Chapter 25 Laboratory Approach
Stomach and Small Intestine
Diagnostic imaging, especially a combination of ultrasonography and radiography, can be helpful for evaluation of patients for obstructions or neoplasia. Endoscopic examination and biopsy of the GI tract is currently regarded as the gold standard and yields information about macroscopic lesions and changes in histologic appearance of the mucosa; however, this approach is invasive. Furthermore, histologic interpretation of biopsy specimens can be highly variable among individual pathologists.1
Detection of Bacterial Pathogens
Specific enteropathogens (e.g., Clostridium perfringens, Clostridium difficile, Salmonella spp., Escherichia coli, Campylobacter spp., or Yersinia enterocolitica) are associated with GI disease in dogs and cats. However, most of these enteropathogens are commensals in the GI tract and have been isolated at similar frequencies from diarrheic and nondiarrheic animals.2 This complicates the clinical interpretation when presumptive enteropathogens are identified. Therefore adjunct laboratory testing for the presence of virulence genes (e.g., E. coli virulence factors), and/or toxins (e.g., C. perfringens and C. difficile) can be helpful in determining the clinical importance of isolated enteropathogens.
Detection of Helicobacter spp.
A high prevalence of Helicobacter-like organisms (HLOs) has been reported in the stomach of dogs and cats, with up to 100% of healthy animals being positive in some studies.3 HLOs are also highly prevalent in the intestine and can be found occasionally in the liver. Helicobacter pylori, the clinically most important Helicobacter species in man, is typically not isolated from companion animals and has been identified so far only in colony cats. The most commonly observed HLOs in pet cats and dogs are Helicobacter felis, Helicobacter heilmannii, Helicobacter salomonis, Helicobacter bilis, Flexispira rappini, Helicobacter bizzozeronii, and Helicobacter baculiformis. Animals usually harbor several different Helicobacter spp. The detection of Helicobacter spp. is based on invasive or minimally invasive methodologies.
Minimally Invasive Methods
Minimally invasive methods allow the detection of Helicobacter spp., but do not allow assessment of concurrent GI disease associated with Helicobacter spp. Serologic tests have a relatively low sensitivity. Most commercial serologic assays test for immunoglobulin (Ig) G and IgM specific for the human pathogen H. pylori, and these assays therefore have little value in companion animals. Antibodies will circulate for several months after eradication of the organism and thus antibody tests have limited use for monitoring therapeutic success.4 The 13C-urea breath test is based on the detection of metabolic activity of Helicobacter spp. The organisms produce the enzyme urease, which catalyzes the metabolism of orally administered 13C-urea. In turn, 13C is released from the urea, incorporated into 13CO2, and can be quantified in either breath or blood samples. This method is the diagnostic test of choice in humans and is also used to evaluate the success of treatment. A 13C-urea breath and blood test has been evaluated for use in dogs, but is not offered commercially at this time.5 Using PCR, Helicobacter DNA can also be detected in fecal samples, but the sensitivity of this approach is unclear in companion animals.6
Invasive Tests
Gastroscopy not only allows for the detection of Helicobacter spp. but also provides information about the presence of gastric lesions. Gastroscopy allows for direct visualization of the gastric mucosa. Helicobacter spp. have a typically patchy distribution in the mucosa, and it is recommended that biopsy specimens or impression smears be obtained from several areas of the stomach.7 However, the cultivation of Helicobacter spp. from biopsy specimens is of low sensitivity. Helicobacter spp. can be detected in biopsies (Fig. 25-1) by means of histochemical stains (e.g., Warthin-Starry or modified-Steiner stains), immunohistochemistry, fluorescence in-situ hybridization (FISH), PCR, or commercially available rapid-urease tests.8,9 It is important to note that the rapid-urease tests (e.g., CLOtest, Kimberly-Clark) may require up to 24-hour incubation before displaying a color change indicating a positive test result, especially if the patient has received acid-suppressing therapy. Brush cytology of the mucosa is a highly sensitive method for the detection of Helicobacter spp. The material obtained is spread across a microscope slide and stained with May-Grünwald-Giemsa, Gram, or Diff-Quick stain.8
Diagnosis of Clostridium perfringens–Associated Diarrhea
Bacterial culture for the isolation of C. perfringens has little diagnostic value as C. perfringens is a commensal that can be detected by PCR in up to 100% of dogs and cats.10 The development of C. perfringens–associated diarrhea has been primarily related to the presence of various toxins, especially C. perfringens enterotoxin (CPE). A subset of C. perfringens organisms carries the gene coding for CPE. When sporulation occurs (this is poorly understood, but suspected triggers include dietary changes or antibiotic use), the enterotoxin is released in high quantities and may potentially cause changes in intestinal permeability leading to diarrhea. Enterotoxigenic C. perfringens can be detected by PCR assays that target the CPE gene; however, our recent unpublished studies reveal that 20% of healthy cats, 37% of healthy dogs, and 37% of diarrheic dogs and cats harbor the CPE gene. Similar results with no significant differences in the prevalence of enterotoxigenic C. perfringens between healthy and diarrheic dogs have been reported in the literature.11 Furthermore, of those animals that carry enterotoxigenic C. perfringens, the expressed enterotoxin can only be detected in a small proportion of cases. Because of this, and because of the high rate of enterotoxigenic C. perfringens carriage in healthy animals, it is recommended that combined testing for the CPE gene by PCR and for the C. perfringens enterotoxin by fecal enzyme-linked immunosorbent assay ([ELISA]; e.g., C. perfringens enterotoxin test; Techlab, Blacksburg, Virginia) should be performed.11 Other enterotoxin tests such as the reverse passive latex agglutination (RPLA) assay have a lower analytical sensitivity and are not recommended. Also, enumeration of C. perfringens endospores is not considered to be a reliable test for diagnosing C. perfringens–associated diarrhea in dogs. In one study, no association was established between C. perfringens endospore counts and the detection of CPE, and no difference was observed in the number of observed C. perfringens endospores between diarrheic and nondiarrheic dogs.2
Diagnosis of Clostridium difficile–Associated Diarrhea
Isolation rates for C. difficile range between 0% and 40%, and this organism has been isolated in similar frequencies from diarrheic and nondiarrheic dogs.2,12 Approximately 50% of C. difficile organisms harbor toxin A and B genes. Consequently, the significance of detecting C. difficile organisms in dogs with diarrhea remains unclear. Currently the most accurate method for diagnosis of C. difficile–associated diarrhea is the detection of the organism (either by culture or by antigen testing for glutamate dehydrogenase) in combination with the detection of toxins A and B by ELISA.
