Chapter 30 Nutritional Assessment and Management
Malnutrition
Definition
Used broadly, the term malnutrition describes any nutritional disorder, including obesity caused by overnutrition, unbalanced nutrition as a consequence of one or more nutrient deficiencies, or cachexia caused by undernutrition.1 Although there is no universally accepted definition for malnutrition, the World Health Organization describes it as “the cellular imbalance between supply of nutrients and energy, and the body’s demand for them to ensure growth, maintenance, and specific function.”2 The term undernutrition, which is sometimes used interchangeably with malnutrition, is defined as “a state of energy, protein or other specific nutrient deficiency which produces a measurable change in body function, and is associated with a worse outcome from illness as well as being specifically reversible by nutritional support”.1 The terms malnutrition and undernutrition are used interchangeably in this chapter.
Isolated deficiencies of vitamins, minerals, and trace elements may occur in the absence of protein-energy malnutrition.2 Suboptimal nutrition also occurs, in which an individual is not unhealthy, but will not function at his or her highest level. For example, it has been shown that an overnight preoperative fast in a healthy person results in postoperative insulin resistance and negative nitrogen balance.3 Circumstances may also arise when an individual has an illness or trauma in which a substrate such as glutamate becomes limiting and is, therefore, a conditionally essential nutrient.1
Disease states may be accompanied by a loss of appetite or inability to eat, a decrease in the assimilation of nutrients, or nutrient losses that result in malnutrition. Malnutrition may also occur when an animal is being fed an insufficient amount of food for its energy needs, either because of poor feeding practices or a high energy requirement, such as in working dogs (Fig. 30-1). Feeding a poor-quality diet may result in energy deficiencies or specific nutrient deficiencies, such as thiamine. Animals fed homemade or vegetarian diets are known to be at higher risk for developing subclinical nutritional deficiencies or imbalances.4
Starvation in Healthy Animals
Starvation in an unstressed (i.e., not ill or injured) animal, also called uncomplicated starvation, initially results in utilization of the body’s carbohydrate stores, especially hepatic glycogen. In dogs this reservoir may last for several days, compared with approximately 24 hours in humans.5 Energy consumption then shifts primarily to fat and some protein metabolism. Both skeletal and visceral body proteins are used to provide gluconeogenic precursors, but liver structural proteins are used for gluconeogenesis before skeletal muscle.6 As all body protein is functional, the primary adaptive shift is to fat utilization. Fatty acids are readily oxidized by the kidney and muscle tissues. Fatty acids are also converted to ketones in the liver which may then be used by the central nervous system. Several tissues, for example, adrenal medulla, red blood cells, and brain, have an obligate requirement for glucose, but the brain can adapt to ketone metabolism during periods of starvation. Glycerol resulting from the breakdown of triglycerides can be converted to glucose. Full adaptation to starvation with minimal protein oxidation can take up to 2 weeks in humans. At that time the respiratory quotient (RQ), a determination of the fuels being used by the body, is usually 0.6 to 0.7, indicating fat oxidation primarily.7,8 If uncomplicated starvation lasts for longer than several days, metabolic rate is decreased as a consequence of a loss of tissue mass, a decreased metabolic rate of the remaining tissues, and decreased physical activity.9
Starvation in Ill or Injured Animals
Metabolism of the starved ill or injured animal is more complicated than in simple starvation (Table 30-1). Tumor necrosis factor (TNF) and interleukin-1 produced during injury or illness are associated with stimulation of proteolysis and inhibition of lipoprotein lipase activity. The counterregulatory hormones, cortisol and glucagon, and the catecholamines contribute to this process, eventually leading to insulin resistance and a tendency to develop hyperglycemia. Because of the metabolic shift, protein is catabolized at an increased rate and the adaptation to lipid metabolism is impaired.7,8,10 The use of protein as an energy source often results in a persistent, marked nitrogen loss and net negative nitrogen balance. The increase in urinary nitrogen excretion can be approximately five times that of a patient in uncomplicated starvation. In humans, the RQ of 0.8 during this state indicates mixed fuel consumption, with approximately 30% from amino acids, 40% from glucose, and 30% from fat.7 It is the loss of body protein, not fat loss, that produces the complications of malnutrition during illness or injury.
