Chapter 58 Large Intestine
Structure and Function
Structure
Macroscopic Anatomy
The large intestine consists of the cecum, colon, rectum, and anal canal (Figure 58-1). In dogs and cats, the ileum communicates directly with the colon, and what is referred to as the cecum in the dog and cat is actually a diverticulum of the proximal colon. The colon is further compartmentalized into ascending, transverse, and descending portions, each segment having slightly different functions and properties. The right colic or hepatic flexure separates the ascending and transverse colon, and the left colic or splenic flexure separates the transverse and descending colon. In dogs and cats, the large intestine contributes 20% to 25% of the total (small and large) intestinal length.1,2
Microscopic Anatomy
As with the small intestine, the cross-sectional structure of the large intestine consists of four distinct layers, that is, mucosa, submucosa, muscularis, and serosa (Figure 58-2). The large intestine differs from the small intestine in the following important ways: villi are absent in the large intestine; the microvilli of the large intestine epithelial cells are much less abundant; goblet cells are more prominent in the large intestine; endocrine cells are less prominent in the large intestine; and crypt-to-epithelial migration is a much slower process in the large intestine.
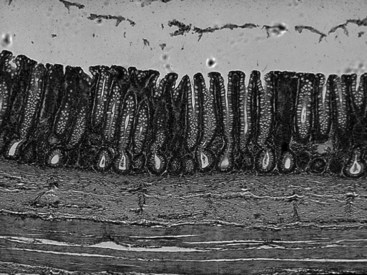
Figure 58-2 Microscopic anatomy of the canine colon.
(Reprinted with permission from Washabau RJ: Diseases of the large intestine. In: Ettinger SJ, Feldman ED, editors: Textbook of Veterinary Internal Medicine, ed 6, Philadelphia, 2005, Saunders, p 1379.)
The mucosa of the large intestine is a flat absorptive surface area differing from the small intestine in that villi are not present. However, numerous straight tubular glands (400 to 600 µm) are present in parallel cylinders and they extend from the muscularis mucosa to the mucosal surface.3 The glands are lined by a continuous sheet of columnar epithelial cells, which are separated from the mesenchymal tissue of the lamina propria by a well-defined basement membrane. The epithelium in the lower half of the crypts is composed of proliferating undifferentiated columnar cells, mucus-secreting goblet cells, and at least three types of endocrine epithelial cells.4 Cellular proliferation is predominantly in the lower part of the crypts in both dogs and cats. The epithelium of the upper half of the crypts consists of differentiating columnar cells, goblet cells, and a few endocrine cells. The flat absorptive surface is lined by many columnar cells as well as a moderate number of goblet cells (10 to 25 goblet cells per 100 epithelial cells),3,5 most of which are largely depleted of their mucous granules. Intraepithelial lymphocytes are relatively sparsely distributed throughout the epithelium (one to seven lymphocytes per 100 epithelial cells),3,5 and as in the small intestine, the predominant T cell subset is the cytotoxic-suppressor (CD8+) type.6,7 The cellular elements of the lamina propria of the large intestine resemble closely those found in the small intestine and include lymphocytes, many plasma cells, mast cells, macrophages, eosinophils, enteric neurons, and fibroblasts.
The muscularis is composed of an inner circular muscular layer forming a tight spiral circumferentially along the course of the colon and an incomplete outer longitudinal muscle layer. The ganglion cells of the myenteric plexus of Auerbach are found between the circular and longitudinal muscle layers. Unmyelinated postganglionic fibers are also found in the circular muscle layer and communicate with the submucosal (Meissner) plexus. The interstitial cells of Cajal, which are located on the submucosal surface of the circular smooth muscle, play a dual role as pacemaker cells and as mediators of neuromuscular transmission in the colon.8–10
Several classification systems for colonic mucosal architecture and cellularity have been proposed (see Chapter 29).1,3,11 It should be emphasized that there are important age,12 site,1,3 diet,13–16 and procedure-related3 differences in the cellularity and architecture of the colonic mucosa, and these differences must be taken into account when interpreting colonic histology. For example, the protein and fiber content of the diet have significant effects on colonic mucosal morphology (e.g., crypt depth and cellularity).14–16 The pathologist should always take these factors into account when interpreting colonic biopsy specimens. The method of biopsy also influences the architecture and cellularity of the mucosa. Compared to full-thickness biopsies, gland length is 25% to 30% shorter and goblet cell numbers are 70% to 75% less in endoscopic biopsies from the same animals. The shallow depth of the endoscopic biopsy apparently causes glandular collapse, and enema or cathartic preparative solutions are believed to cause discharge of mucous goblets.3,11
Function
Mucus Secretion
A lubricant layer of mucus forms a crucial physiologic barrier between the colonic mucosa and the luminal environment. Mucus is a constantly changing mix of secretions and exfoliated epithelial cells, the chief determinants of which are high-molecular-weight glycoproteins or mucins.17 Gastrointestinal mucins are secreted from goblet cells as they ascend from their origin in the crypts up to the colonic epithelium. Mucin secretion is dependent upon the close integration of the cystic fibrosis transmembrane regulator (CFTR), chloride secretion, and granule exocytosis. In addition to their physiologic role as a mucosal barrier, mucins may also have a pathologic role in the metastases of epithelial tumors and enhanced susceptibility to infection.