The tissue culture cytotoxin-B assay detects the presence of toxin B and is considered the most sensitive (94% to 100%) and specific (99%) test for the diagnosis of C. difficile–associated diarrhea.13 It is, however, technically challenging and requires a 2- to 3-day turnaround time. The latex particle agglutination (LPA) assay and RPLA assay have a high rate of false-positive and false-negative results and are not recommended. Various immunologic assays have been developed that allow the detection of either toxin A or B or both toxins simultaneously. While most C. difficile strains contain toxin A (enterotoxin) and toxin B (cytotoxin), the occurrence of toxin A−/toxin B+ strains has been reported. Consequently, it is recommended to use immunoassays that target both toxins. Commercially available human ELISAs that measure both toxins (Premier ToxinA/B and the Techlab ToxinA/B) show a high sensitivity (93%) and specificity (95%) when testing cultured isolates of C. difficile. Both ELISAs show a lower sensitivity (27% to 33%) when canine fecal samples are tested directly for the presence of both toxins.13 Although a positive toxin ELISA result is suggestive of C. difficile–associated diarrhea in animals with compatible clinical signs, confirming the presence of the organism improves the diagnostic accuracy. In man, it is recommended that up to three consecutive samples be tested if the first result is negative.
Detection of Campylobacter spp
The normal GI tract harbors various spiral shaped bacteria, including Campylobacter, Helicobacter, Spirochaetes, and Anaerobiospirillum.10 It is not possible to reliably distinguish these organisms on fecal smear examination. At least 14 different Campylobacter spp. have been identified in the GI tract of dogs and cats, and most animals harbor multiple species.14,15 Campylobacter upsaliensis and Campylobacter helveticus have been reported as the most prevalent Campylobacter spp. in dogs and cats, respectively.14,15 In contrast, Campylobacter jejuni and Campylobacter coli, the most commonly identified pathogens in man are infrequently isolated from dogs and cats, with prevalence rates ranging between 1% and 10% in most studies.15,16 Because various Campylobacter spp. are part of the normal intestinal ecosystem, species-specific assays able to identify the potentially pathogenic and zoonotic C. jejuni or other Campylobacter spp. of interest must be used instead of broad-range culture or detection assays.
Escherichia coli
E. coli is a normal inhabitant of the GI tract of dogs and cats.10 However, diarrheic dogs more commonly harbor E. coli possessing specific virulence genes.17 These include enteropathogenic E. coli (EPEC), enterotoxigenic E. coli (ETEC), verocytotoxin-producing E. coli (VTEC), enterohemorrhagic E. coli (EHEC), and enteroinvasive E. coli (EIEC). Consequently, molecular assays for the detection of these virulence genes must be employed to detect pathogenic strains of E. coli.
Recently, adherent and invasive E. coli (AIEC) strains were associated with the development of histiocytic ulcerative colitis in Boxer dogs, which responds to antimicrobial therapy.18 The AIEC strains can be identified using FISH in mucosal biopsies.
Viral Enteritis
Parvovirus
The diagnosis of parvovirus infection requires a rapid result to initiate immediate treatment. Commonly used in-clinic immunoassays (e.g., SNAP Parvo Test, IDEXX Laboratories) have high positive and negative predictive values for the detection of canine parvovirus (CPV)-2 and can also detect feline panleukopenia virus.19,20 New studies also show that these assays identify all three CPV types—2a, 2b, and 2c.19 However, low virus loads may remain undetected with in-house immunoassays and PCR assays have been shown to have a superior analytical sensitivity.21 Samples from animals that are suspected to have parvovirus infection, but have a negative SNAP test result, can be submitted to a reference laboratory for assessment by PCR or electron microscopy. A disadvantage of the in-house SNAP test is that it cannot differentiate vaccine strains from infectious strains of CPV-2.
Detection of Gastrointestinal Parasites in Feces
Fecal Examination Techniques
Fecal flotation techniques allow the separation of parasites from fecal debris because of the difference in specific gravity of parasite forms (Fig. 25-2). If the flotation solution has a higher specific gravity than the parasite form (e.g., eggs, oocysts, cysts), these will rise to the surface and can be identified (Table 25-1). Because flotation techniques allow the concentration of parasites, a higher sample volume (1 to 5 g) can be analyzed, improving the analytical sensitivity. Fecal flotation should be prepared on fresh feces (<2 hours). Flotation can be performed either as a passive separation technique (e.g., commercial kits including Fecalyzer, Ovassay) or as a centrifugation technique with either a fixed rotor or a swinging bucket rotor. Centrifugation techniques show a consistently higher recovery compared with passive techniques and are currently recommended for routine use in practice. In one study, a direct smear technique failed to identify whipworm eggs 93% of the time.22 A passive flotation technique (e.g., Ovassay) yielded false-negative results in 30% of samples, while the centrifugation technique using Sheather’s sugar solution yielded false-negative results in only 5% of the samples examined.22 Similar results were also observed for other parasite types. The recovery of parasites using commercially available passive flotation kits (e.g., Ovassay) can be improved if the flotation solution is applied for at least 20 minutes.
