Chapter 1 Introduction to cardiac anatomy and physiology
ANATOMY OF THE HEART AND GREAT VESSELS(
ET)
The heart can be regarded as a parallel pump system: deoxygenated blood returning from the body enters the right side from where it is directed via the pulmonary arterial system to the lungs for oxygenation. Oxygenated blood returns to the left side of the heart via the pulmonary veins and is then pumped to the body via the systemic arterial system. Deoxygenated blood returns via the systemic veins to the right side. The heart is located within the middle mediastinal space where its long axis is orientated at approximately 10° to vertical with its base lying dorsal and cranial to the apex. The apex is located above the last sternebra cranial to the sternal portion of the diaphragm. The heart consists of two atria and two ventricles, blood entering via the atria and leaving via the ventricles. The right atrium (RA) occupies the cranial part of the heart base and consists of two main parts, the larger part, the sinus venarum cavarum, into which the veins empty, and a conical out-pouching, the auricle. The auricle is triangular and broad-based and curves around the base of the heart towards the left ending cranial to the origin of the main pulmonary artery (Figs. 1.1 and 1.2). The cranial vena cava (draining structures of the head and neck) enters the most dorsal part of the RA, the caudal vena cava (draining abdominal structures) opens into the caudal part and the azygous vein (draining the caudal thorax) enters between the two cavae. The coronary sinus (draining the coronary circulation) opens into the RA ventral to the caudal vena cava. There are also several smaller veins that drain directly into the RA (see Figs. 1.1 and 1.2). On the internal surface of the RA there are pronounced ridges formed by extensive bands of pectinate muscles and dorsally these form the terminal crest at the base of the auricle (Fig. 1.3). The oval fossa is a diverticulum at the point of entrance of the caudal vena cava that is a remnant of the foramen ovale, the communication that exists between the two atria in the fetus.
The right atrioventricular (AV) or tricuspid valve forms the ventral floor of the RA and the entrance to the right ventricle (RV) (see Figs. 1.1 and 1.2). As its name suggests, the tricuspid valve is composed of three large leaflets: one is septal, one lies on the right margin (parietal) and the third lies between the AV opening and the right outflow tract (angular). The leaflets are anchored to the papillary muscles of the RV by a series of chordae tendineae. The RV is a crescent-shaped structure in cross-section and triangular when viewed from its inner aspect (see Fig. 1.2). It wraps around the cranial aspect of the heart and, in this respect, the convention derived from human anatomy ascribing the terms right and left to the heart, is rather misleading. In the horse, the heart would be better defined as having cranial and caudal components. The internal surface of the RV is trabeculated and moderator bands cross the lumen of the RV from the septum to the opposite wall carrying conduction tissue (see Fig. 1.3). These moderator bands vary in size greatly among individuals. Ventrally, the RV does not reach the heart’s apex. It extends dorsally and to the left to form the right outflow tract leading to the main pulmonary artery (PA) via the pulmonary valve (right semilunar valve) valve (see Fig. 1.3). The pulmonary valve consists of three half-moon-shaped cusps, the right, left and intermediate, which occasionally have small fenestrations along their free edges and are attached to a fibrous ring at the base of the pulmonary artery. The PA arises from the left side of the RV and curves dorsally, caudally and medially to run under the descending aorta where it branches into left and right. The right PA passes over the cranial part of the left atrium and under the trachea while the left PA is in contact with the bulk of the dorsal surface of the left atrium (LA) (see Fig. 1.2).
The LA forms the caudal part of the base of the heart and also has an auricle, extending laterally and cranially on the left side. The left auricle is more pointed than the right auricle and lacks a terminal crest (see Fig. 1.2). The LA lacks the extensive pectinate muscles that characterize the RA. Seven or eight pulmonary veins enter the LA around its caudal and right aspects. A depression may be appreciated on the septal surface, corresponding with the site of the fetal foramen ovale. The ventral floor of the LA consists of the left AV, mitral or bicuspid valve (see Figs. 1.1 to 1.3). This consists of two large leaflets, the septal and parietal leaflets which are larger and thicker than those of the tricuspid valve. The left ventricle (LV) is conical with walls approximately three times thicker than those of the RV, and it forms the bulk of the caudal aspect of the heart including the apex. The portion of the wall that forms the division between the LV and RV is called the interventricular septum while the remainder is termed the free wall. Arising from the free wall are two large papillary muscles that are symmetrically located to the left and right sides of the free wall and anchor the chordae tendineae of the mitral valve (see Fig. 1.2). The interventricular septum (IVS) is mainly composed of muscular tissue, but at its most dorsal extent the membranous or nonmuscular septum is thinner and composed of more fibrous tissue. The left ventricular outflow tract lies in the centre of the heart and the aortic (left semilunar) valve consists of three half-moon-shaped cusps that are stronger and thicker than those of the pulmonary valve. The free edges often contain central nodules of fibrous tissue but may also have fenestrations. The cusps are attached to the fibrous and cartilaginous tissues that comprise the aortic annulus. The proximal segment of the aorta is the ascending aorta; it sweeps dorsally and cranially between the main PA on the left and the RA on the right. It then continues caudally and to the left as the descending aorta. The base of the aorta is bulbous in shape, and this bulbous portion is the sinus of Valsalva. The sino-tubular junction marks the point where the vessel becomes more tubular (see Figs. 1.1 and 1.3). To provide the blood to the myocardium, two coronary arteries arise from the right and left sinus of Valsalva. The most caudally located third part of the sinus lacks a coronary artery and is termed the septal (noncoronary) sinus. The same terminology is applied to the cusps of the aortic valve (see Fig. 1.2). The ligamentum arteriosum can be found in the site corresponding to the remnant of the ductus arteriosus, a vessel joining the PA to the descending aorta in the fetus (see Fig. 1.3).