Water Absorption
In health, approximately 2.7 L of fluid (oral intake, saliva, gastric fluid, bile, pancreatic fluid, and intestinal secretions) is presented each day to the small intestine of a 20-kg dog. Approximately 1.35 L is absorbed in the jejunum, 1.0 L in the ileum, and 315 mL in the colon, leaving 35 mL in feces.18 Thus, the jejunum absorbs 50%, the ileum 75%, and the colon 90% of fluid volume presented to it (Figure 58-3). This fluid absorptive capacity of the colon is largely determined by basal electrolyte (primarily sodium) transport and by the ability of several agonists (aldosterone and glucocorticoids) to augment electrogenic and electroneutral Na absorption. As long as ileocecal flow is less than the colonic absorptive capacity, significant alterations in small intestinal fluid movement may be present, but colonic absorption will prevent the development of diarrhea. On the other hand, when small intestinal absorption and ileocecal flow are normal, a relatively small decrease in colonic absorption (inflammatory bowel disease) will produce significant increases in fecal water output.
Electrolyte Transport
The large intestine regulates the electrolyte and water composition of the feces. There are distinct differences in the mechanisms of electrolyte transport between the ascending and descending colon,19,20 but in general the canine and feline colon absorbs water, sodium, and chloride while secreting potassium and bicarbonate.
In many ways, the mechanisms of absorption and secretion in the large intestine are similar to those of the small intestine, but there are several important differences (Table 58-1). Active nutrient (glucose, amino acid, monoglyceride) transport is prominent in the small intestine, but there is no evidence of active glucose or amino acid absorption in the colon except during the early neonatal period. Sodium transport also differs in the colon. Glucose- and amino-acid–stimulated sodium transport is a well-established property of the small intestine, so much so that oral glucose-electrolyte solutions have been used to reduce the morbidity of cholera and other infectious diarrheal diseases of the small intestine. In contrast, glucose-coupled sodium transport does not take place in the colon and glucose-electrolyte solutions are of no benefit in diarrheal diseases of the large intestine. Sodium transport in the colon instead relies upon electrogenic transport.20,21 The large intestine also differs in its response to mineralocorticoids. Aldosterone markedly increases sodium transport in the colon, but it has only a modest effect in the small intestine. Net sodium absorption ceases in the colon only when the luminal sodium concentration is reduced below 30 to 50 mEq/L, whereas net sodium absorption in the jejunum occurs only at luminal sodium concentrations above 130 mEq/L.21
Table 58-1 Special Features of Colonic Electrolyte Transport
Small Intestine | Large Intestine | |
---|---|---|
Water | Yes | Yes |
Amino acid + glucose absorption | Yes | No |
Lipid absorption | Yes | No |
Glucose-stimulated Na absorption | Yes | No |
Electrogenic Na absorption | No | Yes |
Mineralocorticoid-stimulated Na absorption | Negligible | Significant |
The descending colon possesses both potassium absorptive and potassium secretory processes that are regulated by luminally and hormonally mediated mechanisms, and the overall net potassium movement represents the balance of these oppositely directed transport mechanisms.20,21 The colon, therefore, has the potential to serve as an important regulatory system for the maintenance of overall potassium balance. Although renal potassium transport is critical in the overall control of potassium balance, the colon also contributes to the maintenance of potassium balance by modifying both potassium secretion and absorption, especially when kidney function is impaired.
Immune Surveillance
The colon contains a diverse array of immune cells, including T and B lymphocytes, plasma cells, macrophages, dendritic cells, antigen-presenting cells, mast cells, eosinophils, and neutrophils.3,5–7,22–25 Immune cells are found in the epithelium, lamina propria, and submucosa of the colon. As in the small intestine, appropriate interactions between these different cell types are essential in generating either immune responsiveness or tolerance to the large array of luminal antigens.26,27 Much less is known about colonic immunity, but some generalizations may be made. In the colon, CD8+ T cells are found primarily in the epithelium; very few CD8+ T cells are found in the lamina propria or submucosa. Most intraepithelial lymphocytes are CD3+/CD8+, a phenotype consistent with suppressor-cytotoxic functions. Lamina propria T cells on the other hand are predominantly of the CD4+ helper phenotype. Immunoglobulin (Ig) A-containing plasma cells are more prominent than IgG- or IgM-containing plasma cells in the lamina propria. Thus, in the normal colonic mucosa, a balance would appear to be maintained between helper and suppressor T-cell populations, which allows specific antigen responsiveness while avoiding hyperreactivity.5,6,28 There are many similarities in the immune systems at both sites, but there are important differences in the immune response of the small and large intestine.
