS 9) and “super obese” individuals. Studies in French sport horses and Welsh ponies have demonstrated an exponential relationship between BCS and body fat content (Martin-Rosset et al 2008, Dugdale et al 2011a) although recent work has suggested that the relationship may be more appropriately explained by a power function (body fat % Kave2). Whereas there is a linear relationship between BCS and total fat mass in animals with BCS < 7, BCS is a very insensitive indicator of fat mass at BCS ≥ 7. Ultrasound determinations and the use of BIA (see Chapter 22) have the potential to provide some useful information as to fat content and distribution but much more work is needed to develop simple yet accurate methods for estimation of adiposity (including the distribution of fat mass) in horses and ponies. The availability of such methods will facilitate the development of breed-specific definitions of obesity and improve knowledge of the relationship between adiposity and disease risk.
Recently, there have been several reports of different subtypes of obesity in human populations including individuals that are obese but metabolically healthy (“healthy obesity”) and those that are metabolically obese but normal weight (MONW) (Wildman et al 2008, Romero-Corral et al 2010). Although they have normal BMI, people with MONW have a clustering of metabolic and cardiovascular risk factors normally associated with obesity, including increased triglyceride and cholesterol concentrations and systemic inflammation. In US populations, 32% of phenotypically obese people are metabolically normal, whereas 23% of subjects with BMI in the healthy range (<25 kg/m2) exhibit clustering of cardiometabolic abnormalities (Wildman et al 2008). Although mechanisms underlying differences in these phenotypes have not been fully elucidated, it is apparent that people with “metabolically benign” obesity have lower visceral, liver and muscle fat content when compared to normal weight or obese individuals with insulin resistance and other metabolic abnormalities (Karelis 2008, Stefan et al 2008). These observations highlight the need for more sensitive measures of body fat mass and distribution (e.g., DEXA, MRI) as well as measurement of various metabolic variables when assessing risk for chronic diseases. Similarly, it is likely that different obesity phenotypes exist in horse and pony populations. For example, whereas there is a negative association between BCS and measures of insulin sensitivity in horses (Hoffman et al 2003, Vick et al 2007), not all obese horses are insulin resistant. In addition, insulin resistance and hyperinsulinemia have been detected in horses and ponies with lean or moderate body condition (Bailey et al 2007).
Prevalence
The 1998 USDA National Animal Health Monitoring System (NAHMS) study estimated that approximately 1.5% of the horse population in the United States was overweight or obese (Anon 1998). However, these estimates were based on owner assessment of body condition rather than standardized methods for assessment of body fatness (e.g., BCS). Indeed, the results of recent prospective studies in which standardized methods were used for physical assessment have demonstrated that the prevalence of overweight/ obesity in pleasure horse populations is far higher than the NAHMS estimates. Wyse et al (2008) examined 319 pleasure riding horses kept at 22 horse operations in south-west Scotland and found that 112 animals (35%) were fat (defined as BCS 5/6) and 32 horses (10%) were obese (BCS 6/6). In a study of 366 horses in North Carolina, 48% were considered to be overweight or obese (BCS >6/9, Henneke scale) (Pratt-Phillips et al 2010), while a cross-sectional study of 300 mature horses in south-western Virginia reported that 97 horses (32.3%) were over conditioned (BCS 7) and 56 (18.7%) were obese (BCS 8–9) (Thatcher et al 2012).
It should be noted that the above studies were conducted during the summer months when horses may be more predisposed to weight gain due to increased availability of pasture forage and seasonal changes in feed intake and metabolism (Arnold et al 2006, Kuntz et al 2006). Nonetheless, in a small survey study (127 horses and ponies in north Somerset, England) the incidence of obesity was high (~28%) when animals were evaluated at the end of winter although prevalence was even higher at the end of summer (~35.5%; Giles et al 2012). More studies are needed to establish the prevalence of and risk factors for obesity in different populations of horses across the globe. Two recent studies have reported poor agreement between the owners’ perception of whether their horses were overweight / obese or not and the actual body condition score; less than 50% of owner estimates for fat horses agreed with the BCS (Stephenson et al 2011, Wyse et al 2008). These observations suggest that horse owners tend to underestimate body fatness in their horses and this may be a risk factor for obesity in horses, as has been reported in cats (Allen et al 2000).
