Chapter 3 Neurohistology, physiology and supporting structures
Neurohistology
Key points
Neurons are excitable cells that receive and integrate afferent information from receptors and other neurons, and transmit information to other neurons or effector organs.
Nissl substance (rough endoplasmic reticulum) is a prominent feature of many healthy, neurons. The majority of neurons lack centrioles and cannot divide.
Dendrites receive and integrate multiple synaptic inputs. Dendritic spines are specifically involved in learning and memory; they serve to increase the number of possible contacts between neurons.
Axons are long, non-tapering cytoplasmic processes that transmit efferent impulses; they contain organelles and a cytoskeletal transport system.
Neuroglia provide support and nutrition, maintain homeostasis, form myelin, and participate in signal transmission in the nervous system. They include astrocytes, oligodendrocytes and microglia in the CNS. Schwann cells form myelin in the PNS.
Synapses are the junction between the axon terminals of a neuron and the receiving cell. The majority are categorised as chemical synapses, however electrical synapses also occur.
Cells of the nervous system
Neurons
The soma (plural, somata) contains a relatively large, round nucleus with a prominent nucleolus (Fig. 3.1A). The size of the cell body ranges from 5 µm to more than 100 µm, for interneurons and motor neurons innervating striated muscle, respectively. The soma contains a cytoskeleton made up of neurofilaments and neurotubules. The cytoskeleton extends into the dendrites and axon, setting the diameter of, and providing internal support for, these slender processes.
The soma contains most of the synthetic machinery of the cell, such as the Golgi apparatus and endoplasmic reticulum, while mitochondria are located in both the soma and axons. Nissl substance is granular material that stains with basophilic dyes; it is composed of rough endoplasmic reticulum. Nissl substance is the site of protein synthesis and its prominence in neurons indicates that they are highly metabolically active. In neurons that are damaged, especially after axonal injury, the Nissl granules are dispersed; this reaction is called chromatolysis (Fig. 3.1B).
1. Sensory neurons carry information from the sense organs to the neuraxis. They interface with many different types of receptor cells.
2. Motor neurons carry information from the neuraxis to somatic and visceral muscles.
3. Interneurons have short axons and connect between neurons (Fig. 3.2).
Neuroglia
Astrocytes (Astro – Gk = star shaped) are a heterogeneous population of cells. Morphologically distinct examples of astrocytes include the protoplasmic astrocytes of the grey matter, fibrous astrocytes of the white matter and radial astrocytes of the retina and cerebellum. Most astrocytes express glial fibrillary acidic protein (GFAP) that is used as a cell-specific marker, histologically (Fig. 3.3). Astrocytes perform many functions, including contributing to the blood–brain barrier, regulation of blood flow, provision of nutrients to the nervous tissue, insulating synapses and maintenance of extracellular ion balance. Astrocytes have important roles in the repair and scarring process of the neuraxis following traumatic injuries or inflammatory disease. Bidirectional communication between astrocytes and neurons occurs, and their role in disease processes is increasingly being recognised.
Oligodendrocytes (oligo – Gk = few branches) produce the myelin sheaths that surround many axons in the CNS. Schwann cells myelinate the axons in the PNS (Fig. 3.4). A single oligodendrocyte can myelinate one large diameter axon or up to 100 small-diameter axons, whereas Schwann cells only myelinate single axons. Myelin is essential for rapid, targeted conduction of nerve impulses. The myelin sheath is formed by outgrowth of cell processes from the myelinating cell, spiralling around the axon to form the numerous layers of membranes. Adjacent segments of myelin are called internodes. Internodes are separated by 1-µm gaps called the node of Ranvier. Ion channels clustered in the axonal membrane at the node are used to propagate action potentials along the axon. The myelin sheath is lipid-rich, providing effective insulation and blocking the exchange of ions across the axonal membrane in the internodal regions. Thus the action potential skips over the internodal areas as it jumps between nodes. This is saltatory conduction (saltus – L = to leap or bound). The myelin sheath also physically protects the axon. Its loss renders the axon vulnerable to chemical and mechanical damage.
Microglia are the resident macrophages of the CNS, and thus act as the first and main form of active immunity. Microglia comprise 20% of the total glial cell population within the brain and are constantly surveying the neuraxis for damaged neurons, plaques, and infectious agents. The brain and spinal cord are considered immune-privileged organs in that they are separated from the rest of the body by the blood–brain barrier (BBB) (see Fig. 3.19). This barrier prevents many pathogens from reaching the nervous tissue, but it also blocks most antibodies from accessing the neuraxis, due to their large size. Hence, microglia must be able to recognize foreign bodies, phagocytose them and act as antigen-presenting cells to activate other immune cells.
Neurophysiology
Assoc. Prof Craig Johnson, IVABS, Massey University
Key points
Myelination increases the speed at which impulses are propagated along the myelinated fibre by enabling saltatory conduction.
