Mutants
Ataxic or not
Stationary beam
Suspended string
Inclined grid
Rotorod
References
Grid2 Lc
Ataxic
↓
↓
↓
↓
Grid2 ho-Nancy
Ataxic
↓
↓
?
↓
Grid2 ho-4J
Ataxic
↓
Normal
?
↓
(14)
Rora sg
Ataxic
↓
↓
?
↓
Agtpbp1 pcd
Ataxic
Normal
↓
Normal
↓
(87)
Nervous
Ataxic
↓
↓
↓
↓
(82)
Pogo
Ataxic
↓
?
?
?
(84)
Reln rl-Orl
Ataxic
↓
↓
?
↓
(83)
Cacna1α la het
Non-ataxic
?
↓
?
↓
(92)
Cacna1α rol het
Non-ataxic
↓
↓
?
↓
(90)
Dst dt
Ataxic
↓
↓
↓
?
(85)
FRDA KO
Ataxic
?
?
?
↓
(55)
Calb1 KO
Non-ataxic
↓
?
?
?
Unc13c KO
Non-ataxic
?
?
?
↓
(73)
Hfz KO
Ataxic
?
↓
?
↓
(76)
Plcb4 KO
Ataxic
?
?
?
↓
(77)
ATXN1/Q82 Tg
Ataxic
↓
?
?
↓
(57)
Atxn1/Q78 KI
Non-ataxic
?
?
?
↓
(59)
ATXN2/Q75 Tg
Non-ataxic
?
?
?
↓
(60)
ATXN3/Q84 Tg
Ataxic
↓
?
?
?
(88)
ATXN3/Q71 Tg
Ataxic
?
?
?
↓
(64)
ATXN3/Q148 Tg
Non-ataxic
?
?
?
↓
(65)
Spnb3 KO
Ataxic
↓
Normal
?
↓
(66)
ATXN7/Q92 Tg
Ataxic
?
?
?
↓
(68)
ATXN7/Q90 Tg
Non-ataxic
?
?
?
↓
(69)
ATXN7/Q52 Tg
Ataxic
?
?
?
↓
(70)
Atxn8os KO
Non-ataxic
?
?
?
↓
(71)
3.1 Stationary Beam
In the stationary beam test, the animal either stays put or locomotes along the beam, usually closed at either end to prevent escapes. Distance traveled and number of slips are used as auxiliary measures to distinguish immobile from moving subjects. The beam should be placed at least at a height of 30 cm from a padded surface, to prevent temptations of jumping off deliberately and any type of injury. For optimal performances, the beam should be covered with masking tape or some other material to increase traction. The beam is usually circular, since square-shaped ones facilitate clinging to the side.
The stationary beam test is sensitive to natural mutations with ataxia, as seen in Grid2 Lc (78, 79), Grid2 ho-Nancy (14, 79), Grid2 ho-4J (14), Rora sg (79–81), nervous (82), Reln rl-Orl (83), pogo (84), and Dst dt-J (85, 86), as well as non-ataxic Cacna1α rol heterozygotes (87). When a thick square-shaped beam was substituted for a round one to facilitate clinging against the side, Rora sg mutants were still impaired (86), but not Grid2 Lc (78) or Agtpbp1 pcd-1J (87). Thus, a partial loss of Purkinje cells in Rora sg caused a worse outcome than their nearly total disappearance in the other two mutants, presumably because the presence of the remaining cells causes worse dysfunction than their absence.
The stationary beam test is also sensitive to cerebellar anomalies in genetically modified mutants, such as ATXN3/Q84 ataxic transgenics (88), as well as Spnb3 (66) and Calb1 (75, 89) KOs, as well as non-ataxic Cacna1α heterozygotes (90), in addition to ATXN1/Q82 transgenics prior to ataxia (57). The bar deficit in the ATXN3/Q84 transgenic was alleviated with dantrolene, a calcium stabilizer (88), indicating its sensitivity to pharmacologic intervention.