Key Points
• Available data indicate that obesity is far more common than previously recognized, with the prevalence of overweight/obesity up to 50% in some subpopulations
• Current systems used to estimate body condition enable categorization of animals as thin, moderate or overweight. However, they cannot accurately predict body fat content or distribution, especially in thin (BCS<2–3/9) or fat (BCS≥7/9) animals
Potential contributing factors
The causes of obesity are likely to be multifactorial, with the involvement of genetic and environmental factors, especially overfeeding in combination with minimal physical activity. Very few studies have looked at the risk factors for obesity in horses and ponies (Thatcher et al 2012, Giles et al 2011). In the study by Thatcher and colleagues (2012), the feeding of hay reduced the odds of being over-conditioned or obese, while the feeding of up to 1.4 kg/day of complementary feed (concentrate) increased odds of being over-conditioned /obese. In the UK study (Giles et al 2011), the final logistic regression model for prediction of BCS , coming out of the winter, included breed, age, worming frequency and minutes in trot per hour of ridden exercise but not the estimated amount of feed provided; interestingly however, this model only explained 33% of the variation in BCS.
Overfeeding
Weight gain and obesity reflect an imbalance between energy intake and expenditure, although genetics may play a strong role in the face of excess caloric intake. An individual’s energy requirement is affected by several external factors such as environmental conditions and the level of exercise being undertaken (including activity during turnout) as well as innate factors such as life stage and genetics. Although no study has reported a direct correlation between the prevalence of obesity and excess energy intake, anecdotal observations point to overfeeding as a major contributing factor. Many domesticated horses and ponies spend much of the day in confinement housing (stalls or small drylots) with occasional use in riding activities – two to three times a week. In these circumstances, daily energy requirements are often no higher than maintenance levels and easily met by provision of a moderate-to-good quality forage. Yet many of these animals are also fed grains, sweet feeds and other feeds with high caloric density and/or turned out for several hours a day on energy rich pastures, such that energy intake can greatly exceed requirements. Multivariable logistic regression analysis of data from 300 pleasure horses demonstrated that the feeding of grain-concentrate (1.4 kg/day vs. none) increased the odds for horses to be overweight or obese (odds ratio 2.2) (Thatcher et al 2012). The potential for weight gain to occur with overfeeding was highlighted by the results of an experimental study in which the bodyweight of Arabian geldings was increased by 20% when they were fed 200% of maintenance energy needs over a 3-month period (with 65% of calories from sweet feed; Carter et al 2009b). In the study by Giles et al (2011), however, very little of the observed variation in BCS, in animals spending >6 h per day out at pasture, could be explained by traditional management factors such as feeding level, feed type and amount of structured exercise.
The caloric intake of animals turned out to pasture may greatly exceed requirements and contribute to weight gain, especially at times of the year when there is an abundant supply of nutrient-rich forage (e.g., during spring and early summer when environmental conditions favor forage growth and nutrient storage). Ponies provided 24-hour access to pasture may consume up to 5% of bodyweight per day as dry matter and up to 1% BW in only 3 h of pasture turnout, highlighting the potential for weight gain in pastured animals (Longland et al 2011a, b).
Disruption of seasonal patterns of feed intake and body weight regulation
Wild animals adapted to temperate climates demonstrate seasonal fluctuation of body mass in concert with a number of other physiological adaptations that are entrained to photoperiod and result in fairly stable body weight over a number of seasons (Fuller et al 2001, Arnold et al 2006, Kuntz et al 2006). Appetite is highest during spring and summer, coinciding with a plentiful supply of forages and promoting growth (young stock) and deposition of adipose tissue and weight gain (mature animals). During winter when feed is less plentiful, appetite and metabolic rate decrease and energy stored in white adipose tissue is mobilized to compensate for energy deficits due to reduced feed intake (Fuller et al 2001). These seasonal adaptations are also apparent in domesticated ponies. For example, thin and moderately conditioned Welsh Mountain pony mares provided ad libitum access to a complete feed consumed more in summer (maximal 4.6 ± 0.3% of body mass [BM] as dry matter intake [DMI] per day) than in winter (maximal 3.5 ± 0.1% BM as DM intake/day) (Dugdale et al 2011b). Interestingly, peak DMI by obese (BCS >8) ponies in this study was half that reported for non-obese ponies and the body weight remained constant in the obese ponies regardless of the season. These observations raise questions about the effect of obesity on energy requirements and appetite.
