Nutritional Diseases
Reviews of nutritional diseases recognized in South American camelids (SACs) would suggest similar pathologic mechanisms and presentations compared with other species.1–4 Literature citations of specific nutrient deficiency or toxicity diseases of SACs are limited, which is most likely a reflection of underreporting. Although SACs are susceptible to any nutritional disease, certain disease entities seemingly account for the greatest number of disease-related citations, suggesting some unique metabolic characteristics that predispose to these syndromes. Specifically, vitamin D-associated rickets, copper toxicity, and hepatic lipidosis are diseases for which SACs seemingly have greater susceptibility, as indicated by published reports. This chapter will address the commonly encountered nutritional diseases of llamas and alpacas based on literature reports and clinical experience. Some disease entities are further discussed in other system-specific chapters, as indicated.
Macronutrient Diseases
Camelids, like all other animals, are susceptible to either a deficient or an excessive intake of energy and protein relative to requirements, which results in variable stages of malnutrition or obesity, respectively. In general, camelids are quite resilient to the pathologic effects of starvation because of their unique metabolic adaptations (for a complete discussion, refer to Metabolic Diseases, Chapter 41).
Protein Energy Malnutrition
Epidemiology
The most common reason for PEM is poor quality forages coupled with the animal’s inability to consume sufficient amounts relative to requirements.5 Growing animals, females in late pregnancy, and lactating females have the greatest energy and protein requirement and hence are most susceptible to PEM. Under South American conditions, animal body weight (BW) and body condition score (BCS) changes will mimic seasonal forage growth patterns. Camelids gain significant body weight and condition and give birth during the rainy season in concert with high-quality forage availability. During the dry season, animals lose considerable weight and condition and are in various stages of malnutrition (see discussion in Chapter 11). Animals giving birth during the dry season are much more prone to PEM, secondary infections, and parasitic disease problems, which often lead to death.
Llamas and alpacas raised outside of South America are exposed to a greater diversity of environmental conditions ranging from extreme hot and humid environments (Southern United States and Europe) to extremely cold winter conditions (Northern United States and Europe, Canada, and Alaska). Average winter daily temperature in northern North America falls below -5 °F (15°C) and may go down to –31 °F (–35°C).5 These are environmental extremes that llamas and alpacas never experience in their native environment. Hot and humid environments bring challenges such as prevention of heat stress, which is a significant health risk for llamas and alpacas. Cold, wet conditions also have challenges associated with providing effective protective shelter and increased energy intake to compensate for additional maintenance requirements. If animals are wet, mud covered, or exposed to wind chill, then maintenance energy may be increased by as much as 75%. Either environmental extreme may result in a situation of potential PEM with poor-quality forage.
In these cold conditions, camelids will expend additional energy to maintain body temperature. Data from other ruminant species suggest that maintenance energy is increased by 1% for every 33.8°F (1°C) below an animal’s lower critical temperature.6 On the basis of data from sheep and assuming a full fleece, the lower critical temperature (LCT) for llamas and alpacas would be approximately 32°F to 50°F (0°C to 10°C).6 Younger animals have greater surface area relative to body size and thus have greater heat loss and a higher LCT compared with adults.
Clinical Findings
Body weight loss and a decline in BCS are the most common presenting signs.5,7 Growing animals also show a slowing or near-total cessation in gain. Depending on the reproductive status of the animal, delayed puberty, anestrus, irregular estrus, decreased birth weights, and embryonic death may be observed. Lactating females show a marked decline to cessation of milk production. Pregnant and lactating females experiencing PEM may be prone to hepatic lipidosis as a secondary complication.8,9 Time frame and severity of clinical manifestations will be dependent on the degree of dietary energy and protein deficiencies. Animals experiencing PEM maintain a healthy appetite until near terminal stages in contrast to animals afflicted by an infectious or parasitic disease, which have reduced appetites despite their energy deficit.
Diagnosis and Treatment
Routine BCS (refer to Chapter 12 for method) or BW determinations may be used to diagnose potential problems. A thick fleece may easily hide BW and condition changes from view, requiring direct palpation to determine the BCS. Once unexplained BW loss or BCS loss has been identified, a cause needs to be determined. Chronic infectious, parasitic, and dental diseases may induce BW loss and condition loss as in PEM.5 Protein-energy malnutrition is often a secondary process to chronic disease conditions.