3.2 Suspended Wire or Coat-Hanger
In the wire suspension test, the animal either stays put or locomotes upside down while clinging to a thin metal or plastic wire closed at either end. Because the animal must carry its own body weight, this test is more dependent on muscle strength than the previous one. To obtain measurements of movement time and climbing abilities, the animal may reach and then climb at either end on a diagonal wire of a coat-hanger. As with beam tests, the horizontal wire should be placed at least at a height of 30 cm from a padded surface. Despite cerebellar damage, the animal may land on four feet, or on its side, as the air-righting reflex is still active. Some cerebellar mutants are susceptible to convulsions when placed upside down. In such cases, the animal’s fall should not count. Instead, the animal should rest awhile and the trial repeated after recovery.
Latencies before falling from the coat-hanger were lower than normal in natural mutations with ataxia, such as Grid2 Lc (78, 79), Grid2 ho-Nancy (79), Rora sg (79–81), Agtpbp1 pcd-1J (87), Reln rl-Orl (83), and Dst dt-J (85, 86). Latencies before falling were also lower in Grid2 Lc mutants placed on the suspended wire (91). On the contrary, ataxic nervous mutants did not fall sooner than controls, probably helped by relatively preserved Purkinje cells at the level of the vermis, crucial in the control of posture and equilibrium, though their movement times were longer (82). However, non-ataxic Cacna1α rol (90) and Cacna1α ln (92) heterozygotes were deficient in the wire suspension test, though ataxic Spnb3 (66) and Hfz (76) KOs were not. Thus, on one hand, ataxia does not necessarily lead to deficits in hanging from a wire. On the other, some non-ataxic mutants are deficient, indicating no obligatory relation between the two signs.
3.3 Inclined or Vertical Grid
In the inclined or vertical grid test, the animal is placed on a metal surface oriented from 0 to 180°. As with the previous test, the grid should be placed at least at a height of 30 cm from a padded surface. In comparison to non-ataxic controls, the number of falls from a vertical grid increased in Grid2 Lc (78), Rora sg (81), and Dst dt-J (85, 86) mutants, but not in Agtpbp1 pcd-1J (87). As noted above, the superior performance of Agtpbp1 pcd-1J relative to Rora sg on the same grid may be explained by nearly total as opposed to partial Purkinje cell depletion, the former being more favorable to behavioral performances. Their superior performance relative to Grid2 Lc may be ascribed to later onset of Purkinje cell depletion or to relative preservation of cerebellar granule cells. Their superior performance relative to Dst dt-J is attributed to the latter’s spinocerebellar and lemniscal atrophy leading to crawling in addition to ataxia.
3.4 Rotorod
Unlike the above-named tests, animals placed on the rotorod move in synchrony with a moving surface. An animal with postural deficits but with preserved muscle strength may cling to the rotating beam without moving, though cerebellar lesions usually cause hypotonia. To distinguish moving from immobile animals, the latter may be removed from the beam after turning twice around, as if it fell, since passively rotated animals tend to repeat this strategy until the end of testing. A more time-consuming approach is to videotape and measure time spent in passive rotation. In most models, the rod is placed lower than 30 cm above an unpadded surface, since animals are less inclined to jump away from a moving beam. However, it is judicious to start the test at the slow pace of 4 revolutions per minute (rpm), accelerating up to 40 rpm, because at faster initial speeds animals tend to jump off deliberately. If the beam is not rotated at the start, animals sometimes fall off immediately when surprised by the first jerk of beam movement.
The rotorod test is sensitive to natural mutations with ataxia, as seen in Grid2 Lc (91, 93–95), Grid2 ho-Nancy (14, 95), Grid2 ho-4J (14), Rora sg (80, 95), Agtpbp1 pcd-1J (87), nervous (82), and Reln rl-Orl (83), as well as non-ataxic Cacna1α la (92) and Cacna1α rol (90) heterozygotes.