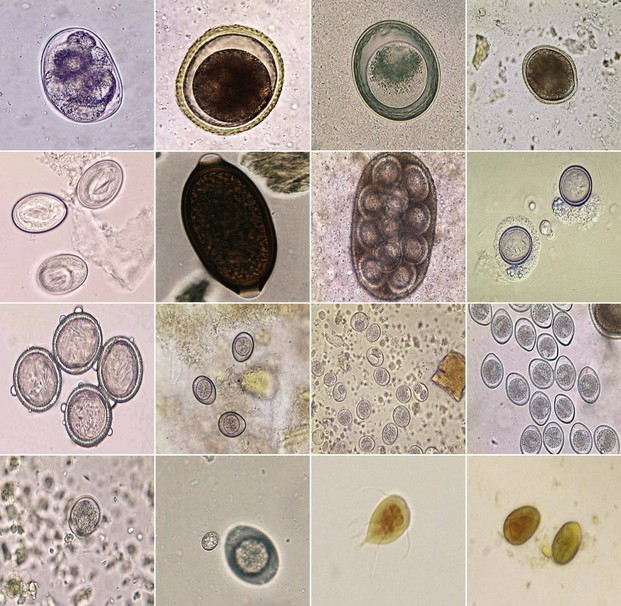
Figure 25-2 Commonly observed parasite eggs, oocysts, and trophozoites in fecal samples.
(All photos courtesy of Dr. Byron L. Blagburn, College of Veterinary Medicine, Auburn University.)
Box 25-1 summarizes the standard procedure for the centrifugal flotation technique. The specific gravity of the flotation solution plays a critical role in the identification of parasites. The higher the specific gravity of the parasite egg, the higher must be the specific gravity of the flotation solution to allow the ova to rise to the surface. For example, eggs of Physaloptera spp. (specific gravity 1.14) and Taenia spp. (specific gravity 1.23) have a higher specific gravity than the commonly used ZnSO4 solutions (specific gravity 1.18), thus they will likely remain undetected. Table 25-2 lists the specific gravity of commonly used flotation solutions, and Table 25-1 lists the specific gravity of common parasite eggs. A specific gravity hydrometer may be used to measure the correct specific gravity of the solution. The use of a micrometer to measure the size of cysts and eggs may help identify parasites (see Table 25-1). The use of centrifugation and Sheather’s sugar solution (specific gravity 1.27; commercially available from Jorgensen Laboratories) is currently recommended as the most efficient method for the recovery of the most common parasite eggs and oocysts.23
Box 25-1
Procedure for Fecal Examination Using a Centrifugation Technique
1. Two to 5 g of feces (preferably abnormal stool) are mixed with 10 mL of flotation solution.
2. This emulsion is poured through a tea strainer or a cheese cloth into a fecal cup. Straining is important to remove the heavy fecal matter.
3. The emulsion is poured into a 15-mL conical centrifugation tube. The amount of flotation solution to be added depends on the rotor used (swinging bucket or fixed angle).
4. The coverslip is removed after 10 minutes and placed on a glass slide. The entire glass slide should be systematically scanned using ×10 magnification (i.e., 100 diameters). The ×40 magnification can be used for identification and measurement of parasites. It is important to standardize the procedure (including microscopic examination) for consistent results.
Table 25-2 Specific Gravities of Flotation Solutions
Solution | Specific Gravity* | Preparation in Water |
---|---|---|
Water | 1.00 | n/a |
Sodium chloride | 1.18 | 350 g per 1 L |
Magnesium sulfate | 1.20 | 450 g per 1 L† |
Zinc sulfate | 1.18 | 331 g per 1 L |
Sodium nitrate | 1.18 | 338 g per 1 L |
Sheather’s sucrose¶ | 1.27 | 454 g per 355 mL w/ 6 mL formaldehyde† |
* Verify specific gravity with hydrometer.
† Water must be heated to get substance into solution.
Diagnosis of Parasites of the Esophagus and Stomach During Fecal Examination
Spirocerca lupi eggs are barrel shaped and elongated with parallel sides (Fig. 25-3). Compared with other parasite eggs they are small (see Table 25-1), typically measuring 20 to 37 × 11 to 18 µm, and contain a larva when laid. The current gold standard for the detection of S. lupi is esophageal endoscopy and is required for differentiation between neoplastic and nonneoplastic S. lupi nodules.25 Thoracic radiography is up to 86% sensitive if signs of thoracic vertebrae spondylitis, an undulating aortic border, and a caudal mediastinal mass are included.24 The reported sensitivity for the detection of eggs in feces is low and was highest (reaching 67%) using a centrifugal flotation technique with NaNO3 (specific gravity 1.22).25
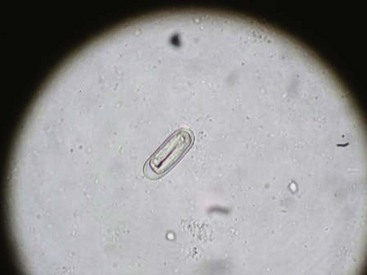
Figure 25-3 Spirocerca lupi egg containing a larva.
(Picture courtesy of Dr. Jevan Christie, University of Pretoria, South Africa.)
Stomach worms can cause vomiting, anorexia, melena, and anemia. The small stomach worm of cats (Ollulanus tricuspis; size 0.7 to 0.9 cm) can be detected during microscopic examination of vomitus or gastric lavage solution. O. tricuspis is sometimes also observed in dogs. Eggs of the large stomach worm of dogs and cats (Physaloptera spp.) have a high specific gravity (see Table 25-1) and are therefore difficult to detect in fecal smears or by fecal flotation using standard flotation solutions. However, as a result of the size and appearance of the worms (1 to 6 cm in length, cream to white colored, often coiled), they can be sometimes visualized in vomitus or during endoscopic examination.