The heart lies within the pericardial cavity that is comprised of the parietal pericardium and visceral pericardium (epicardium). The parietal pericardium attaches to the tunica externa of the proximal aorta, pulmonary artery, vena cavae and pulmonary veins. Between the parietal pericardium and visceral pericardium (epicardium) is a thin film of free serous fluid (pericardial fluid). The ventricular myocardium consists of muscle layers arranged both longitudinally and also spiralling circumferentially. The muscular tissue of the atria is separated from that of the ventricles by a fibrous skeleton that surrounds the atrioventricular orifices. The myocardium receives its blood supply from the coronary arteries and veins. There is an extensive autonomic nervous supply to the heart from the vagus nerve and sympathetic trunk (see Chapter 2).
ELECTRICAL PROPERTIES OF THE HEART
From the SA node, the impulse spreads over the atria to the AV node producing electrical potentials that inscribe a P wave on the surface electrocardiogram (ECG). Since spread of the cardiac impulse through the atria is in an overall direction that is dorsal to ventral, P waves are typically positive in a base apex ECG recording (see Chapter 6). The impulse is then conducted slowly through the AV node producing a delay recognized by an isoelectric segment (PR segment) on the ECG. The degree of AV conduction delay is influenced by autonomic tone with vagal tone reducing and sympathetic tone increasing rate of conduction. Autonomic tone, therefore, becomes an important determinant of heart rate in horses with dysrhythmias such as atrial fibrillation.
After relatively slow conduction through the AV node, the cardiac impulse is rapidly conducted over the bundle of His and Purkinje system to the terminal Purkinje fibres and the working ventricular myocytes. The equine Purkinje system is widely distributed throughout the right and left ventricular myocardium, penetrating the entire thickness of the ventricular walls. This vast distribution of the Purkinje system is physiologically important because the conduction velocity of working ventricular myocytes is approximately 6 times slower than conduction velocity of the Purkinje cells. Consequently, the time duration and sequence of ventricular activation and, ultimately, the surface ECG is affected. Specifically, the earliest phase of ventricular activation in horses consists of depolarization of a small apical region of the septum (Fig. 1.4). This early depolarization is often in an overall left to right and ventral direction. The electrical potentials generated from this early phase of ventricular activation may produce the initial portion of the QRS complex on the surface ECG. However, there is significant variation in the direction of this early phase of ventricular activation, and in some horses the vectors of local electrical activity effectively cancel each other thereby eliminating any deflection on the surface ECG. Thus, the duration of QRS complexes in normal horses may vary from 0.08 to 1.4 seconds. Immediately after early ventricular activation of the apical portion of the septum, the major masses of both ventricles and the middle portion of the septum are depolarized with a single “burst” of activation that results from the vast distribution and penetration of the Purkinje fibres. Since this depolarization occurs without a spread of the impulse in any specific direction it contributes negligibly to genesis of the QRS complex on the ECG. The final phase of equine ventricular activation consists of depolarization of the basilar third of the septum, which occurs in an apical to basilar direction. This final phase of activation is responsible for generating most of the QRS complex and normally produces a negative deflection in a base apex recording (see Fig. 1.4).2,3
THE CARDIAC CYCLE
The cardiac cycle describes and relates temporally the mechanical, electrical and acoustical events that occur in the heart and great vessels. The cardiac cycle is usually described from onset of systole to end of diastole. It is helpful to describe the cardiac cycle with a diagram showing time on the horizontal axis (Fig. 1.5). An understanding of the cardiac cycle is essential for understanding function of the normal heart and for an appreciation of how various diseases disturb normal function.