Motility
The colon has evolved to serve two important functions: extraction of water and electrolytes from the luminal contents in the ascending and transverse colon, and control of defecation in the descending colon. This specialization of function is attributed to regional differences in colonic motility patterns.29–33 Electrical slow-wave frequency and rhythmic phasic contractions are slower in the proximal portion of the colon, thus facilitating extraction of water from the fecal mass by diffusion and active transport. Retrograde giant contractions (RGCs) and antiperistalsis further facilitate the mixing of contents in the proximal colon. In contrast to that of the proximal colon, motility of the distal colon is characterized primarily by migrating spike bursts and powerful giant migrating contractions (GMCs) that propagate the fecal mass toward the rectum.4,33
Colonic smooth muscle generates at least four different types of contractions to perform the complex motility functions of mixing and propulsion: (a) tone, (b) rhythmic phasic contractions (RPCs), (c) RGCs, and (d) GMCs. The time course, frequency, and force generated by each of these contractions are significantly different from each other.30–33 The precise role of tone in circular muscle cells is not known, but the resulting decrease in the diameter of the colon may enhance the efficiency of the phasic contractions in mixing and propulsion. Tone is normally of small to moderate force and can last for prolonged periods of time (several minutes to hours). RPCs produce mixing and net slow distal propulsion of luminal contents in the postprandial and fasting state. Phasic contractions occur rhythmically at a few cycles per minute, last for approximately 3 to 5 seconds, and generate a moderate force (75 to 100 g). RGCs occur infrequently, last for approximately 20 seconds, generate a very strong force (>150 g), and are propagated from their point of origin into the ascending colon. RGCs facilitate mixing in the ascending colon. The GMCs are of a similar magnitude and frequency to the RGCs, but they generate mass movements from their point of origin to the anorectal junction. It has been suggested that different signal transduction pathways are used by smooth muscle cells to trigger different contractions.34,35 It is remarkable that the same smooth muscle cells can generate so many different types of contractions using a limited number of second messengers.
Bacterial Fermentation
The colon contains the largest concentration of bacteria in the gastrointestinal tract, up to 1011 organisms per gram of feces. The colonic microflora play an important role in the nutrition of the animal primarily via the production of short-chain fatty acids (SCFAs).36 Major fiber fermentation substrates include cellulose, hemicellulose, and pectin, substrates that typically are not digested by pancreatic or intestinal amylases. Acetate, propionate, and butyrate account for more than 85% of formed SCFAs, and they accumulate in concentrations up to 150 mmol/L in the colon of dogs.37 SCFAs are rapidly absorbed by the colonic mucosa, are readily metabolized by colonic epithelial cells, and have various physiologic effects. Among their physiologic effects, SCFAs promote differentiation and proliferation of colonocytes,38 stimulate absorption of water and electrolytes,39 provide 7% to 10% of an animal’s overall energy requirements,37 and influence or modify motility of the gastrointestinal tract.40
In general, anaerobic bacteria (Bacteroides spp., Bifidobacterium spp., Clostridium spp., and Lactobacillus spp.) predominate in the canine colon, accounting for up to 90% of the colonic microflora. Most are facultative or aerotolerant anaerobes, not true obligate anaerobes.41 Enterobacteria and streptococci are the predominant aerobic bacteria found in the canine colon. In the cat, approximately equal numbers of anaerobic and aerobic bacteria are found in the colonic lumen.42
The colonic flora changes significantly with developmental stages. The aging canine colon becomes more readily populated by Clostridium perfringens and other obligate anaerobes, and less populated by aerobes and aerotolerant anaerobes.41,43 This change takes place in the ascending and descending colon, but is most significant in the descending colon where anaerobic conditions tend to predominate. Senescent changes in bacterial populations of the colon conform to the principles of successional ecology.44 Over time, changes in pH, redox (oxidation-reduction) potential, bacterial competition, and nutrient availability facilitate the proliferation of anaerobic bacteria permitting them to eventually displace the aerotolerant forms.
The colonic flora may also be influenced by an animal’s breeding and genetic background. For example, far fewer Bifidobacterium spp. are recovered from the feces of Beagle dogs than are recovered from other dog breeds.43,45 This represents a problem for studies of colonic bacteriology because many of the reference studies have been generated from Beagle dogs.
Diet has a major impact on numbers and types of bacteria recovered from the colon. Bacteroides spp. appear to be particularly susceptible to changes in the diet.45–47 The protein, carbohydrate, and fiber content of the diet all appear to influence the ability of bacteria to grow within the colon. Thus, dietary history should always be considered when interpreting results of fecal or colonic bacterial cultures.
Geographic locale and environmental conditions can also influence colonic bacteriology in the dog. Bifidobacterium spp. are readily isolated from dogs from Japan but they are inconsistently found in American dogs.41,45 Housing conditions appear to be another confounding factor. Open housing environments41 appear to facilitate colonization of a greater proportion of facultative anaerobic bacteria compared to closed facility conditions.48
Motility patterns of the colon influence the bacterial ecology of the colon. The antiperistaltic activity of the ascending and transverse colon particularly facilitates the mixing of fecal material with endogenous bacteria. Abnormalities in the motility patterns of this part of the colon lead to changes and proliferation of obligate anaerobes similar to that which is found in the descending colon.29
Disease and medical therapies, particularly antibiotics, alter the microbial characteristics of the colon. These changes are poorly understood in dogs and cats but they very likely contribute to clinical symptomatology (see Chapter 2).
Diagnostic Evaluation
History and Physical Examination
Colitis
History
Inflammation is the most important pathophysiologic condition of the colon. Colitis is responsible for the major clinical signs of hematochezia, mucus in the feces, dyschezia, abdominal discomfort, tenesmus, urgency, and increased frequency of defecation. The colon is an important target organ for inflammatory bowel disease (IBD) in the dog, whereas the upper gastrointestinal tract (stomach and small intestine) is more frequently involved in feline IBD. Clinical signs are useful in localizing the anatomic site of the diarrhea to the small or large bowel (Table 58-2), although some animals will have diffuse involvement of the small and large intestines.