Diagnosis of Parasites of the Small and Large Intestine
Giardia spp
A centrifugation technique using Sheather’s Sugar Solution is currently considered the best method for performing routine fecal flotation in practice; however, the sugar solution may distort or even destroy Giardia cysts, making them more difficult to diagnose for an inexperienced examiner. Therefore, the use of ZnSO4 (specific gravity 1.18 to 1.2) may be a better method for diagnosis of Giardia spp. in practices that do not routinely analyze fecal samples. However, even the use of ZnSO4 requires an experienced examiner and false negatives can occur. The in-clinic Giardia SNAP test (IDEXX SNAP Giardia test) has a comparable sensitivity to ZnSO4 centrifugation and ameliorates the need for identification of Giardia cysts based on morphology. However, false-negative results can occur and the combination of the SNAP Giardia test and ZnSO4 centrifugation performed on the same sample may show the highest sensitivity.23 Giardia cysts are shed intermittently and a single negative result on either or both of these tests does not rule out a Giardia infection. Therefore, the examination of three consecutive fecal samples over a period of 7 days (or pooled samples from consecutive defecations) examined using both ZnSO4 and the Giardia SNAP test may achieve 94% accuracy.26 It is important to note that the number of recovered cysts is typically independent of the clinical disease. Cysts are shed intermittently and it is not possible to draw conclusions about the severity of the disease.
The detection of Giardia antigen using a fluorochrome-labeled monoclonal antibody (immunofluorescence antibody test [IFA]; Merifluor Cryptosporidium/Giardia Direct Immunofluorescence Test Kit; Meridian Bioscience Inc, Cincinnati, Ohio) is often used as the gold standard for the diagnosis of a Giardia infection (Fig. 25-4).27,28 A recent study compared IFA with ZnSO4 flotation centrifugation, the in-clinic IDEXX SNAP Giardia test, and a commercial ELISA for Giardia antigen (Giardia II Test Direct ELISA; Techlab Inc, Blacksburg, Virginia). The authors found discordant results, suggesting that at the observed prevalence rates in a clinical setting (estimated prevalence rate: 4% to 10%), the in-house tests have poor positive predictive values but good negative predictive values.27 This would suggest that when used in practice, these assays are helpful in ruling out giardiasis, but are incapable of ruling in the diagnosis.27 These results also indicate that the in-house methods should not be used as screening tools in healthy animals, as false-positive results may occur, leading to an improper diagnosis and treatment.27
The use of a nested PCR assay for the detection of Giardia from fecal samples has been reported, and has shown good sensitivity for detecting Giardia duodenalis in cats.29 The nested PCR allows determination of the genotype (also called assemblage) of the organisms, which is useful for epidemiologic studies.29,28
Cryptosporidium spp
Cryptosporidium oocysts can be isolated using centrifugal flotation with sucrose, but this technique showed a low sensitivity of 21.4% in one study.30 The oocysts have a spheroid shape and because of their very small size (4 to 6 µm in diameter), they can easily be missed on fecal smears. An acid-fast stain will stain the oocysts red on fecal smears. The currently recommended test for detection of Cryptosporidium spp. is an IFA that will also simultaneously identify Giardia cysts (Merifluor Cryptosporidium/Giardia Direct Immunofluorescence Test Kit; Meridian Bioscience Inc, Cincinnati, Ohio). This test requires a fluorescence microscope and is offered by major reference laboratories. Figure 25-4 illustrates the size comparison between Cryptosporidium oocysts and Giardia cysts using the IFA.
A PCR test has been described, showing a higher analytical sensitivity for Cryptosporidium parvum in feline fecal samples compared with IFA.31 In a study of dogs and cats with diarrhea, 24.3% were positive for Cryptosporidium on PCR, while only 2.7% were positive on IFA.32 Commercially available in-clinic immunoassays have a low analytical sensitivity (42.9% to 71.4%) compared with IFA, but have been shown to have acceptable specificity (>96%) for detecting Cryptosporidium spp. in feline fecal samples.30
Canine Schistosomiasis
Canine schistosomiasis, also named bilharziasis, is caused by the trematode Heterobilharzia americana. Nutria, raccoons, and other vermin are the natural reservoirs for H. americana. Transmission is via penetration of the skin by cercariae in water sources containing the snail intermediate host. Clinical signs of infected dogs include lethargy, weight loss, hyporexia, vomiting, and diarrhea.33 Diagnosis can be made on visualizing a granulomatous response to the migrating eggs in the hepatic or intestinal tissue at necropsy or histopathology. Parasite eggs can be demonstrated using saline fecal sedimentation (Fig. 25-5).34 Canine schistosomiasis can also be diagnosed with an immunoassay detecting antigen in feces or urine that was originally developed for use in humans or by a fecal PCR assay specific for H. americana.33
Tritrichomonas foetus
T. foetus, a flagellated protozoan, has been identified as a pathogen in cats, causing diarrhea after both experimental and natural infection.35 Trichomonads do not form cysts, but they reproduce by binary fission and are transmitted directly between hosts as trophozoites (Fig. 25-6). Pathogenic and nonpathogenic species of trichomonads can be found in the GI tract of dogs and cats. Diagnosis of a T. foetus infection can be made by the visualization of trophozoites on a direct fecal smear, by culture or PCR analysis of fecal material, or by observation of organisms in colonic mucosal biopsy specimens. The best results for all the detection methods are obtained if diarrheic stools are examined. Direct fecal smear examination has a low sensitivity (<14%) and low specificity (T. foetus can be misdiagnosed as Giardia spp. or the nonpathogenic Pentatrichomonas hominis). For the cultivation of organisms, the in-house culture system (In Pouch TF; Biomed Diagnostics, San Jose, California) should be inoculated with approximately 50 mg of freshly voided, loop or flush-collected feces, and then incubated at 25°C (77°F) for up to 12 days.36 The pouch must be evaluated under a microscope every couple of days. Giardia spp. and P. hominis organisms do not survive in the culture media for longer than 24 hours and thus positive cultures are strongly suggestive of T. foetus infection.36 The reported sensitivity for culture is 1000 T. foetus organisms per 50 mg of feces. T. foetus DNA can be amplified from fecal samples by PCR.37 A nested PCR assay has been reported as the most sensitive method for detecting T. foetus in fecal samples (10 T. foetus organisms per 50 mg of feces).