The rotorod test is also sensitive to cerebellar anomalies in genetically modified mice. ATXN1/Q82 transgenic mice were impaired on the rotorod as early as 5 weeks of age, prior to signs of ataxia, when the main histopathology is the accumulation of cytoplasmic vacuoles inside Purkinje cells (57). Moreover, non-ataxic Atxn1/Q78 KI mice were impaired in the same test (59). Rotorod performance improved in the conditional ATXN1/Q82 model with delayed transgene expression relative to the original transgenic (58). After stopping transgene expression in the conditional model, rotorod performance was also ameliorated (96). Non-conditional ATXN1/Q82 transgenics crossbred with mice overexpressing the inducible form of rat HSP70, encoding a molecular chaperone involved in protein folding, had Purkinje cells with thicker and more arborized dendritic branches than the single transgenic and better rotorod performance (97). Rotorod performance also improved after RNA interference (98), intranasal insulin-growth factor (99) or injections of taurine-conjugated ursodeoxycholic acid (100). As with the SCA1 model, FRDA null mutants were impaired on the rotorod prior to ataxia (55).
Other ataxic mutants showing impairments on the rotorod include ATXN3/Q71 (64), ATXN7/Q92 (68), and ATXN7/Q52 (70) transgenics, as well as Spnb3 (66), Atxn8os (71), Hfz (76), and Plcb4 (101) KOs. Moreover, non-ataxic ATXN2/Q75 (60), ATXN3/Q148 (65), and ATXN7/Q90 (69) transgenic mice, as well as Unc13c (73) KOs mice were deficient on this test.
4 Exploratory Activity
Motor activity and spontaneous alternation tests (Table 2) are relevant to cerebellar pathology. Motor activity in the open-field and T- or Y-mazes provides an estimate of motor capabilities as well as general arousal levels. Spontaneous alternation testing provides an estimate of the motivation to explore unfamiliar environments.
Table 2
Exploratory activity by cerebellar or spinocerebellar mutants vs. controls
Mutants | Ambulatory activity | Spontaneous alternation | References |
---|---|---|---|
Grid2 Lc | ↑ | ↓ | (105) |
Grid2 ho-Nancy | ↓ | ↓ | (109) |
Grid2 ho-4J | Normal | ? | (108) |
Rora sg | ↓ | ↓ | (104) |
Agtpbp1 pcd | ↑ | ↓ | (106) |
Nervous | ↑ | ↓ | (107) |
Reln rl-J | ? | ↓ | (114) |
Reln rl-Orl | Normal | ↓ | (83) |
Dst dt | ↓ | ? | (85) |
ATXN1/Q82 Tg | ↑ | ? | (57) |
ATXN3/Q71 Tg | ↓ | ? | (64) |
4.1 Open-Field and T-Maze Activity
When evaluated for distance travelled in a T-maze, Rora sg mutants (104) were hypoactive relative to non-ataxic controls, due at least in part to ataxia and frequent falls, especially after attempted rears against the walls. But despite ataxia and stumbling, Grid2 Lc (105), Agtpbp1 pcd-1J (106), and nervous (107) mutants were hyperactive in the same maze relative to their respective controls. Non-ataxic ATXN3/Q148 mutants were likewise hyperactive in the open-field (65), as well as ATXN1/Q82 mutants prior to ataxia (57). The lower activity of Rora sg relative to Grid2 Lc and Agtpbp1 pcd-1J reproduces their profile on the square-shaped stationary beam, only Rora sg being deficient (78, 81, 97). Thus, activity levels in cerebellar mutants gauge the extent of motor deficits in a complementary manner to tests measuring balance and equilibrium. Exploratory activity was normal in ataxic Reln rl-Orl (83) and Grid2 ho-4J (108) mutants, though the Grid2 ho-Nancy mutants were hypoactive (109), perhaps indicating an allele-specific deficit. ATXN3/Q71 transgenic mice with cerebellar and basal ganglia dysfunction were also hypoactive (64), as well as the Hfz KO (76) and Dst dt-J mutants (85), the latter result being expected due to their crawling phenotype.