Action potentials trigger the release of neurotransmitter at a chemical synapse or neuromuscular junction. The neurotransmitter crosses the synaptic cleft and binds to receptors on the postsynaptic membrane causing chemically-gated ion channels to open. Ion transfer causes change in the postsynaptic membrane potential.
Neurotransmitters are classed as excitatory or inhibitory depending on whether they depolarise or hyperpolarise the postsynaptic potential.
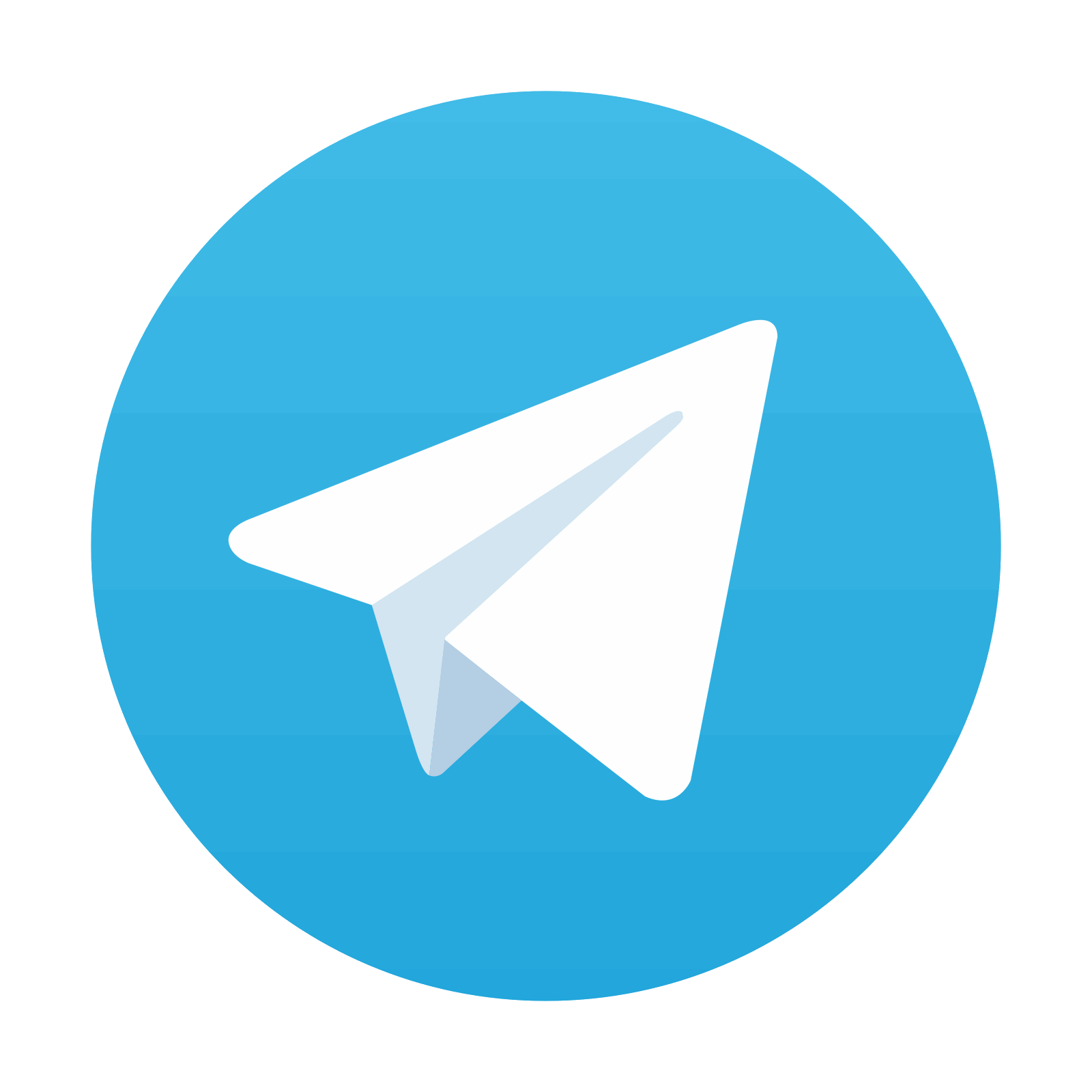
Stay updated, free articles. Join our Telegram channel

Full access? Get Clinical Tree
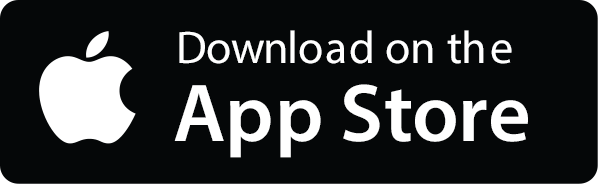
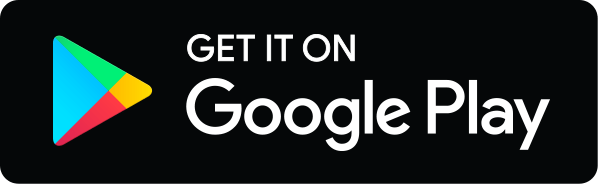