Assessment of Gastrointestinal Function and Pathology
Gastrointestinal Permeability Testing
Various GI diseases can cause epithelial damage that will lead to an increase in intestinal permeability and subsequently to the translocation of antigens and/or pathogens through the intestinal mucosa. For the assessment of GI permeability exogenous nonmetabolizable markers, such as polyethylene glycol (PEG), mono- and disaccharides, or radiolabeled substances are administered orally.38–40 An increased recovery of those markers (e.g., lactulose, 51-chromium-labeled ethylenediaminetetraacetate [51Cr-EDTA]) in serum or urine indicates an increase in GI permeability, while a decreased recovery of other markers indicates a decreased absorptive capacity of the marker (e.g., methylglucose).39 Traditional probes such as 51Cr-EDTA and PEG do not undergo metabolism or bacterial degradation in the GI tract and remain intact during their passage through the gut. Therefore the increased appearance of such probes in serum or urine indicates an increased permeability of the GI tract, but it is not helpful in localizing a specific site of the GI tract. In contrast, the various sugar probes are metabolized or undergo bacterial degradation in different parts of the GI tract. Therefore, a mixture of sugar probes can be used to help localize the site of increased GI permeability. For example, orally administered sucrose is too large to penetrate the intact gastric mucosa and is broken down by brush border enzymes upon entering the small intestine. Therefore, sucrose is typically not recovered in urine or serum of healthy animals with an intact gastric mucosa. However, an increase in the urine or serum concentration of sucrose is indicative of an increased gastric permeability suggestive of gastric mucosal damage. In contrast, the sugar probes lactulose and mannitol will survive the passage through the stomach and proximal small bowel, but will undergo bacterial degradation in the distal small intestine and colon. Therefore, changes in their recovery suggest an altered permeability in the small intestine. Sucralose is resistant to bacterial degradation in the colon and is also not absorbed in the GI tract. An increase in serum or urine sucralose can therefore be indicative of increased GI permeability in any part of the intestine. If sucralose is used in combination with other sugar probes, it can serve as a marker for large bowel permeability (i.e., increase in sucralose concentration but normal concentration of small intestinal sugar probes).
Rhamnose (R) and lactulose (L) are commonly used as markers for small intestinal permeability. The monosaccharide rhamnose crosses the epithelium passively through small pores in the intestinal cells (transcellular uptake). The disaccharide lactulose crosses the epithelium through larger pores located near the tight junctions of the intestinal cells (paracellular uptake). These larger pores occur less frequently than the smaller pores that allow penetration of monosaccharides. In animals with an intact intestinal epithelium, less lactulose in relation to rhamnose (expressed as lactulose/rhamnose [L/R] ratio) will cross the intestinal mucosa and can be recovered in serum or urine. Intestinal disorders cause a decrease in the total epithelial surface area in the intestine, causing a reduction in the transcellular pores and an increase in the permeability of the tight junctions. This results in an increase in the L/R recovery ratio. For the evaluation of small intestinal absorptive capacity, the monosaccharides xylose (X) and methylglucose (M) are commonly used, as they both are absorbed through a carrier-mediated transport. The two different markers are often measured together to assess intestinal damage, as xylose is absorbed by fructose carriers and methylglucose is absorbed by glucose carriers in the small intestine.38,40
Measurement of permeability may be helpful in disorders that are not accompanied by histopathologic changes in the GI mucosa. For example, increases in intestinal permeability have been reported in dogs with small intestinal bacterial overgrowth (SIBO), gluten-sensitive enteropathy, intestinal ischemia–reperfusion injury, nonsteroidal antiinflammatory drug–induced damage, and diet-responsive diarrhea.41,42 However, intestinal permeability testing was not useful as an indicator of clinical disease activity in dogs with chronic enteropathies.38 There is currently a lack of standardization for the various methods used for the assessment of intestinal permeability among laboratories. Furthermore, only few studies have been performed in clinical patients, and the interpretations of the results of GI permeability testing have not been well elucidated for routine clinical settings.
Evaluation of Intestinal Protein Loss
Protein-losing enteropathy (PLE) is defined as a heterogeneous group of diseases in which plasma proteins are lost into the GI lumen. Any GI disease, if severe enough, can lead to intestinal protein loss. Common causes for PLE include inflammatory enteropathies (e.g., inflammatory bowel disease [IBD]), infectious enteritis (bacterial, parasitic, or fungal enteritis), lymphangiectasia, intestinal neoplasia (e.g., lymphoma), intussusception, immunoproliferative disease, and small intestinal dysbiosis.43 Although most animals with GI protein loss display panhypoproteinemia, it is not uncommon to encounter patients with hypoalbuminemia but without hypoglobulinemia. This is a result of an increased globulin production caused by concurrent or underlying disease (e.g., histoplasmosis, ehrlichiosis, chronic skin disease, immunoproliferative small intestinal disease in the Basenji). Therefore, it is crucial to determine serum albumin concentrations in addition to total plasma protein. Other causes of protein loss such as protein-losing nephropathy and hepatic insufficiency must be excluded.43
ELISAs for the measurement of fecal α1-PI (α1-proteinase inhibitor) have been validated for cats and dogs and are useful in assessing protein loss through the GI tract.44,45 α1-PI is a serum proteinase inhibitor, and is present in the lumen of the GI tract in trace amounts. α1-PI has a similar molecular mass (approximately 60 kDa) to albumin. When GI disease is severe, α1-PI is lost together with albumin into the lumen. Albumin is usually rapidly degraded by proteolytic enzymes, but α1-PI is a proteinase inhibitor that is resistant to proteolytic enzymes and can be measured in feces as an intact molecule. α1-PI serves as an indicator of loss of plasma proteins into the GI tract.
Other methods for the measurement of enteric protein loss involve determining the recovery of intravenously administered radioactively labeled substances (e.g., 51Cr-albumin) in fecal samples.46 Although considered the gold standard, those tests have the obvious disadvantage of the use of radioactivity.