Table 30-1 Comparison of the Metabolic Changes of Uncomplicated Starvation Versus Stressed Starvation of the Ill or Injured
Metabolic Parameter | Uncomplicated Starvation | Stressed Starvation |
---|---|---|
Inflammatory mediators | ↑ | ↑↑↑ |
Primary energy sources | Glucose, fat | Glucose, amino acids, fat |
Protein synthesis | ↓ | ↓↓ |
Catabolism | ↑ or ↓ | ↑↑↑ |
↑, increased; ↓, decreased.
Consequences of Malnutrition
Wound healing, which involves increased cellular activity and new protein synthesis, is compromised by malnutrition.4 If the loss of lean body mass exceeds 10%, there may be decreased substrates for wound healing.11 Specific micronutrients are also necessary for collagen synthesis and epithelialization, in particular, vitamins C and A, and zinc, copper, and manganese.2 Malnutrition is also a predictor of postoperative complications after human abdominal surgery.12
Malnutrition affects the immune system in many ways, including decreases in the ratio of CD4:CD8 T cells, lymphokine and monokine production, complement C3, opsonic function, intracellular killing capacity of neutrophils, phagocyte dysfunction, antibody affinity, response of acute phase reactants to infectious challenge, and secretory immunoglobulin (Ig) A antibody response.13,14 Seven days of starvation in cats results in immunosuppressive effects, including decreases in leukocyte and lymphocyte numbers.15
Other consequences of malnutrition include fatigue, impaired muscle strength and thermoregulation, and decreased respiratory, pancreatic, gastrointestinal, mental, endocrine, and cardiovascular functions.1 As fat and lean components of the body are depleted, the extracellular fluid compartment stays the same or increases. The ability to excrete water and sodium may be reduced, increasing the tendency to develop edema.1
Morbidity and mortality rates are increased in malnourished patients. Postoperative mortality rates in human cancer patients approach 23% for the malnourished, but are only 4% in those in better nutritional condition.16 Nutritional support in elderly patients reduces the mortality rate from 18.6% to 8.6% at 6 months.17 Emaciated cats are significantly more likely to die within a year than those of optimal body condition.18 Box 30-1 summarizes the consequences of malnutrition.
Assessment of Malnutrition
A number of methods have been used to assess nutritional status in humans, most of which include a combination of medical and dietary history, physical examination, anthropometric measurements, and laboratory data.19 Many of the assessment protocols for humans use the body mass index (BMI), which is a ratio of weight in kilograms and the square of the height in meters, and anthropometric measurements such as mid-arm muscle circumference.16 These methods are not practical in dogs because of extreme variations in body shape and size. A feline version of BMI has been developed for healthy cats and has been validated by dual-energy X-ray absorptiometry for cats weighing between 3 and 9 kg of body weight.20 The feline BMI uses the rib cage circumference in centimeters and the leg index measurement (LIM), which is the hind leg length from the middle of the patella to the dorsal tip of the calcaneal process in centimeters. The amount of body fat is then calculated using the equation:
The degree of weight loss can be difficult to assess if the animal’s previous weight is unknown, and ascites may make weight loss assessment more difficult for the owner. Humans do not give reliable estimates of their own food intake21; the reliability of owners’ assessment of the food intake of their pets has not been established.