< div class='tao-gold-member'>
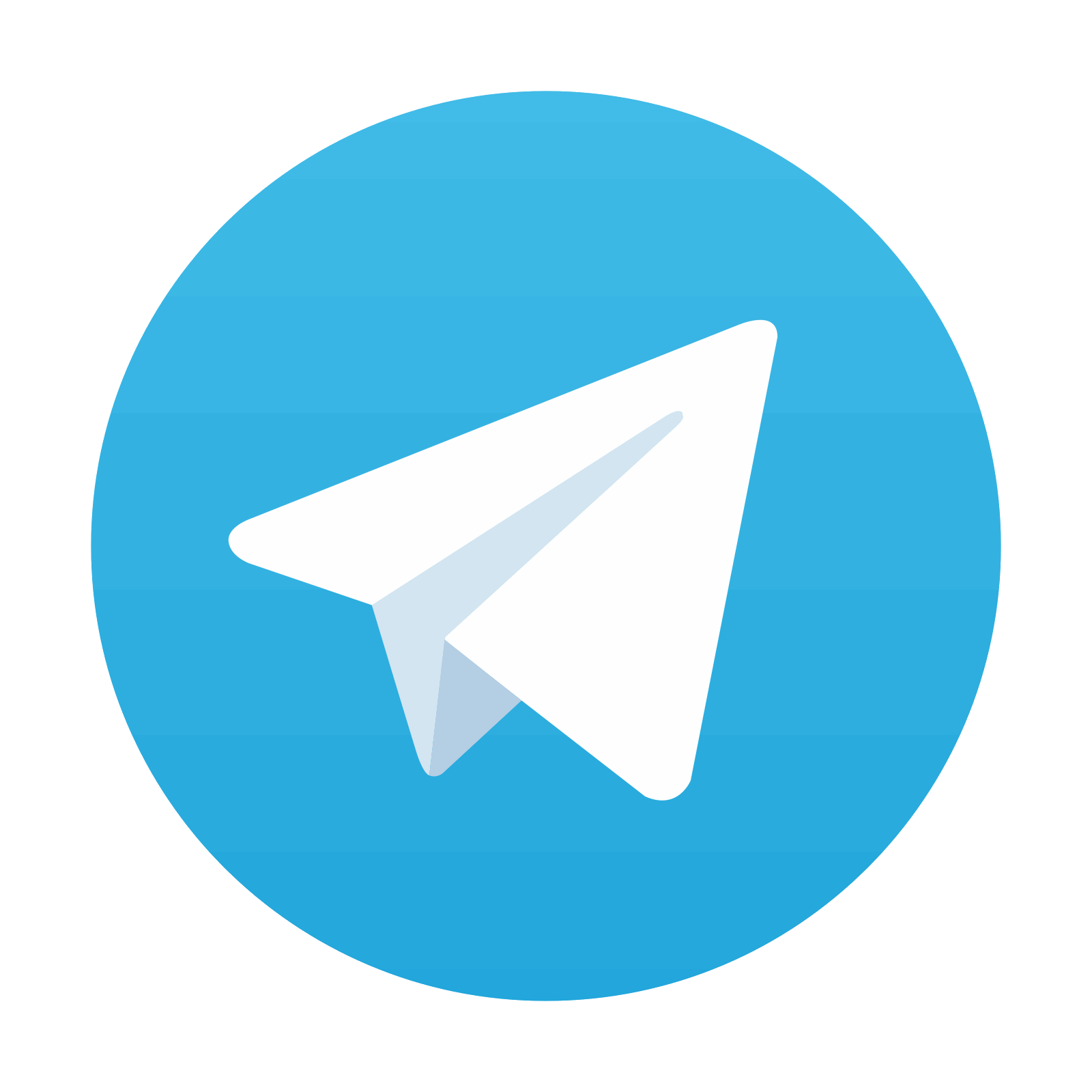
Stay updated, free articles. Join our Telegram channel

Full access? Get Clinical Tree
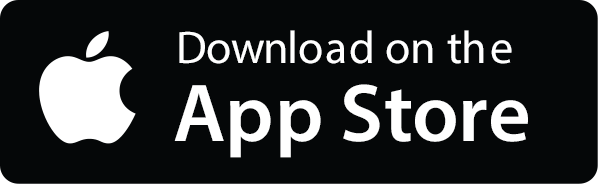
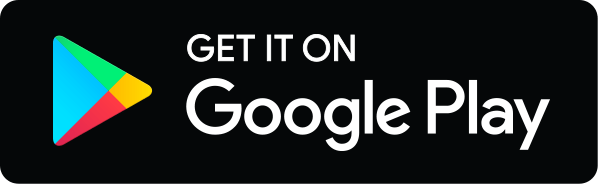