Table 58-2 Clinical Signs Associated with Large Bowel Diarrhea
Signs | Small Bowel | Large Bowel |
---|---|---|
Weight loss | May be present | Uncommon |
Vomiting | May be present | Uncommon |
Flatulence | Present with malassimilation | Unusual |
Defecation frequency | Normal to mild increase | Marked increased frequency |
Fecal volume | Increased | Normal to mild increase |
Urgency | Absent | Usually present |
Tenesmus | Absent | Usually present |
Mucous in feces | Usually absent | Frequently present |
Hematochezia | Absent | Often present |
Melena | Sometimes present | Absent |
Steatorrhea | Present with malassimilation | Absent |
The history should include specific questions about diet, parasite control, environment, travel history, concurrent medical disease, and drug history. Dietary sensitivity reactions1 and parasitism are major causes of colitis in many pet populations. Dietary history should include information regarding type of diet, incidence of dietary indiscretion, supplements, snacks, and treats. Information concerning previous fecal examinations and anthelmintic usage may provide useful clues to the cause of the diarrhea. Environmental history should identify other pets in the household and the composition of their diets, and any behavioral interactions or hierarchies that might influence the development of clinical signs. The travel history may yield important information about exposure to histoplasmosis, pythiosis, and heterobilharziasis, all of which have regional distributions. Concurrent medical disease (e.g., Addison disease, IBD, pancreatitis) also helps to place the current episode of colitis in context. The drug history should include information about the use of alternative and complementary medicines that could contribute to clinical symptomatology.
Physical Examination
The physical examination may be normal in many cases of colitis. The most consistent physical examination findings are pain and irregularities of the colonic mucosa on digital rectal examination. The perineum should be examined carefully to exclude perineal diseases such as perineal hernia and perianal fistula. Physical examination may reveal other important findings, including fever (IBD, cecal or colonic perforation, fungal infection), abdominal pain (IBD, colonic neoplasia, cecal or colonic perforation), abdominal mass (colonic neoplasia, granulomatous colitis, intussusception), small intestinal thickening (concurrent small intestine IBD, small intestine lymphoma), mesenteric lymphadenopathy (small intestine IBD, small intestine lymphoma), hepatosplenomegaly (lymphoma, disseminated fungal infection), and uveitis (protothecosis, lymphoma). A scoring system has been developed to relate clinical signs with histologic findings in canine IBD.2
Constipation
Physical Examination
For both inflammation (colitis) and constipation, the scope of the medical investigation is shaped by prior history, suspected etiology, chronicity, and signs of systemic illness (Figures 58-4 and 58-5).3,4
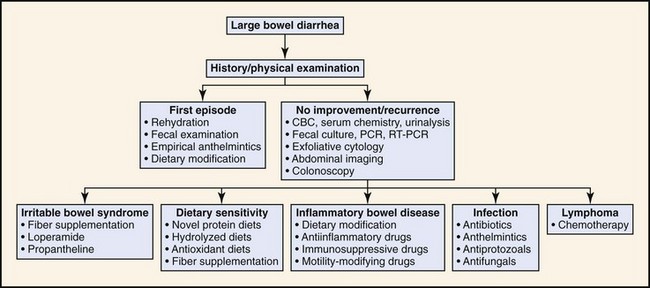
Figure 58-4 Diagnostic approach to large intestinal diarrhea.
(Reprinted with permission from Washabau RJ: Diseases of the large intestine. In: Ettinger SJ, Feldman ED, editors: Textbook of Veterinary Internal Medicine, ed 6, Philadelphia, 2005, Saunders, p 1384.)
Laboratory Data
Complete blood count, serum chemistry, and urinalysis should be considered in animals with signs of colonic disease, particularly those with signs of systemic disease. These tests may provide evidence of anemia (chronic disease, GI blood loss), leukocytosis (IBD, neoplasia, cecal or colonic perforation), eosinophilia (parasitism, Addison disease, mast cell disease, hypereosinophilic syndrome), thrombocytopenia (concurrent immune thrombocytopenia), hypoproteinemia (protein-losing enteropathy), hyperglobulinemia (IBD, infection, neoplasia), hypercalcemia (neoplasia, fungal disease), hypoglycemia (leiomyosarcoma), and hyponatremia/hyperkalemia (Addison or pseudo-Addison disease). This minimum database is also useful to screen animals prior to anesthesia and colonoscopy. If fungal, oomycete, or algal infections are possible causes in the pet’s geographic area, special serologic tests are available for the diagnosis of some of these diseases (Table 58-3). Feline leukemia virus (FeLV) and feline immunodeficiency virus (FIV) testing are warranted in cats with unexplained, chronic diarrhea.
Fecal Parasitic Evaluation
Direct fecal smears and fecal flotation studies should be performed to evaluate for helminth, protozoal, and some bacterial infections (see Table 58-3). Direct fecal smears may be useful in detecting Giardia, Tritrichomonas, and Campylobacter organisms. Zinc sulfate flotation is the most accurate and practical fecal flotation test available, and it may be more sensitive for the detection of Giardia and Tritrichomonas infections. Because some animals may have intermittent shedding of helminth ova and/or Giardia trophozoites and cysts, suspected infections should always be treated with anthelmintics or antiprotozoal agents before animals are subjected to colonoscopy.