Body condition scores (BCSs) provide a semiquantitative method for evaluating fat and lean body tissue percentages.22,24 Scoring systems for dogs and cats have been developed using systems of 1 to 5 points, 1 to 6 points, and 1 to 9 points, with lower numbers representing thinner condition and higher numbers indicating obesity. In both dogs and cats, a BCS using a 9-point scale has shown good correlation (0.92 for dogs and 0.91 for cats) with percentage of body fat as determined by dual-absorption X-ray absorptiometry (DEXA).23,24 This BCS system, using pictures and descriptions, shows good repeatability within and between observers. In dogs, a score of 4 or 5 out of 9 is optimal, depending upon the breed, and in cats a score of 5 is optimal. Unfortunately, the BCS systems do not take into account muscle wasting, and many ill animals may show loss of muscle that is disproportionate to the loss of fat because of the metabolic changes of stressed starvation. Consequently, a muscle mass scoring system has been developed that is based on palpation of skeletal muscle over the skull, scapulae, spine, and pelvis. Animals with no muscle wasting are scored as a 3, those with mild wasting are scored as 2, moderate wasting is scored as a 1, and a 0 score represents severe wasting.25
Multifrequency bioelectrical impedance has been used experimentally to assess body composition in healthy cats,26 but has not yet been used in the clinical setting. Disturbances of water and electrolyte metabolism in the ill patient would limit the usefulness of this technique in the hospital setting.16 Another method used in research settings for determination of body composition is DEXA, which has good accuracy but requires anesthesia and may not be practical for ill animals.27
Nutritional assessment in humans has included measurements of serum albumin and other proteins, including acute-phase reactants. These parameters may be affected by factors other than the metabolic changes of illness, including hydration status and changes in vascular permeability. Furthermore, normal serum albumin concentrations are maintained in starving people without stress, such as humans with anorexia nervosa,16 and a study in elderly humans showed frequent discordance between serum albumin and clinical nutritional assessments. This may mean that changes in serum albumin seen in ill patients are more likely to be caused by the underlying disease.28 Measurement of serum insulin-like growth factor 1 (IGF-1) concentrations in healthy dogs has shown correlation with nutritional restriction,29 but IGF-1 is not yet performed in routine clinical practice and the association with nutritional restriction has not been examined in ill animals. Although there appears to be no reliable laboratory marker for assessment of nutritional status, the identification of specific nutrient deficiencies, such as folate, cobalamin, and iron, can be very useful. Box 30-2 summarizes the nutritional assessment for cats and dogs.
Prevalence of Malnutrition
The prevalence of malnutrition in hospitalized human patients ranges from 13% to 78%.30–32 The prevalence of malnutrition among cats and dogs is not as well documented. In a multicenter study of canine and feline BCSs using a 5-point scale, dogs had a mean BCS of 3.3 ± 0.7 and cats had a mean BCS of 3.2 ± 0.7.33 The most commonly reported score for both species was 3, indicating ideal body weight. In another study of 276 hospitalized dogs that utilized a 9-point scale, the average BCS was 5.0 with a range of 1 to 9, and a normal distribution of scores.34 Another study of small animal patients referred to an internal medicine service using the 9-point scale showed a median BCS for dogs of 6.5, although 20% of the dogs had a less-than-ideal BCS.35 Of dogs, 34.7% had a history of decreased food intake and 45.8% had recent weight loss. Although many of the dogs had a good or even fat BCS, the history of weight loss and poor appetite was consistent with a risk of malnutrition and loss of functional lean body mass. For cats, the median BCS was 4.0, with 53.3% of the cats having a less-than-ideal BCS. Of cats, 53.3% had a decreased appetite and 56.7% had lost weight. In another study of canine cancer patients using a 9-point BCS scale, 55% of dogs were scored at 6 or higher, and only 4% were scored at 3 or less.25 However, using the 3-point muscle-wasting score, 15% had moderate to severe muscle wasting and 20% had mild muscle wasting, consistent with the loss of lean body tissue.25 The latter result emphasizes the importance of determining muscle mass scores in addition to BCSs.