Starvation or dietary insufficiency result in mobilization of adipose stores, characterized by an increase in blood nonesterified fatty acid (NEFA) up to about 0.6 to 1.0 milliequals per liter (mEq/L), a mild increase in blood β-hydroxybutyrate up to about 1 to 2 milligrams per deciliter (mg/dL), and little to no change in blood triglycerides.9,10 Hypoalbuminemia and hypoproteinemia are often observed, but total protein concentration as well as other serum chemistry parameters may be variable, depending on underlying or secondary conditions. Anemia, neutropenia, and lymphopenia often are associated with PEM, although altered white blood cell (WBC) number and differential count may reflect underlying chronic infectious or parasitic disease.
Elevation in blood NEFA concentration is often not associated with any clinical signs but may indicate risk. Experimentally, pregnant camelids and lactating camelids on restricted diets may develop more rapid and more severe fat mobilization, possibly resulting in hepatic lipidosis.9 Nonpregnant, nonlactating camelids develop a lower degree of fat mobilization and do not accumulate lipid in their liver from negative energy balance alone. Thus, camelids that do not develop exuberant fat metabolism with dietary caloric insufficiency rarely require any treatment beyond restoration of an appropriate diet. Animals identified early in the disease process can be recovered with appropriate feeding therapy and supportive care (see Chapter 33), although those becoming weak and recumbent have a very poor prognosis even with aggressive therapy.
Prevention
Appropriate feeding regimes where forage quality is matched to nutrient needs of the animal are the cornerstone of PEM prevention. Where forage quality is insufficient, feeding of supplemental feeds is necessary, especially in cold climatic conditions (Table 13-1). In cold environmental conditions, dry matter (DM) intake increases moderately to account for greater energy needs, although this response may not be sufficient under more severe conditions. To achieve such feeding programs, forage quality will need to be evaluated by chemical analysis (refer to Chapter 9, Nutritional Requirements). Routine assessment of animals’ energy status by body weight or condition scoring is a recommended practice, especially for those individuals with higher energy requirements and prior to and during the cold season. Important times to assess BCS would be during early to mid-pregnancy, early to mid-lactation, and periodically (4–6 times per year) to other animals of the herd to assess energy status.11
TABLE 13-1
Intake Amounts of Different Feeds That Would Meet Additional Energy Needs to Support Thermoregulation under Increasing Cold Stress Conditions*
lb/day, Pound per day; Mcal/lb, megacalorie per pound; ME, metabolizable energy.
*The calculations are based on a 2.5 Mcal ME maintenance requirement.
†Higher intake amounts of fat or vegetable oil are not recommended due to potential adverse effects on forestomach microbial populations.
Obesity
Epidemiology
Obesity is considered one of the more prevalent nutritional problems in llamas and alpacas outside of South America.12,13 Consumption of energy in excess of requirement results in fat deposition leading to obesity. Recent work suggests excess protein intake in alpacas may also lead to obesity.14 Ad libitum feeding of high-quality forage or overfeeding additional supplements are primary contributors to obesity. Many commercially available nutritional supplements contain substantial amounts of cereal grains or readily fermentable fiber, which are good energy sources. Even “low energy” supplements may contain unwanted caloric intake given the efficient fiber fermentation process in SACs. The noted discrepancy in feed intake expectations between North and South American data explains the greater obesity issues in camelids in North America. Deleterious effects of obesity include greater susceptibility to heat stress (refer to Chapter 41), metabolic derangements, infertility, and associated locomotion problems.
Treatment and Prevention
For weight reduction to be successful, a methodical approach to limiting energy intake and monitoring response should be instituted. In companion animal species, required energy intake is calculated based on ideal BW, then fractionally reduced to 60% to 65% or 70% to 75% of total in dogs and cats, respectively. In a feed restriction study, llamas that lost 15% to 20% of BW within a 10-to-14 day period were at high risk (50%) for some degree of hepatic lipidosis.9 The unique metabolic adaptations of camelids are similar to those of cats. Cats, unlike dogs, are susceptible to hepatic lipidosis with moderate to severe calorie restriction and rapid weight loss. Thus, the approach for weight reduction in cats might be an appropriate recommendation for weight loss in camelids. Caloric intake is restricted to 70% to 75% of ideal BW requirement to target 0.5% to 1% of weight loss per week in obese animals.
Protein Excess
Renal disease, large birth weights, and increased-diameter fiber have been blamed on excessive dietary protein; however, none of these associations has been proved. A recent study has suggested excessive dietary protein may lead to obesity.14 In this study, dietary protein supplementation did not alter micron size but improved staple length and yield. Additional dietary protein above requirements is cost prohibitive, although more work is necessary to determine the appropriate balance of dietary protein to meet forestomach microbe and animal needs. Of greater concern relative to dietary protein is provision of nonprotein nitrogen (NPN) sources.