Fecal Bacterial Culture
Fecal cultures should be considered in animals with suspected bacterial infections of the intestine and colon. Risk factors for bacterial colitis include young age, crowded or poor hygienic environmental conditions, coinfection with helminth or protozoal parasites, viral immunosuppression,5 boarding or kenneling conditions, and multiple-pet households. Bacterial pathogens associated with colitis or enterocolitis lesions in dogs and cats have included Brachyspira pilosicoli, Campylobacter spp., C. perfringens, and Clostridium difficile, certain Escherichia coli (enterotoxigenic, enteroinvasive, enteropathogenic, enterohemorrhagic, enteroadherent), Salmonella spp., and perhaps Yersinia enterocolitica (see Table 58-3). The role of enteropathogenic bacteria in the pathogenesis of colitis-type diarrhea has been reviewed recently by Marks et al.5a Culture results should always be interpreted in light of clinical signs, other findings such as coinfections, and substantiating laboratory data such as serologies or polymerase chain reaction results. Fecal cultures should not be performed for the purpose of diagnosing small intestinal bacterial overgrowth.
Molecular Diagnosis
A number of polymerase chain reaction (PCR) and reverse transcriptase (RT)-PCR assays have been developed for gene amplification products and messenger RNAs of infectious organisms (see Table 58-3). Assays are now available for the molecular diagnosis of Tritrichomonas foetus, Pythium insidiosum, Campylobacter jejuni, C. perfringens, C. difficile, E. coli, Salmonella spp., and B. pilosicoli, and many more are likely forthcoming. Molecular detection is the standard for infectious disease diagnosis in many instances.6
Fecal Cytologic Examination
Exfoliative rectal or endoscopic cytology may be useful in identifying etiologic agents (e.g., fungal elements, neoplastic cells) and inflammatory cells (e.g., lymphocytes, eosinophils). Rectal smears may be obtained with a cotton-tipped applicator or conjunctival spatula during anorectal examination, or with a cytology brush at the time of endoscopy. A good correlation (specificity, 97%; sensitivity, 93%) was shown between exfoliative cytology and subsequent endoscopic or surgical biopsy in one study.7 Additional studies are still needed to verify the diagnostic value of this test.
Imaging
Survey abdominal radiographs may occasionally document colonic foreign objects, mesenteric or sublumbar lymphadenopathy, intussusception, and extraluminal compression, but survey radiographs are generally nonspecific in the diagnosis of colonic disease. Contrast studies (e.g., barium enema) are performed infrequently because of their poor sensitivity and requirement for general anesthesia. Ultrasonographic imaging has proved more useful in documenting mass lesions, lymphadenopathy, intussusceptions, and bowel thickening. Abdominal ultrasound may also be used to facilitate percutaneous aspiration of luminal masses, mucosal thickenings, and lymph nodes.8,9 Computed tomography (CT) colonography, also known as virtual colonoscopy, has been successfully implemented in human medicine, and has been studied experimentally in the dog.10
Colonoscopy
Colonoscopy is indicated for the diagnosis of colitis-type diarrhea unresponsive to dietary modification and medical therapy, suspected colorectal neoplasia, chronic constipation, unexplained stricture, and evaluation of prior surgical or medical treatment.11 Colonoscopy is performed after other noninvasive diagnostic tests (e.g., fecal parasitologic examination, fecal bacteriologic examination, exfoliative cytology, abdominal ultrasonography, survey ± barium-contrast radiography) have failed to diagnose the disease.
Rigid or flexible endoscopy may be performed, but flexible endoscopy provides better visualization and examination of the entire colon. The normal colonic mucosa is pink in color, smooth in texture, and glistening in appearance (Figure 58-6). Unlike the esophageal, gastric, and duodenal mucosa, submucosal blood vessels are readily apparent. The mucosa should not hemorrhage when abraded by the endoscope; active hemorrhage usually implies an underlying disorder such as inflammation or infection. The colonoscopic procedure should include examination of the more proximal structures—for example, ascending colon, cecum, ileocecal sphincter, and distal ileum—whenever possible. The proximal colon is an important site of inflammation, parasitism, ileocolic intussusception, cecal inversion, and neoplasia.
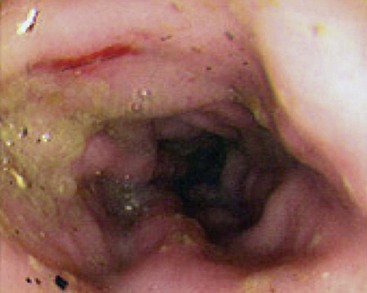
Figure 58-6 Endoscopic appearance of large intestinal inflammatory bowel disease.
(Reprinted with permission from Washabau RJ: Diseases of the large intestine. In: Ettinger SJ, Feldman ED, editors: Textbook of Veterinary Internal Medicine, ed 6, Philadelphia, 2005, Saunders, p 1386.)
Fecal material must be completely evacuated before the colonic mucosa can be properly examined (Box 58-1). Incomplete bowel preparation is the major reason for an unsuccessful colonoscopic examination. Patient permitting, food should be withheld for 36 to 48 hours so that fecal material does not accumulate in the colon. The patient should also be given warm water enemas and/or gastrointestinal lavage solutions. Lavage solutions are preferable to warm water enemas,12–14 but both may be given to facilitate successful colonoscopy. Polyethylene glycol solutions are isosmotic, are administered orally, and induce a diarrhea that rapidly removes fecal material from the bowel. Large volumes may be administered without inducing significant changes in water or electrolyte balance.12 Electrolyte solutions should be given at a dose of 25 mL/kg at 24 and 12 hours prior to colonoscopy. Warm-water enemas should be performed gently, without irritating substances, at 24, 6, and 2 hours before colonoscopy. The combination of a 36- to 48-hour food fast, gastrointestinal lavage solution, and enemas usually results in a colon that is free of fecal fluids and solids.