Gastrointestinal Disease and Risk of Malnutrition
Human patients hospitalized with gastrointestinal disorders have among the highest rates of malnutrition.2 The highest prevalence is in those people with inflammatory bowel disease (IBD), liver disease, and pancreatitis. The pathophysiologic mechanisms are manifold and include decreased intake, maldigestion, malabsorption, inflammation, increased nutritional requirements, and drug–nutrient interactions. Decreased appetite and reduced intake are obvious findings in many of the canine and feline gastrointestinal, pancreatic, and hepatobiliary disorders.
In humans it is noted that cobalamin is often deficient in cases of atrophic gastritis and disorders of the terminal ileum, and iron, folate, and magnesium deficiencies are common in patients with primary enteropathies.16 Similarly, decreased cobalamin and folate have been reported in cats with gastrointestinal diseases, including IBD, intestinal lymphoma, pancreatic inflammation, and cholangitis.36,37 Iron-deficiency anemia may develop as a consequence of IBD in the dog.38
Reduced food intake and intestinal malabsorption may be present with many primary enteric diseases or secondary to cholestatic liver disease.39 Fat malabsorption may result in deficiencies of fat-soluble vitamins A, D, E, and K, and zinc.6,40 Pancreatic insufficiency classically presents with signs of malnutrition caused by maldigestion and decreased nutrient absorption. The reader is referred to Chapter 32 for more information on nutritional requirements in gastrointestinal, pancreatic, and hepatobiliary disorders.
Contribution of Hospital Practices to Worsening Nutritional Status
Ten percent to 60% of human patients lose weight during hospitalization.16 Inadequate intake or fasting prior to anesthesia or diagnostic procedures may contribute to this weight loss. In one study, 20% of meals were missed, often because of fasting for investigative or therapeutic procedures.41 In another study, estimated nutrient requirements were met in only 50% of patients because of inappropriate feeding instructions and failure to deliver prescribed nutrients.42
In a multicenter canine study it was found that a positive energy balance (>95% of calculated resting energy requirements) was achieved in only 220 (27%) of 821 “dog-days.”34 Reasons cited for patient negative-energy balance included poorly written feeding orders (22%), orders to withhold feed (34%), and, in many dogs (43%), a refusal to eat offered food. Anorexia in ill animals may arise as a consequence of the effects of cytokines such as pain and drug therapy. The reader is referred to Chapter 7 for more information on the mechanisms and management of anorexia.
In a study of enteral feeding prescribed by a nutrition support service, patients received 91% of their prescribed feedings.43 Animals in this study received more than two-thirds of their goal calories on only 76 of 104 days, which may have resulted from continuous tube feedings rather than intermittent bolus feedings. The interruption of continuous feedings for procedures may have resulted in a smaller total intake than the use of intermittent bolus feedings.43 Box 30-3 summarizes some of the causes of worsening nutritional status in hospitalized patients.