Mineral Deficiency Diseases
Macromineral Deficiencies
Epidemiology
In contrast to domestic ruminants and horses, clinical hypocalcemia and hypomagnesemia appear to be rare in camelids, except in New Zealand, where grass tetany and Ryegrass staggers are major differential diagnoses for camelids with staggering gaits (see under Neurologic Diseases, Chapter 38). Both blood abnormalities may occur secondary to hypoproteinemia, which is common in camelids, and more specifically secondary to hypoalbuminemia. However, lack of albumin does not affect the functional, ionized fractions of blood calcium and magnesium, and hence does not induce clinical abnormalities. Phosphorus deficiency is most often associated with vitamin D deficiency (see Vitamin Deficiency discussion below).
Diagnosis and Treatment
As indicated by clinician experiences, hypocalcemia and hypomagnesemia in camelids present with similar clinical signs, diagnostic criteria, and therapeutic response compared with other ruminants.1,2 As with ruminants, diagnosis is based on history, signalment, and response to therapy, with blood analysis used for confirmation of the diagnosis.
Prevention
Hypocalcemia and hypomagnesemia prevention is based on appropriate mineral supplementation and accounting for interfering mineral interrelationships (see Chapter 9). It is assumed that the same mineral interrelationships influencing calcium and magnesium homeostasis in ruminants are applicable to camelids. Excess dietary phosphorus and potassium and inadequate dietary magnesium adversely affects calcium homeostasis. Excess potassium is the primary dietary factor adversely influencing dietary magnesium availability. Although these mineral interrelationships are of concern, the most important factor is ensuring adequate dietary content of calcium and magnesium to meet requirements (refer to Table 9-6, Chapter 9). Once dietary calcium is deemed adequate, phosphorus intake should be evaluated to ensure a dietary calcium-to-phosphorus ratio between 2 : 1 and 1.5 : 1. For magnesium, the primary concern beyond adequate dietary amount is interference from potassium. If dietary potassium content is high, typical of high quality pasture or forages, dietary magnesium content needs to be increased. A suggested dietary potassium-to-magnesium ratio of 4 : 1 is suggested.
Copper Deficiency
Epidemiology
Copper (Cu) deficiency is a concern in ruminant animals because of the unique interaction between molybdenum (Mo), sulfur, and dietary copper that results in reduced availability. Forestomach microbes metabolize dietary molybdenum and sulfates to generate a range of chelating thiomolybdate compounds. Thiomolybdates bind to dietary copper making it unavailable for incorporation into copper-dependent metalloenzymes. Some thiomolybdates are absorbed and bind to copper-containing enzymes reducing their biologic activity. Additionally, excessive dietary zinc and iron may impede dietary copper availability. Copper is usually provided in the forage or mineral supplements of camelids. In many geographic regions, forage copper is low (<4 parts per million [ppm]) or contains high concentrations of substances that interfere with copper absorption. Mineral supplements tend to contain enough or potentially too much copper, but this is also variable.
Clinical Presentation
Clinical signs in domestic ruminants include ill thrift, immunodeficiency, poor fiber quality, anemia, chronic diarrhea, dilute hair color (achromotrichia), and leukoencephalomalacia. Whereas all these signs have been identified in sick camelids, their connection to copper deficiency is difficult to confirm. Two llamas (10 and 23 months of age) with low serum copper concentrations (<0.14 microgram per milliliter [mcg/mL]; deficiency <0.29 mcg/mL) and responding to copper supplementation had presented with anemia and poor condition.15 Other reports had linked copper deficiency to neurologic deficits (hindlimb ataxia, posterior paresis) similar to the disease “swayback” observed in neonatal sheep.1,16,17 Reported serum copper concentrations were not considered deficient and the affected animals did not respond to copper therapy, thus questioning the role of copper in the observed disease process. In one case of a 6-month old llama with ascending paralysis, very low liver ([5 microgram per gram [mcg/g]) and kidney (0 mcg/g) copper concentrations were determined, suggestive of copper deficiency.1
Diagnosis
History and signalment can be suggestive of a copper deficiency disease process, but more supportive evidence must come from determinations of feed and animal copper status. Feed copper concentrations below 4 ppm dry weight are strongly suggestive of deficiency. Values between 4 and 7 ppm dry weight are marginal and may lead to deficiency. Animal copper status is best determined by liver copper concentration, although serum copper concentration may be supportive of a diagnosis. Hepatic copper concentration less than 10 mcg/g dry weight is strongly supportive of a deficiency diagnosis, whereas values between 10 and 90 mcg/g dry weight are marginal and may induce some deficiency disease signs (refer to Chapter 12). Serum copper concentration less than 0.3 microgram per milliliter (mcg/mL) is supportive of a deficient status. Higher serum copper concentrations do not rule out potential deficiency status as serum copper values are not as diagnostic for copper status.