Endoscopic examination of the colon has been described in detail.11 General anesthesia is induced and the patient is placed in left lateral recumbency. The tip of the endoscope is introduced into the anorectal canal, and air is insufflated to dilate the anorectal and colonic lumen. As with upper gastrointestinal endoscopy, the tip of the endoscope should never be advanced unless the lumen is in full view. While inspecting the colonic mucosa, the endoscope is advanced through the descending colon to the splenic flexure. To facilitate passage of the endoscope through the splenic flexure, the tip of the endoscope is deflected slightly upward, and the endoscope is pushed into the transverse colon. The transverse colonic mucosa is now more visible as the endoscope enters the short, straight segment of the transverse colon. At the hepatic flexure, the tip of the endoscope is deflected caudally to enter the ascending colon. While keeping the tip of the endoscope in the center of the lumen, the endoscope is advanced the full length of the ascending colon into the cecum and ileocecal sphincter. The cecum is easily inspected, and the endoscope is then advanced through the ileocecal sphincter to evaluate the distal ileum. Endoscopic biopsies may be obtained at each sequential site or during withdrawal of the endoscope at the end of the study (see Chapter 27).
Diseased tissue, nondiseased tissue, and the transition zone between diseased and nondiseased tissue should be biopsied during colonoscopic procedures. This standard helps to verify the extent of the disease, ensures that disease in the submucosa has not been missed, and provides representative tissue samples to the pathologist to diagnose the disease.15 In some cases of severe IBD or colonic neoplasia, advanced tissue necrosis may prevent the diagnosis of the disease process in the central part of the lesion. In the absence of gross mucosal abnormalities, three to five biopsy specimens should be obtained from each of the mid-ascending, mid-transverse, and mid-descending colonic regions (see Chapter 29).
Inflammation
Frédéric P. Gaschen and Karin Allenspach
Inflammatory Bowel Disease
Definition
IBD is an idiopathic inflammatory disorder affecting the gastrointestinal (GI) tract of dogs and cats. Canine and feline IBD can be defined on the basis of clinical and histopathologic criteria. Clinically, IBD is diagnosed in animals with chronic (longer than 3 weeks’ duration) GI signs such as anorexia, vomiting, diarrhea (small and/or large bowel in origin), and weight loss for which no other known cause of gastroenterocolitis can be documented. Therefore, affected animals fail to respond to symptomatic treatment, including parasiticides, antibiotics, and diet.1 In addition, histopathologic evaluation reveals inflammatory infiltration of the colonic mucosa with predominantly round cells (lymphocytic plasmacytic colitis), eosinophils (eosinophilic colitis), neutrophils (suppurative or neutrophilic colitis), macrophages (granulomatous colitis), or a combination thereof. The inflammatory process can solely involve the colon (colitis) or affect the whole GI tract (enterocolitis).
Pathophysiology and Mechanism
Immunologic Basis of IBD
In people, IBD encompasses two main disorders: Crohn’s disease can occur anywhere in the GI tract and is characterized by a transmural inflammation, whereas ulcerative colitis is restricted to the colon and is characterized by a mucosal inflammation. IBD is clearly a multifactorial disease in which physiologic interactions of the innate and adaptive immune system with luminal microbial antigens are disrupted.2,3 Overall, three factors are thought to be required for the development of IBD: (a) the presence of bacteria in the intestinal lumen with potential dysbiosis in the luminal microbiome; (b) defective mucosal barrier function that allows bacterial and/or food antigens to come in contact with the innate and adaptive immune cells in the lamina propria; and (c) an aberrant innate and adaptive mucosal immune response to bacterial antigens.2,3
In some human IBD patients, genetic alterations affecting the expression of important molecules of the innate immunity (e.g., polymorphisms in the gene encoding the nucleotide oligomerization domain 2 [NOD2]) are responsible for the abnormal recognition and response to luminal microbiota.2 NOD2 is an intracellular pattern recognition receptor that binds to muramyl dipeptide derived from peptidoglycan common to both Gram-positive and Gram-negative bacteria.