Box 30-3
Contributing Causes to Worsening Patient’s Nutritional Status within the Hospital
Hypermetabolism causing increased calorie requirements (uncommon)
Lack of nutritional status assessment
Delayed initiation of nutritional support
Inappropriate diet, e.g., too low in calories or unpalatable
Poorly written feeding orders or no feeding orders
Incorrect calculation of caloric requirements
Interruption of continuous feeding for procedures or to take animal out of cage
Management of Malnutrition
Management of malnutrition starts with appropriate assessment of the patient’s nutritional status, including dietary history. Impairment of organ function occurs with starvation long before significant physical changes are observed, and nutritional deprivation is especially likely to be overlooked in the obese patient during hospitalization.16 Initiation of nutritional support is indicated in patients meeting the criteria of acute weight loss of 5%; chronic weight loss of greater than 10%; anorexia for more than 3 days; or indications of decreased protein intake or malabsorption, poor body condition, weakness, or nonhealing wounds. Daily monitoring of the patient’s nutritional status while hospitalized helps to ensure adequate intake. Patients should be weighed daily, although day-to-day fluctuations in weight may be greatly influenced by fluid balance. Energy requirements change as a consequence of illness, although in most patients requirements are not increased above resting energy requirements.16
Nutritional support in the starving and malnourished patient may induce a shift from the catabolic to the anabolic state. In the refeeding of a severely malnourished patient, a metabolic shift from endogenous to exogenous energy sources, particularly carbohydrates, may cause depletions in serum concentrations of phosphate, magnesium, and potassium, which move intracellularly under the influence of insulin. This is described as the refeeding syndrome,44 and may be seen with either parenteral or enteral nutritional support. Hypophosphatemia resulting in hemolytic anemia has been described in cats during the provision of enteral nutrition.45 Eight of these nine cats were in poor body condition prior to feeding, and the ninth, which was an obese cat, had a history of anorexia. Initiating feeding at less-than-resting energy requirements may help prevent refeeding syndrome. In another case of feline refeeding syndrome, the phosphorus, potassium, and magnesium were all deficient, and the cat showed concurrent cardiac abnormalities,46 which have also been reported in human refeeding syndrome.44