Iron Deficiency
Epidemiology
Iron (Fe) deficiency potentially results either from inadequate intake, which is typical of growing animals on milk-based diets, or from chronic blood loss most typically from parasite infestation.18 Younger animals are more susceptible to iron deficiency disease as a result of their higher requirement, lower intake, and high risk for parasitic disease. Adult herbivores consume a diet that is more than adequate in iron, even accounting for the low availability of dietary iron sources.
Clinical Presentation
Across a range of species, anemia of some form is the clinical manifestation. Three llamas (14–29 months of age) presenting with characteristic microcytic, hypochromic anemia and poor growth were considered to have iron deficiency.19 Serum iron concentrations in these cases were between 20.1 and 59.7 mcg/dL, below established reference range for adult llamas (see Table 12-11, Chapter 12) and the animals responded to iron supplementation.
Treatment and Prevention
Depending on the severity of deficiency, iron supplementation may be implemented with whole blood transfusion or parenteral iron dextran. A safe dosing scheme for camelids using iron dextran has not been established. A conservative approach (150 mg per cria at a 2- to 3-week interval) is recommended, as anaphylactic or more severe toxic reactions may occur. In most cases of iron deficiency, dietary supplementation is all that is necessary. Ferrous sulfate and many calcium-phosphate minerals are readily available iron sources. Most trace mineralized salt products will have sufficient iron if consumed in adequate amounts. In mineral salt products, it must be ensured that the source of iron is not ferric oxide only, which is not available. Most forages and commercial supplements will have sufficient iron content to meet or exceed dietary requirements.
Selenium Deficiency
Epidemiology
Nutritional myodegeneration (i.e., white muscle disease) resulting from clinical selenium deficiency has been reported in dromedary camels.20 Although no published reports of selenium deficiency disease in llamas and alpacas exist, it has been empirically diagnosed and is a disease of concern in many regions of North America, Europe, Australia, and New Zealand.1,2,13,21 Animals of any age may be affected, although younger animals most commonly experience clinical disease. More recently selenium has been associated with iodine function through the actions of selenium-dependent 5′-deiodinase enzymes that convert thyroxine (T4) to the metabolically active triiodothyronine (T3).22 This biologic action might account for selenium’s association with clinical disease manifestations other than myodegeneration.
Clinical Presentation
Severe selenium deficiency results in pathologic degeneration of skeletal muscle fibers with secondary fibrosis.21,22 Affected animals show clinical signs reflective of specific muscles affected and severity of degenerative changes to muscle fibers. Typically, both hind legs are symmetrically affected; however, tongue and heart muscles are commonly involved in newborn or young growing animals. With skeletal muscle damage, affected young or older animals show various degrees of lameness, weakness, or difficulty moving. Sudden death may occur in younger animals with damaged heart muscle. Newborn animals with tongue lesions will have difficulty nursing and may be diagnosed as “dummy” animals. Severe selenium deficiency in pregnant females has been associated with abortion and stillbirth. All of these clinical presentations have been documented in most domesticated species, although overt myopathy is rare in camelids.
Diagnosis
Diagnosis of selenium deficiency may be achieved through assessment of selenium status using serum, whole blood, or hepatic selenium concentrations or of whole blood glutathione peroxidase activity. With respect to blood selenium distribution, llamas and alpacas are different from cattle, sheep, and goats. Llamas and alpacas have a greater amount of selenium-dependent glutathione peroxidase activity in serum, which results in less difference between serum and whole blood selenium concentrations. Deficient whole blood selenium concentrations are defined as <120 nanograms per milliliter (ng/mL) with adequate between 150 and 220 ng/mL for either llamas or alpacas (refer to Chapter 12). Serum selenium concentrations <80 or <110 ng/mL are considered deficient for adult alpacas and llamas, respectively. Criteria defining adequacy and deficiency are variable with age. Expected hepatic selenium concentrations range from 1.0 to 2.5 mcg/g dry weight, similar to expected values for other species. Fetal hepatic selenium concentrations are higher (5.25 mcg/g dry weight) because of the concentrating ability. Hepatic selenium concentrations below 0.4 mcg/g dry weight are considered deficient.