Toll-like receptors (TLRs) are a family of transmembrane receptors with a ligand-binding extracellular domain that recognize microbe-associated molecular patterns. They are an important component of the innate immune system. In the GI tract, specific TLRs are found located on epithelial cells, macrophages, and dendritic cells. TLR2 recognizes bacterial lipopeptides, TLR4 is activated by lipopolysaccharides, and unmethylated cytosine-phosphate diester–guanine Cpg DNA found in prokaryotic genomes and DNA viruses act as ligand for the intracellular receptor, TLR9.4,5 Once activated, TLRs elicit an intracellular signaling cascade leading to the production of proinflammatory cytokines. However, the physiologic function of TLRs in the GI tract includes maintaining hyporesponsiveness to innocuous luminal commensals, inhibition of allergic responses to food allergens and protection of mucosal barrier function.4,5 Polymorphisms in the genes encoding TLR1, TLR2, TLR4, and TLR6 are associated with an increased risk for development of IBD in human patients and in murine animal models.4,5
NOD and TLRs are also a focus of attention in canine chronic enteropathies. Cultured primary canine colonocytes can express NOD2, TLR2, and TLR4 when stimulated by their respective ligands.6 In a study investigating TLR expression profiles in endoscopic biopsies, it was found that dogs with chronic enteropathies that needed steroid treatment to keep their symptoms controlled had increased levels of TLR2, TLR4, and TLR9 messenger RNA (mRNA) expression compared with healthy dogs.7 However, no difference in TLR expression was detected between biopsies sampled before and after clinically successful therapy, regardless of whether dogs had been treated with diet or steroids.7,8 Another study identified a correlation between clinical severity of disease and expression of TLR mRNA. Duodenal mucosal mRNA expression of TLR2 was markedly increased in dogs with clinically severe IBD when compared with healthy controls, and TLR2 expression was correlated with clinical severity of diseases in a linear fashion.8 Breed differences in TLR expression profiles have been reported in dogs with IBD. German Shepherd Dogs (GSD) with IBD have increased levels of TLR4 mRNA and decreased levels of TLR5 mRNA in the intestine compared to healthy greyhounds.8a Differences in TLR expression mimic those reported in people with Crohn’s disease and have prompted genetic investigations into polymorphisms of pattern recognition receptors in dogs with IBD. In GSDs, single-nucleotide polymorphisms (SNPs) in TLR4 and TLR5 have been associated with an increased incidence of IBD.8b SNPs in TLR5 are associated with increased risk of development of IBD in 38 other breeds, and have been shown to be functionally hyper-responsive in GSD. This particular mutation may play a role in the pathogenesis of IBD in dogs in general.8b Finally, although it does not appear that food antigens play a direct role in the pathogenesis of human IBD, various dietary components can exert deleterious or beneficial effects on the intestinal microflora and on the intestinal mucosa, making it plausible that dietary changes could influence the inflammatory process in the mucosa of dogs with IBD.9 To date, the effects of dietary antigens on canine and feline IBD have not been studied in detail. However, dietary treatment is viewed by most as an integral part of the therapeutic approach to chronic inflammatory GI diseases.
A number of studies have investigated the inflammatory process associated with canine and feline colitis. Immunohistochemical studies have shown T and B lymphocytes, as well as IgG-positive plasma cells to be increased in the colonic mucosa of dogs with IBD.9–11 Semiquantitative RT-PCR has been used to evaluate cytokine mRNA expression in colonic mucosal biopsies. Human ulcerative colitis patients typically show a Th2 dominated inflammatory response.2 In one study the prevailing cytokine mRNA expression pattern in dogs was that of a Th1 immune response.12 Nevertheless, the accuracy of these results has since been questioned, at least in the duodenal mucosa, where the more reliable quantitative real-time RT-PCR showed a lack of difference in cytokine mRNA expression between healthy dogs and dogs with IBD.13 However, colonic cytokine mRNA expression has not been investigated again using the more accurate amplification techniques, and it is prudent not to overinterpret the results from the initial study. To date there are no published data about cytokine expression in feline colitis, but duodenal mucosa of cats with IBD revealed a complex pattern of cytokine mRNA expression with a possible increase in Th1 cytokine mRNA expression.14,15
Influence of Diet
A high proportion of dogs and cats with colitis respond to dietary therapy alone (Table 58-4); however, it is important to distinguish the various reasons for diet-responsive colitis. In humans, as well as in dogs and cats, adverse reactions to food can result from immunologic (food allergy) and nonimmunologic (food intolerance) mechanisms.16 So far, in small animals, the clinical differentiation between food allergy and food intolerance relies heavily on restricted antigen dietary trials.17,18 All dogs and cats with GI manifestations of adverse reaction to food get better when fed a novel diet, especially if it is designed to avoid antigens from their original diet. When challenged with their original food, they all show a relapse of GI signs. However, only food-allergic dogs and cats also relapse when their low-allergen diet is supplemented with proteins from a single source such as beef, chicken, milk, or any protein that was part of their original offending food.17 Finally, a number of animals with colitis may simply benefit from being fed a highly digestible diet. These dogs and cats may have mild IBD that responds to the novel diet, which usually also offers additional benefits, such as an improved ratio of n6-to-n3 fatty acids or the presence of prebiotics. These animals usually do not experience a relapse when fed their original diet.