Hypophosphatemia and red blood cell abnormalities have also been reported in dogs during feeding after nutrient deprivation.44 Phosphorus should be monitored daily for the first week of nutritional support in malnourished animals. Decreased serum potassium and magnesium and hyperglycemia all play a role in refeeding syndrome and should be closely monitored. As thiamine plays a role in carbohydrate metabolism, and it may be depleted during starvation, provision of thiamine supplementation prior to nutrient supplementation has been recommended.44
Appropriate nutritional support should be initiated early in patients, following rehydration and stabilization, and ideally within 24 hours of hospitalization. Initial feeding protocols may be formulated at less-than-resting energy requirements, as even small amounts of nutritional support reverse the catabolism of endogenous protein for energy.47
Monitoring of the patient’s food intake is important, as often records only note that “the patient ate,” and do not describe the quantity or the type of food eaten. Supplemental nutritional support may be provided orally, enterally via feeding tubes, or parenterally via either central or peripheral vein. Enteral feeding stimulates the gut-associated immune system and provides nutrients such as glutamine to the intestinal mucosa.48 Enteral nutrition is also generally safer and more economical than parenteral nutrition. The reader is referred to Chapter 33 for further information about enteral and parenteral nutritional support.
Obesity
Definition and Prevalence
Definition
Obesity is defined as a disease where excess body fat has accumulated such that health may be adversely affected.1 BMI is most commonly used to quantify human adiposity and recent epidemiologic studies suggest that the optimal BMI for nonsmoking adult whites is 20 to 25.1 Additionally, epidemiologic data confirm that disease and mortality risk progressively increases for people classified as “overweight” (BMI 25 to 30), “obese” (BMI 30 to 40), and morbidly obese (BMI >40).2 Companion animals are currently classified as overweight when their body weight is more than 15% above their “optimal body weight,” and classified as “obese” when their body weight exceeds 20% to 30% of optimal3; data suggest that, like in humans, adverse consequences develop when dogs and cats are not maintained in optimal body condition.3–6
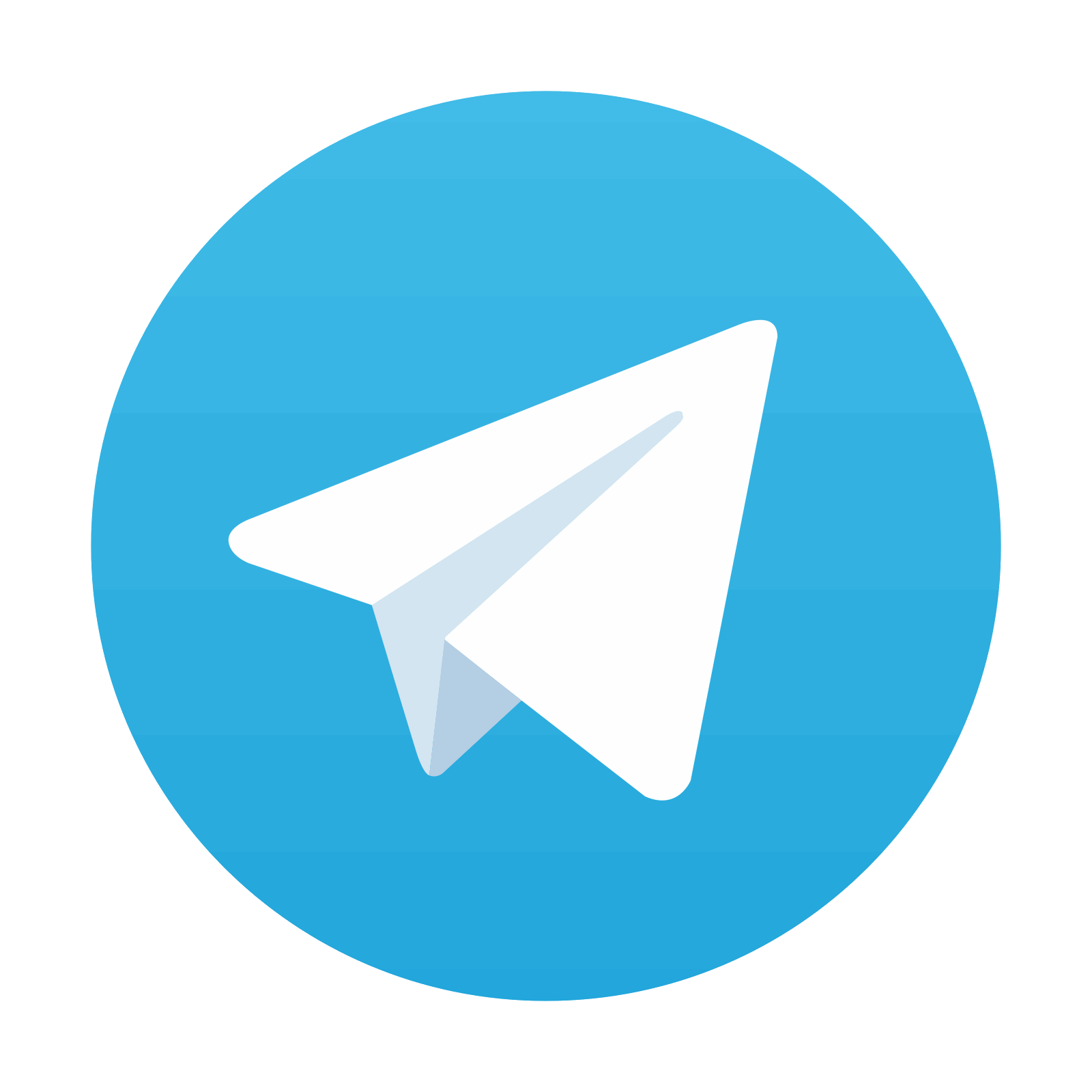
Stay updated, free articles. Join our Telegram channel

Full access? Get Clinical Tree
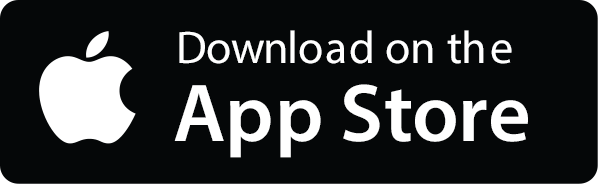
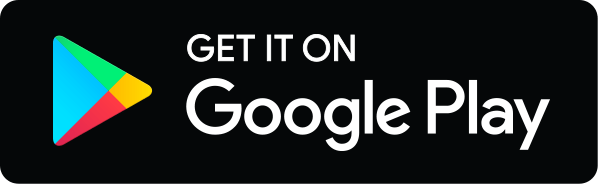