Minimally Invasive Markers for Assessment of Gastric and Intestinal Inflammation and Damage
Serum Gastrin
A commercially available chemiluminescence immunoassay for use in humans (Immulite 2000, Siemens Diagnostics) has been validated for measurement of serum gastrin concentrations in dogs and cats.47 Serum should immediately be separated from blood cells, frozen, and shipped on ice. The measurement of serum gastrin is useful in cats and dogs with unexplained gastric erosion or ulceration and mucosal hyperplasia. A several-fold and persistent increase (multiple consecutive samples should be evaluated after a 12- to 24-hour fast) in serum gastrin concentration in animals with concurrent clinical signs is suggestive of a gastrinoma. Normal fasting serum gastrin concentration makes gastrinoma unlikely. If serum gastrin is not markedly increased and a gastrinoma is suspected, a secretin stimulation test can be performed. However, it should be noted that, at least in dogs, the administration of a proton pump inhibitor can lead to massive increases of serum gastrin concentrations. Thus, such medication should be discontinued for at least 10 days before measurement of serum gastrin concentration,
Inflammatory Markers
Fecal calprotectin (also called calgranulin A/B or S100A8/A9) and calgranulin C (S100A12) have been reported to be increased in serum and/or plasma in human patients with various inflammatory disorders and are also sensitive and specific fecal markers for human IBD. Species specific assays for dogs have been developed and show promise for the assessment of intestinal inflammation in this species.48,49 The clinical utility of these assays is under investigation. N-methylhistamine is a stable metabolite of histamine and has been used as a marker for mast cell activity and systemic release of histamine in human IBD. The use of N-methylhistamine in dogs and cats as a marker for GI disease is currently under investigation.
C-reactive protein (CRP) is a sensitive but nonspecific inflammatory marker and has been shown to correlate with the degree of experimentally induced damage to the gastric mucosa and with the clinical activity index in canine IBD.50,51 New studies have evaluated the index of variability for CRP, suggesting that the use of population-based reference range is not appropriate for evaluating changes in CRP concentrations in individual patients (Ruaux CG, research abstract, ACVIM Forum 2010). Therefore, for assessment of the severity of GI disease, serial CRP measurements should be performed, and modest changes in serum CRP concentrations may indicate of the severity of GI disease.
Citrulline
Citrulline is an amino acid that is synthesized mostly by intestinal enterocytes and also to some degree by hepatocytes. Citrulline has been used as a marker of functional enterocyte metabolic mass. For example, human studies have demonstrated a correlation between fasting plasma citrulline concentrations and the functional length of the small intestine in patients with short bowel syndrome.52 Plasma citrulline concentration has been shown to be significantly decreased in dogs with parvovirus enteritis, indicating that citrulline is a potential marker for spontaneous acute and severe intestinal failure.53
Occult Blood Testing
Fecal occult blood tests are used to detect blood in stools before visible melena occurs. Several assays are commercially available and are based on different test principles (e.g., guaiac or o-toluidine fecal occult blood tests). Their clinical use in dogs and cats is limited as they have poor sensitivity and specificity. Because the assay is not specific for a patient’s hemoglobin, but can also react with any dietary hemoglobin, false-positive test results occur frequently. Therefore, to increase the specificity of occult blood testing, patients must ideally be on a meat-free diet for at least 3 days before fecal samples are collected.54 However, it should be noted that the effect of diet is much smaller on tests based on o-toluidine when compared with those based on guaiac. Tests performed on three consecutive bowel movements may help to avoid false-negative results. Manual stool collection may cause iatrogenic blood contamination.
Miscellaneous Tests
An increased plasma L-lactate concentration (>6.0 mmol/L) has been shown to be a negative prognostic marker for postoperative survival time in dogs following gastric dilation volvulus.55 Serum pepsinogen concentrations have been measured in humans to assess gastric inflammation and H. pylori infection. However, serum pepsinogen and serum gastric lipase concentrations were not useful in detecting gastric lesions in dogs or cats.56
Evaluation of Gastrointestinal Motility
The assessment of GI motility remains challenging, mostly because of the lack of practical and reproducible minimally invasive test procedures.57 Scintigraphy is considered the gold standard for evaluation of gastric emptying but requires the use of a radioactive probe. Alternatively, radiopaque markers, such as barium-impregnated polyethylene spheres (BIPSs), have been introduced as an alternative. Both these markers have the disadvantage that, depending on their size, they only mimic emptying of either solid or liquid food.58 Ultrasonography is a reliable and quantitative method for assessing the emptying time of liquids and solids when performed by an experienced ultrasonographer. A minimally invasive method to assess gastric emptying time in dogs and cats is the 13C-octanoic acid breath test.59 This test allows labeling of a complex meal with 13C-octanoic acid, a medium-chain fatty acid that is absorbed in the duodenum. In liver 13C is released and oxidized. A rise in 13CO2 in the expiratory air or in blood indicates that gastric emptying has occurred.
The use of a wireless capsule (SmartPill), originally designed for use in humans, has been reported for assessment of GI motility in dogs.60 The capsule measures 13 × 26 mm and is given orally to dogs. The capsule records pH, pressure, and temperature along its passage through the GI tract. These parameters allow calculation of gastric emptying time, small intestinal and/or colonic transit time, and also the total GI transit times. This system appears reproducible in dogs, and changes in GI motility in response to dietary modification and motility altering drugs have been demonstrated. Because of the size of the capsule, it leaves the stomach at the onset of the interdigestive migrating motor complexes (MMCs) after all liquids and solids have already been propelled into the small intestine. Because of the size of the capsule, this method cannot be used in cats and only in dogs of more than 15 kg body weight.
Assessment of Intestinal Function and Pathology
Serum Cobalamin and Folate
The uptake of cobalamin (vitamin B12) and folate from the small intestine is dependent on several factors and can therefore be used as an indirect marker for the assessment of GI disease. Disorders that may affect serum cobalamin and/or folate concentrations include small intestinal inflammation, exocrine pancreatic insufficiency (EPI), and small intestinal dysbiosis (also referred to as small intestinal bacterial overgrowth [SIBO] or antibiotic-responsive diarrhea [ARD]). As a consequence of different sites of absorption, specific changes in serum cobalamin and folate concentrations can yield information on the localization and potentially the severity of intestinal disease (Table 25-3). Serum concentrations of cobalamin and folate should be assessed in any dog or cat with clinical signs of GI disease.