Table 58-4 Efficacy of Various Treatments for Chronic Colitis in Dogs and Cats Based on Published Case Series
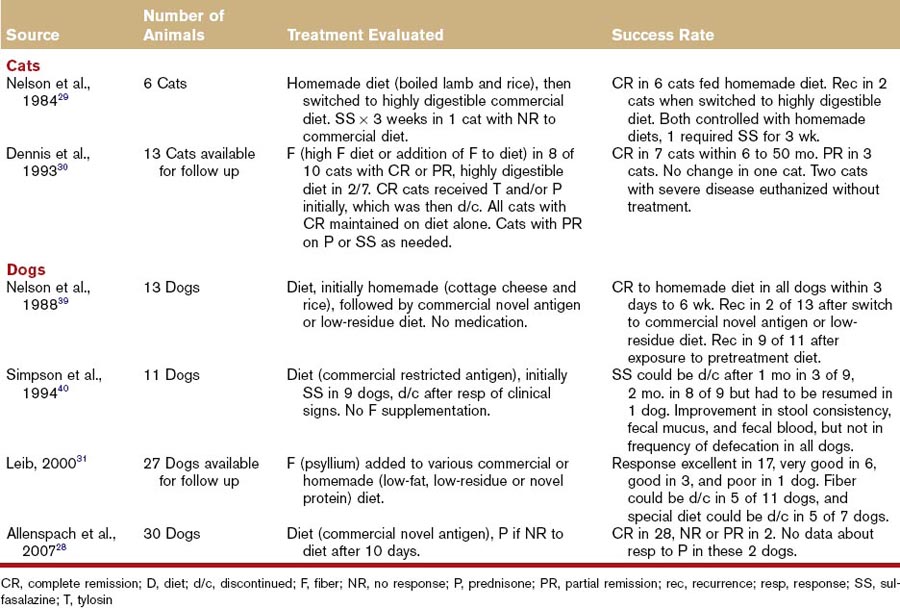
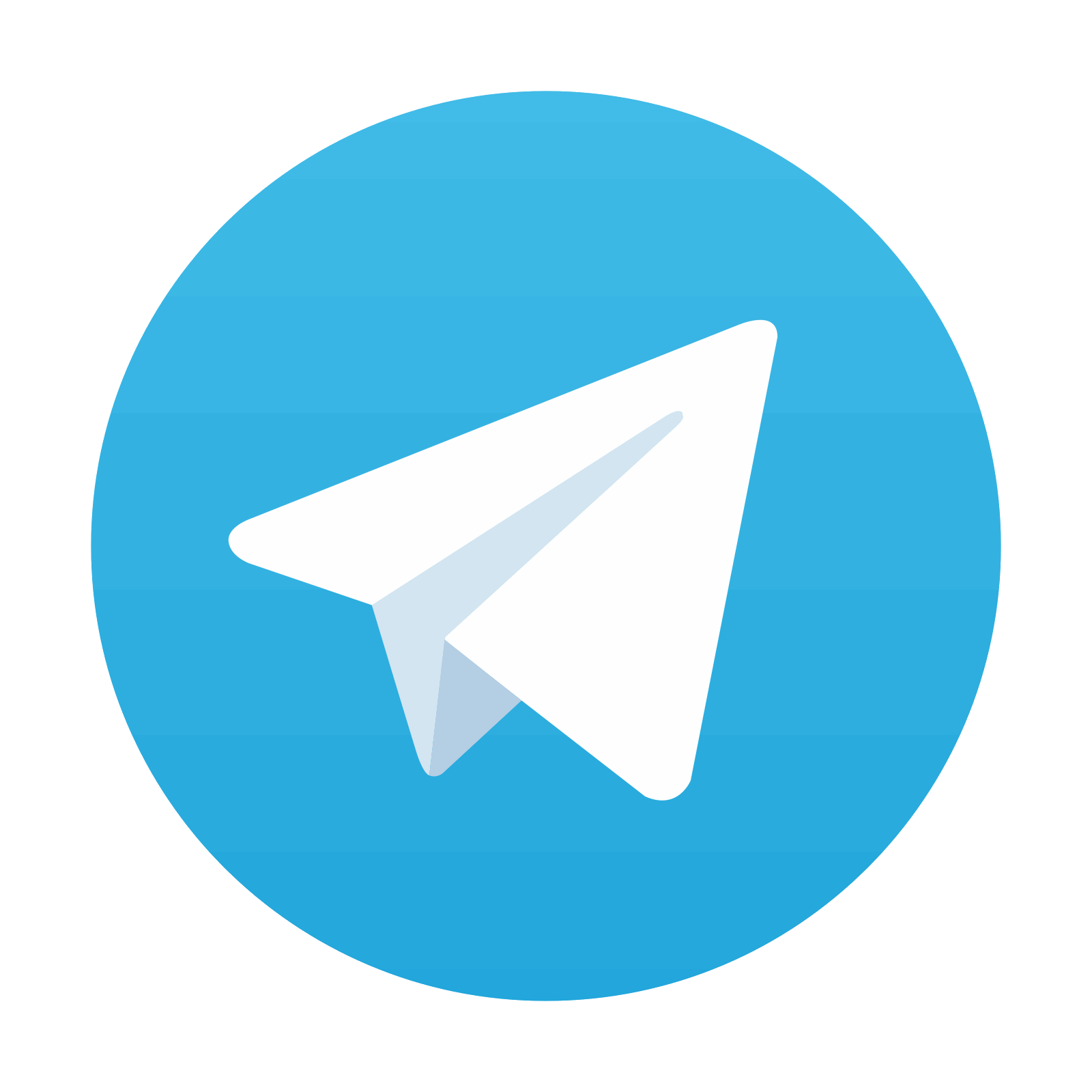
Stay updated, free articles. Join our Telegram channel

Full access? Get Clinical Tree
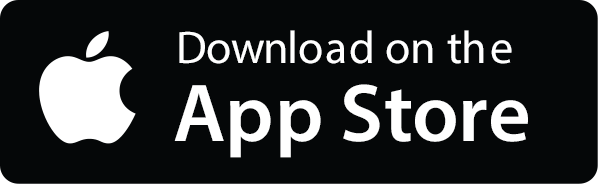
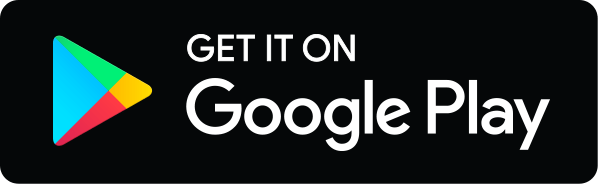