Genetics
Genetics may be another factor in the predisposition to obesity. Horse owners and veterinarians often use the term “easy keeper” to describe a horse or pony with a tendency to be overweight and that appears to require fewer calories than most horses to maintain condition. Ponies and certain horse breeds (e.g., Morgans, Arabs, Paso Finos) appear to fit this description, whereas other breeds (e.g., Thoroughbreds) fit the description of “hard keepers” that often have difficulty in maintaining bodyweight and condition. One hypothesis, as yet unproven, is that certain lines of horses and ponies have inherited genetic traits that have facilitated survival on poor quality forages and/or in the face of limited feed availability – the so-called “thrifty genotype” (Treiber et al 2006). This strategy may fail when these animals are supplied with abundant feed, particularly grains or pasture forage rich in NSC, resulting in weight gain, obesity and difficulty in sustaining weight loss once obese.
Altered hormonal regulation of appetite and energy balance
Studies in humans and laboratory animal species indicate complex regulation of appetite, body weight and adipose tissue mass. The hormones leptin and ghrelin are recognized to have a major influence on energy balance, and alterations in the leptin and ghrelin systems have been implicated in the development and/or maintenance of obesity (Klok et al 2007). Ghrelin, which is primarily produced in the stomach, appears to function as an appetite-stimulatory signal and short-term mediator of energy balance. In humans, plasma ghrelin concentrations increase during the preprandial period and the magnitude of the increase is correlated with hunger scores (Cummings et al 2004). As well, intravenous infusion of ghrelin induces hunger and food intake in people (Wren et al 2001). For this reason, ghrelin is sometimes referred to as the “hunger hormone”. In addition to its orexigenic effect in humans, ghrelin stimulates gastrointestinal motility, gastric acid secretion and pancreatic exocrine secretion, all of which increase in anticipation of meals (Delzenne et al 2011). Interestingly, ghrelin concentrations are lower in obese compared to normal weight humans, consistent with observations that appetite is reduced in the obese state (Klok et al 2007). Ghrelin concentrations and appetite increase after weight loss and persistent elevations in ghrelin may, in part, contribute to poor weight loss maintenance (Adams et al 2010). In horses, plasma ghrelin decreases in response to oral and intravenous glucose administration (Gordon and McKeever 2006), while feed intake and plasma ghrelin concentrations were higher in horses subjected to interval exercise vs. the control (no exercise) condition (Gordon et al 2006). These observations suggest that plasma ghrelin responds to nutritional signals and may play a role in appetite regulation in horses. To date, however, there have been no reports of ghrelin concentrations in obese equids before or after weight loss.
Leptin, the product of the ob gene, provides information to the brain regarding availability of body fat stores, promoting satiety and reduction in food intake when energy balance is positive or fat stores are plentiful (Spiegelman & Flier 2001). Leptin is primarily produced by adipocytes and circulating concentrations are generally in direct proportion to the amount of adipose tissue (Schwartz et al 1996). One study in horses has shown a positive association between circulating leptin concentrations and BCS (Buff et al 2002). However, other studies have shown a wide range of leptin concentrations in horses with similar apparent adiposity (Gentry et al 2002), suggesting that factors other than adipose tissue mass affect leptin production and secretion.
Leptin regulates feeding behavior by binding to central nervous system receptors (primarily in the hypothalamus) and modulating the activity of neurons in appetite control centers (Meister 2000). Leptin causes an increase in the expression of melanocortins, such as alpha-melanocyte-stimulating hormone (α-MSH), which help to regulate energy homeostasis by inducing satiety and increasing fat metabolism. In other species, it has been suggested that α-MSH is very important in the integration of metabolism and appetite. A weak positive correlation has been shown between this hormone and BMI in horses (Donaldson et al 2004). However, further work is needed to determine whether a melanocortin receptor defect contributes to the pathophysiology of obesity or alternatively whether plasma α-MSH concentration is simply a correlate of adiposity (Donaldson et al 2004).