Table 25-3 Interpretation of Serum Cobalamin and Folate Concentrations in Dogs and Cats with Gastrointestinal Diseases
Serum Folate | Serum Cobalamin | |
---|---|---|
Increased | Dogs: small intestinal bacterial overgrowth | Clinical significance unknown in dogs and cats |
Cats: clinical significance unknown | Recent parenteral administration (<4 weeks) of cobalamin | |
Increased dietary intake of folic acid | ||
Vitamin supplementation | ||
Decreased | Proximal or diffuse small intestinal disease (e.g., IBD, lymphoma, fungal disease) | Distal or diffuse small intestinal disease involving the ileum (e.g., IBD, lymphoma, fungal disease) |
Small intestinal bacterial overgrowth | ||
Exocrine pancreatic insufficiency | ||
Hereditary cobalamin malabsorption | ||
Low dietary intake (vegetarian diet) |
Cobalamin
The physiologic mechanism of cobalamin absorption is complex and requires a functioning digestive system (Fig. 25-7). Dietary cobalamin is bound to animal-based protein. After digestion of these carrier proteins in the stomach, the cobalamin is immediately bound to R-protein, a cobalamin transporter secreted in saliva and gastric juice. Pancreatic enzymes (i.e., trypsin and chymotrypsin) digest the R-protein in the small intestine, and the free cobalamin is bound by intrinsic factor. The exocrine pancreas is a major source of intrinsic factor in dogs and appears to be the exclusive source of intrinsic factor in cats.61 This is in contrast to humans where intrinsic factor is produced predominantly in the stomach. Intrinsic factor/cobalamin complexes are then absorbed by specific mucosal receptors located in the ileum.
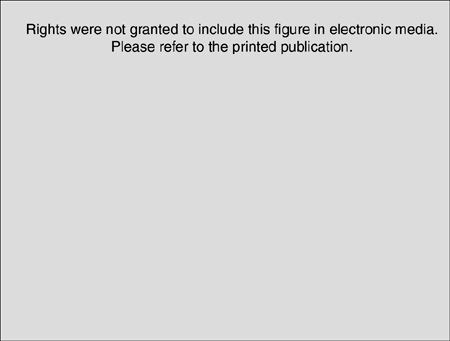
Figure 25-7 Absorption of dietary cobalamin.
(Reprinted from Suchodolski J, Steiner J. Laboratory assessment of gastrointestinal function. Clin Tech Small Anim Pract 18:207, 2003. With permission from Elsevier.)
Major disorders that interfere with cobalamin uptake are EPI, distal or diffuse small intestinal disease, and excess bacterial utilization of cobalamin in bacterial dysbiosis. Aberrations in the small intestinal microbiota may lead to increased competition for cobalamin resulting in decreased absorption by the host. Bacteria in the gut may compete with receptors located in the distal small intestine and prevent cobalamin uptake by enterocytes. Bacteroides spp. are the principal organisms involved because they can utilize cobalamin-intrinsic factor, whereas other bacteria can only bind free cobalamin, which is present in lower concentrations in the gut. The reported sensitivity of serum cobalamin for the diagnosis of SIBO is 25% to 55%.62
Abnormalities of both vitamins are common in dogs and cats with EPI. Therefore, EPI should be ruled out in patients with GI signs and a decreased serum cobalamin concentration. Serum cobalamin must bind to intrinsic factor in order to be absorbed by receptors located in the distal ileum. Because the exocrine pancreas is the exclusive source of intrinsic factor in cats and the main source in dogs, EPI will lead to decreased secretion of intrinsic factor and, therefore, decreased uptake of cobalamin. Cats with EPI almost always have subnormal serum cobalamin concentrations. Similar subnormal cobalamin concentrations have been described in excess of 80% in dogs with EPI.63
The measurement of serum concentrations of cobalamin is only an indirect test for assessing cobalamin deficiency. Cobalamin deficiency leads to accumulation of serum or urine methylmalonic acid (MMA), the concentrations of which are often dramatically increased in patients with cobalamin deficiency and have been shown to decrease with cobalamin supplementation.64 It has also been shown that some patients with low normal serum cobalamin concentrations have increased MMA concentrations, indicating cobalamin deficiency on a cellular level.64 Therefore, cobalamin deficiency may even be present when serum cobalamin concentration is in the low end of the reference interval (<350 ng/L) and parenteral cobalamin supplementation should be considered in patients with compatible clinical signs. The measurement of MMA concentration is technically challenging and currently only available through few laboratories. Very young puppies (up to 13 weeks) have been shown to have lower serum cobalamin concentrations than adult dogs. Also, a hereditary form of cobalamin deficiency has been reported in both dogs and cats, and affected animals present usually at a young age (6 to 12 weeks).65
Folate
Folate is a water-soluble vitamin that, similar to cobalamin, is abundant in canine and feline diets, making nutritional deficiency unlikely. Dietary folate is typically present in the poorly absorbable polyglutamate form. In the proximal small intestine, the brush-border enzyme folate deconjugase removes all but one glutamate residue from the molecule (Fig. 25-8). The uptake of folate monoglutamate occurs through specific carriers located in the proximal small intestine. Folate uptake is increased at a slightly acidic pH. Because erythrocytes contain high concentrations of folate hemolysis may lead to falsely increased serum folate concentrations.
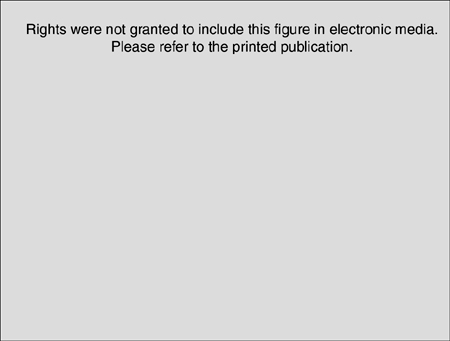
Figure 25-8 Absorption of folate.
