1. Neurohumoral mechanisms regulate blood pressure and blood volume to ensure adequate blood flow for all body organs. 2. The autonomic nervous system affects the cardiovascular system through the release of epinephrine, norepinephrine, and acetylcholine. 3. The arterial baroreceptor reflex regulates arterial blood pressure. 4. The atrial volume receptor reflex regulates blood volume and helps to stabilize blood pressure. 5. The cardiovascular state of conscious subjects is determined by an ongoing and ever-changing mixture of reflex effects and psychogenic responses. The influences of the nervous system and hormones on the cardiovascular system are referred to collectively as the neurohumoral mechanisms of cardiovascular control. The neurohumoral mechanisms are also called extrinsic control mechanisms because they act on organs from the outside. As described in Chapter 24, the mechanisms of cardiovascular control that act locally, within individual tissues, are referred to as intrinsic control mechanisms. The local, or intrinsic, mechanisms predominate over extrinsic mechanisms in the control of blood flow to the “critical” organs, which include the heart (i.e., coronary circulation), brain, and working (exercising) skeletal muscle. In contrast, neurohumoral, or extrinsic, control mechanisms predominate over the intrinsic mechanisms in the control of blood flow to the “noncritical” organs, which include the kidneys, the splanchnic organs, and resting skeletal muscle. The noncritical organs are those that can withstand temporary reductions in blood flow (and metabolism) to make extra blood flow available for the critical organs, whose optimal function on a moment-to-moment basis may be necessary for survival (e.g., in a life-threatening situation involving “fight or flight”). The autonomic nervous system is the “neuro” arm of neurohumoral control. Sympathetic and parasympathetic neurons influence the cardiovascular system through the release of the neurotransmitters norepinephrine and acetylcholine. In addition, sympathetic nerves affect the cardiovascular system by stimulating the release of epinephrine and norepinephrine from the adrenal medulla. The adrenal secretions enter the bloodstream as hormones and circulate throughout the body. Chapter 13 contains additional, basic information about the autonomic nervous system. Table 25-1 summarizes the main cardiovascular consequences of the activation of adrenergic and cholinergic receptors. α-Adrenergic receptors (both α1 and α2) are located in the cell membranes of the smooth muscle cells of the arterioles in all organs and in the smooth muscle cells of the abdominal veins. These adrenergic receptors are innervated by postganglionic sympathetic neurons, which release the neurotransmitter norepinephrine. Circulating epinephrine or norepinephrine can also activate the adrenergic receptors. Activation of these α-adrenergic receptors leads to constriction of the arterioles or the veins. TABLE 25-1 Receptors Involved in Autonomic Control of the Cardiovascular System Sympathetic control of the heart is exerted through the β1-adrenergic receptors, which are found on every cardiac muscle cell. These beta receptors are activated by norepinephrine or epinephrine. Chapters 19 and 21 discuss the effects of activation of the cardiac β-adrenergic receptors. In brief, pacemaker rate increases, cell-to-cell conduction velocity increases, and refractory period decreases. In addition, contractility is increased, so the cardiac contractions are quicker and stronger. The overall effect is increased heart rate and increased stroke volume.
Neural and Hormonal Control of Blood Pressure and Blood Volume
Neurohumoral Mechanisms Regulate Blood Pressure and Blood Volume to Ensure Adequate Blood Flow for All Body Organs
The Autonomic Nervous System Affects the Cardiovascular System Through the Release of Epinephrine, Norepinephrine, and Acetylcholine
Receptor Type
Location
Usual Activator
Effect of Activation
Function
α Adrenergic
α1 and α2
Arterioles (all organs)
Norepinephrine from sympathetic neurons, or circulating epinephrine and norepinephrine
Vasoconstriction
Decreases blood flow to organs; increases total peripheral resistance (major effect)
Veins (abdominal organs)
Norepinephrine from sympathetic neurons, or circulating epinephrine and norepinephrine
Venoconstriction
Displaces venous blood toward heart
β Adrenergic
β1
Heart (all cardiac muscle cells)
Norepinephrine from sympathetic neurons, or circulating epinephrine and norepinephrine
Increased pacemaker rate; faster speed of conduction; decreased refractory period; quicker, stronger contractions
Increases heart rate, stroke volume, and cardiac output (major effects)
β2
Arterioles (coronary and skeletal muscle)
Circulating epinephrine and norepinephrine
[β2 receptors not innervated]
Vasodilation
Increases coronary blood flow; increases skeletal muscle blood flow
Muscarinic Cholinergic
M2
Heart (all cardiac muscle cells, but sparse direct innervation of ventricular muscle cells)
Acetylcholine from parasympathetic neurons
Opposite of β1
Decreases heart rate and cardiac output (major effect)
Sympathetic nerve endings at ventricular muscle cells
Acetylcholine from parasympathetic neurons
Inhibition of norepinephrine release from sympathetic neurons
Decreases magnitude of sympathetic effects on ventricular muscle cells
M3
Arterioles (coronary)
Acetylcholine from parasympathetic neurons
Vasodilation (mediated via nitric oxide)
Increases coronary blood flow (minor effect)
Arterioles (genitals)
Acetylcholine from parasympathetic neurons
Vasodilation (mediated via nitric oxide)
Causes engorgement and erection
Arterioles (skeletal muscle)
Acetylcholine from specialized sympathetic neurons
Vasodilation (mediated via nitric oxide)
Increases muscle blood flow (in anticipation of exercise)
Arterioles (most other organs)
[Receptors not innervated; normal activator unknown]
Vasodilation (mediated via nitric oxide)
Function unknown
Stay updated, free articles. Join our Telegram channel

Full access? Get Clinical Tree
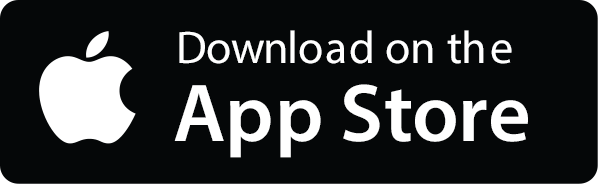
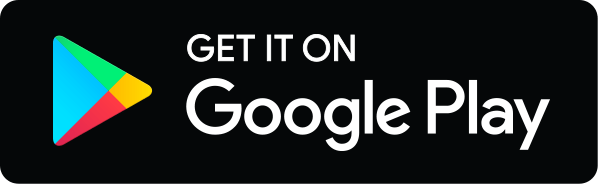