Leptin increases energy expenditure in rodents and humans via activation of the sympathetic nervous system (Enriori et al 2006). In obese, leptin-deficient mice (ob/ob), exogenous administration of leptin reduces hyperphagia and obesity (Halaas et al 1995). However, most obese people have greater leptin concentrations than lean individuals and obesity is associated with a disturbed diurnal variation in circulating leptin (Enriori et al 2006). It remains unclear whether these abnormalities in the leptin system are a cause or consequence of obesity, but one view is that chronic over-feeding can lead to development of leptin resistance with a sustained hyperleptinemia associated with a failure of leptin to suppress appetite and increase energy expenditure. In this context, leptin resistance may contribute to the maintenance of obesity.
Plasma leptin concentration in horses is affected by energy balance and feeding state (McManus & Fitzgerald 2000, Cartmill et al 2005, Buff et al 2006). Higher plasma leptin concentrations were reported in fed horses compared with fasted horses, with higher values in the afternoon than the morning only in the fed horses (Buff et al 2006). Plasma leptin concentration decreases in response to short-term feed restriction (McManus & Fitzgerald 2000, Van Weyenberg et al 2008a) and increases following meal feeding (Cartmill et al 2005). The insulin increase associated with meal feeding appears to drive the postprandial increase in plasma leptin (Cartmill et al 2005). Dexamethasone administration has also been shown to be a potent stimulator of leptin secretion in horses, whereas physiological elevation of cortisol concentrations following ACTH administration produced only minor increases in leptin (Cartmill et al 2005).
Hyperleptinemia, defined as a serum leptin concentration >10–12 ng/ml, has been identified in obese horses and ponies (Cartmill et al 2003, Frank et al 2006). One research group has categorized horses with high BCS (i.e. overweight/obese) by low (<5 ng/ml) and high (>12 ng/ml) resting leptin concentrations (Cartmill et al 2003), and have reported that horses from the high leptin group also have decreased insulin sensitivity and exaggerated insulin responses to glucose infusion when compared to “low leptin” horses of similar body condition (Cartmill et al 2003, Caltabilota et al 2010). The authors hypothesized that the hyperleptinemic condition is a result of reduced insulin sensitivity, associated with a chronic increase in circulating insulin and hence long-term stimulation of adipose tissue output of leptin (Caltabilota et al 2010).
Lack of physical activity
Decreased need and/or opportunity for physical activity are considered to be primary drivers in the still growing epidemic of obesity and associated metabolic disorders in human populations (Booth et al 2008). It is well established that even short-term physical inactivity (or decreased daily ambulatory activity) increases fat mass, reduces lean body mass and impairs systemic as well as skeletal muscle insulin sensitivity in both rodents and humans (Booth et al 2008, Laye et al 2007). Although the role of physical activity in development of obesity in horses and ponies is unknown, it is likely that a lack of exercise – in combination with excess feeding – is a contributing factor.
Disease associations
There is mounting evidence, in humans and other companion animals (dogs, cats), linking obesity with increased risk of multiple chronic diseases including diabetes mellitus, cancer and cardiovascular disease (Kopelman 2000, Laflamme 2011). Studies in humans have indicated that obesity increases all-cause mortality rates (Seidell 2010), while one study in dogs reported that even moderately overweight animals were at greater risk for earlier morbidity. In addition, median lifespan was reduced when compared to lean dogs (Kealy et al 2002). Box 28.1 lists diseases and disorders that have been associated with obesity in horses and ponies.
Box 28.1
Conditions of Horses for Which Risk May Be Increased by Obesity
Obesity has been proposed as a risk factor for laminitis in horses and ponies, especially the pasture-associated form of this condition (Frank et al 2006, Treiber et al 2006). Obesity and/or the regional accumulation of fat deposits (e.g., “cresty neck”) are a feature of the equine metabolic syndrome, a recently described condition that is associated with increased susceptibility to laminitis (Johnson 2002, Frank et al 2010). A recent study in the UK reported that the majority of animals with pasture-associated laminitis were overweight or obese. Moreover, BMI was positively associated with severity of laminitis and negatively associated with outcome (survival) (Menzies-Gow et al 2010). It remains to be established whether obesity directly increases the risk and severity of laminitis (e.g., due to mechanical forces exerted on the lamellar tissue associated with increased load bearing) or whether the increased risk is due to other factors associated with obesity, such as insulin resistance (IR) and inflammation (see Chapter 27 and Box 28.2 for further discussion on risk factors and mechanisms of laminitis).