(Reprinted from Suchodolski J, Steiner J. Laboratory assessment of gastrointestinal function. Clin Tech Small Anim Pract 18:208, 2003. With permission from Elsevier.)
In dogs increased serum folate concentrations can be indicative of small intestinal dysbiosis (SIBO/ARD). Resident bacteria in the distal small intestine (i.e., ileum) and in the large intestine can produce large quantities of folate. Because the carriers responsible for folate uptake are located exclusively in the proximal small intestine, folate produced in distal sections of the intestine cannot be absorbed. However, if folate-producing bacteria proliferate in the proximal small intestine, the bacterial folate can be absorbed by the host resulting in increased serum folate concentrations. The reported sensitivity of serum folate for the diagnosis of SIBO in dogs ranges from 50% to 66%, while no such data are available for cats.62 Increases in serum folate concentrations are also often observed in dogs with EPI. Reduced pancreatic secretions, which normally help to suppress bacterial colonization in the small intestine, may lead to bacterial overgrowth in the small intestine. In contrast to dogs, cats with EPI often have a concurrent chronic enteropathy that may damage folate receptors in the proximal small intestine, leading to decreased serum folate concentrations.
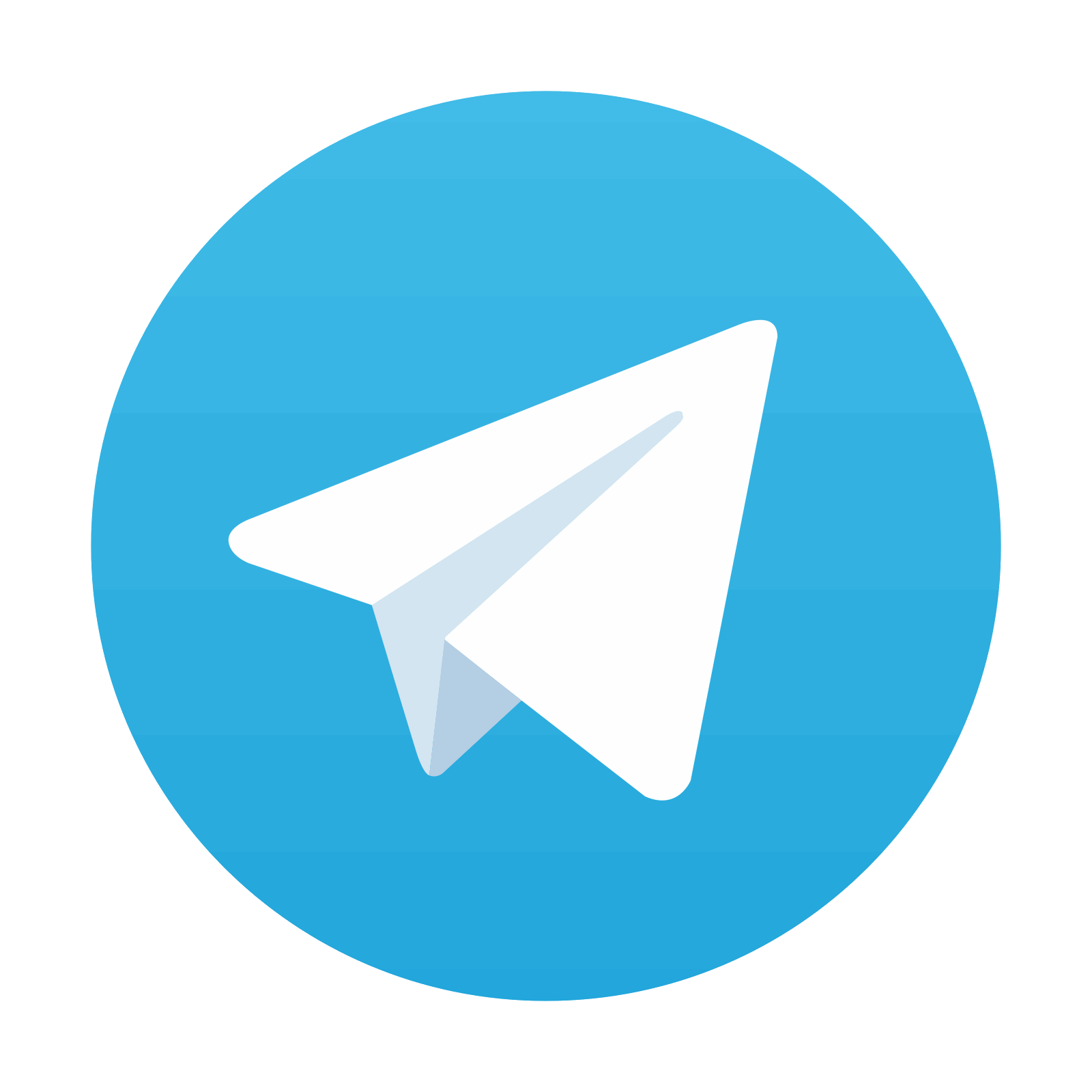
Stay updated, free articles. Join our Telegram channel

Full access? Get Clinical Tree
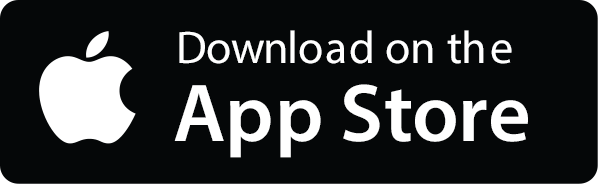
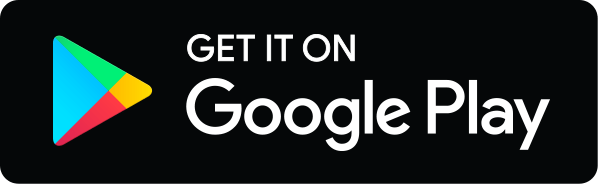