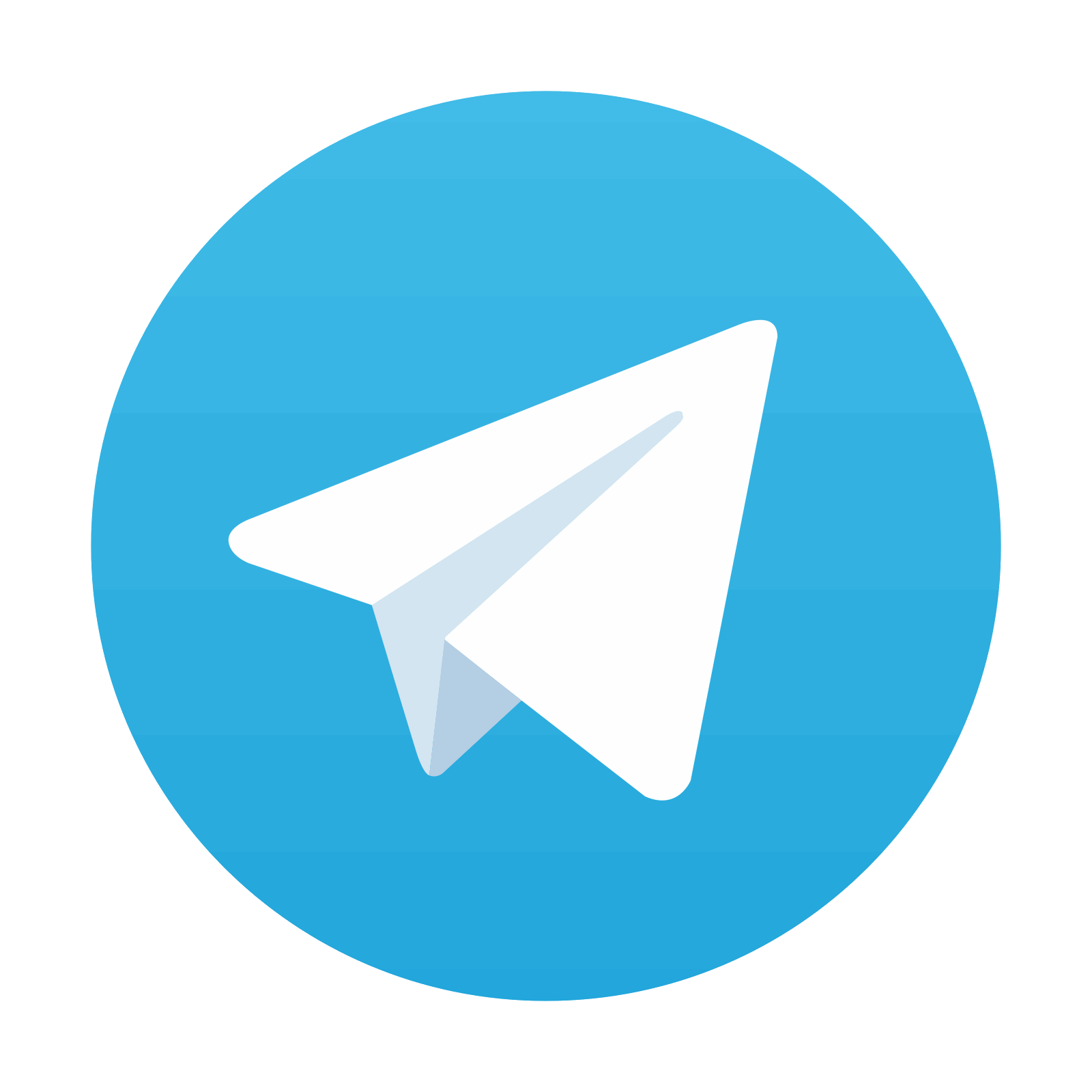
Stay updated, free articles. Join our Telegram channel

Full access? Get Clinical Tree
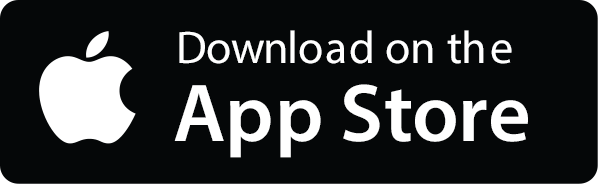
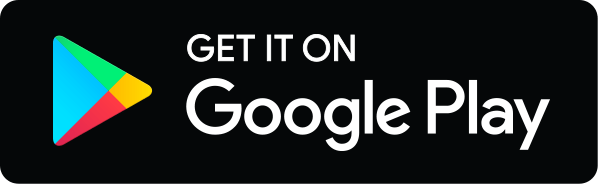