Box 28.2
Equine Metabolic Syndrome
In human medicine, the metabolic syndrome is a set of diagnostic criteria that identifies individuals at high risk for morbidity associated with IR, primarily type 2 diabetes and cardiovascular disease (Alberti et al 2006). Although the criteria for diagnosis of metabolic syndrome vary, the core components are obesity (especially visceral), IR, dyslipidemia and hypertension. In horses and ponies susceptible to recurrent laminitis, an insulin resistant phenotype resembling the human metabolic syndrome has been described (Johnson 2002, Treiber et al 2006, Bailey et al 2008, Frank et al 2010). Descriptions of this laminitis-predisposed phenotype have varied but have included a clustering of obesity (generalized and/or regional), IR, hyperinsulinemia, hyperleptinemia, mild hypertriglyceridemia and current or historical (e.g., founder lines) evidence of laminitis. Johnson (2002) proposed use of the term equine metabolic syndrome (EMS) to describe horses and ponies with this phenotype, while Treiber et al (2006) coined prelaminitic metabolic syndrome (PLMS) for identification of a set of risk factors in Welsh and Dartmoor ponies that predict increased risk of pasture-associated laminitis.
A diagnosis of EMS is based upon clinical examination and evaluation of basal insulin concentrations and/or glucose and insulin dynamics in response to oral or intravenous glucose challenge (Frank 2011). Affected animals may be hyperinsulinemic at rest (insulin >20 mU/l) or exhibit an exaggerated increase in serum insulin in response to glucose administration. Recently, an oral sugar test has been developed to enable simple assessment of insulin dynamics (Frank 2011). This test involves the oral administration of 15 ml corn syrup per 100 kg BW (about 75 ml for a 500 kg horse, providing 75 g of sugars). Preliminary studies have indicated insulin concentration exceeds 50 mU/l between 60 and 90 min post-dosing in insulin resistant animals whereas serum insulin remains <30 mU/l in healthy horses. Further validation studies are required, however. Dietary restriction and increased physical activity are cornerstones of the management of EMS. For EMS animals that do not respond to conservative management, the administration of levothyroxine sodium has been recommended to promote weight loss and improve insulin sensitivity but responses can be individually variable. The biguanide drug metformin has also been used for treatment of IR but efficacy is uncertain (Tinworth et al 2011).
In horses, as in other species, obesity has been associated with IR (Powell et al 2002, Hoffman et al 2003, Frank et al 2006, Vick et al 2007). In a cross-sectional study of Thoroughbred mares, insulin sensitivity assessed by use of the euglycemic-hyperinsulinemic clamp technique was negatively correlated with BCS and estimated fat mass (Vick et al 2007). Evidence of a cause-and-effect relationship between body condition (fatness) and insulin sensitivity has come from weight gain and loss studies. In Arabian geldings, insulin sensitivity was decreased to one-quarter of the baseline value after a 20% weight gain (with an increase in mean BCS from 6/9 to 8/9; Carter et al 2009a), while weight loss of 10% to 15% in obese ponies resulted in improved glucose tolerance (Van Weyenberg et al 2008b) or mitigation of resting hyperinsulinemia (Dugdale et al 2010). Conversely, Quinn et al (2009)
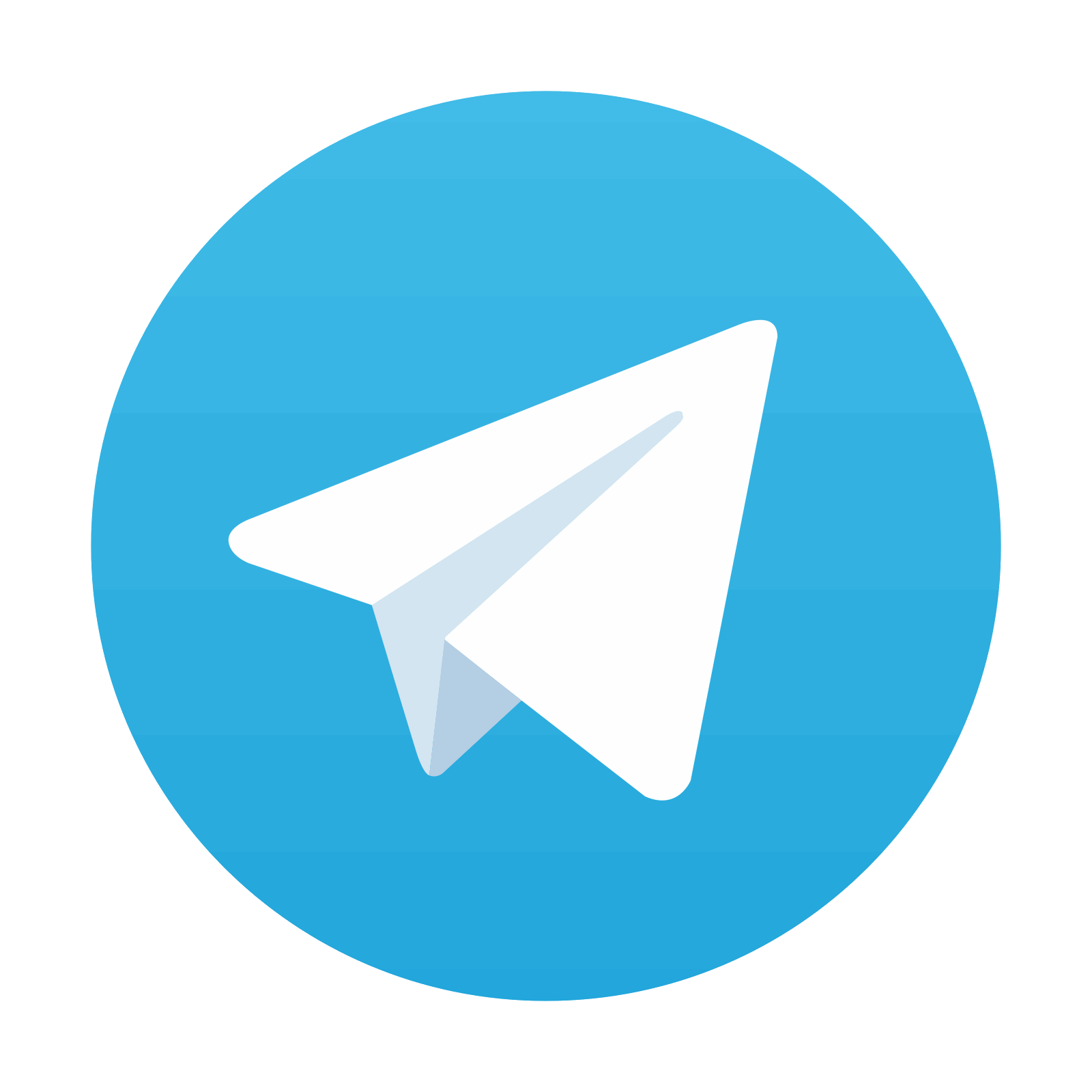
Stay updated, free articles. Join our Telegram channel

Full access? Get Clinical Tree
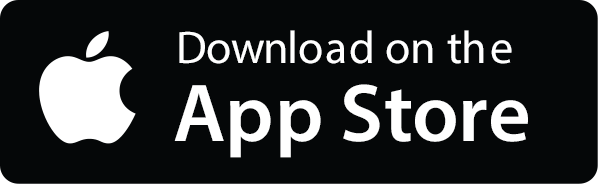
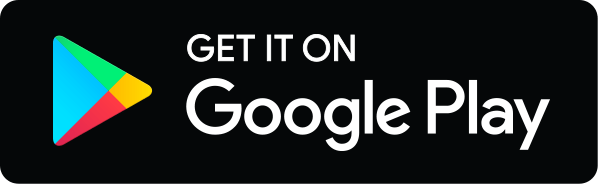