4.2 Spontaneous Alternation
After entering one of two maze arms in a T- or Y-maze, rodents have a strong tendency to enter the opposite side (110). The spontaneous alternation test is sensitive to natural mutations with cerebellar atrophy, as shown in Grid2 Lc (105, 111, 112), Grid2 ho-Nancy (109), Rora sg (104, 113), Agtpbp1 pcd-1J (106), nervous (82), Reln rl-Orl (83), and Reln rl-J (114). However, these results did not extend to the ataxic Hfz KO (76). In general, the results are concordant with a role for the cerebellum in disinhibitory tendencies, as seen in animals with damage to the vestibular system (115), the limbic system (116, 117), and the prefrontal cortex (118), attributable to two-way vestibulo–cerebello–cortical connections. The gross motor control implied in turning right or left precludes involuntary entries into a maze arm as the main reason for their perseveration.
5 Spatial Orientation in Water Mazes
The lurching gait so characteristic of Grid2 Lc mutants on dry ground is no longer evident in the water, as these show good swimming abilities (119). Nevertheless, Grid2 Lc mutants were deficient in spatial orientation toward a hidden platform as well as visuomotor guidance toward a visible one, distance swum and escape latencies being increased (91, 112, 120–122) (Table 3). These results confirm a role for the cerebellum in the visual guidance of movement (123). The same pattern was evident in Reln rl-Orl mutants with ectopias in cerebellum and hippocampus (83) as well as Cacna1α la heterozygotes (92). In contrast, Rora sg (113), Agtpbp1 pcd-1J (124), and nervous (82) mutants were deficient only in the hidden platform subtest, concordant with a role for cerebello-limbic or cerebello-neocortical connections in spatial orientation, further supported by findings of the same dissociation in rats with immunotoxin-induced degeneration of Purkinje cells (125) as well as electrolytic lesions in lateral cerebellar cortex or dentate nucleus (126–128).
Due to their susceptibility to drowning, Dst dt-J mutants cannot be tested in the hidden platform version (129). In the visible platform subtest, escape latencies were higher than controls but path lengths were lower. Unlike the cerebellar mutants described above, the Dst dt-J line is characterized by slower swim speed and intact directional guidance, presumably due to spinocerebellar as opposed to cerebellar atrophy.
6 Regional Brain Metabolism
The effects of cerebellar damage on brain metabolism have been examined by high-resolution cytochrome oxidase (COX) histochemistry. COX is the fourth enzyme of the mitochondrial electron transfer chain of oxidative phosphorylation, immediately prior to ATPase. COX labeling is a specific marker of neuronal activity, since the contribution of glial cells on oxidative metabolism is minimal (130). Because COX histochemistry is expressed as a function of tissue weight, a loss in neuron numbers does not necessarily lead to reduced enzymatic activity. Studies in brain metabolism offer insights into the functional impact of cerebellar dysfunction on remaining cells of this structure as well as cerebellar-related pathways.
In cerebellar cortex of 15- and 25-day-old Grid2 Lc mutants, Purkinje cells could be observed prior to their degeneration. COX activity in these cells was higher than that of wild-type, but unchanged in the molecular layer (131). Hypermetabolism in Purkinje cells probably reflects chronic depolarization of their membrane potential (5). In adult mutants where these cells have disappeared, COX activity of shrunken molecular and granule layers did not differ from that of wild-type, indicating normal metabolic activity relative to tissue weight but higher metabolic activity relative to absolute levels (132). COX activity relative to tissue weight was higher than controls in deep cerebellar and lateral vestibular nuclei (Table 4), attributable to lost gamma-amino butyric acid (GABA)-ergic inhibitory input from Purkinje cells, since excitatory synapses have higher energy demands than inhibitory ones (130). COX activity of Grid2 Lc mutants was also elevated in several regions directly connected with the cerebellum, probably as a result of the hypermetabolic deep nuclei, the only pathway out of the cerebellum. In an opposite fashion, COX activity decreased in inferior olive, precursor to retrograde degeneration as a consequence of Purkinje cell atrophy.
Table 4
Variations of cytochrome oxidase (COX) activity in cerebellar cortex and deep nuclei of cerebellar mutants
Mutants | Molecular layer | Purkinje cell layer | Granule cell layer | Deep nuclei | References |
---|---|---|---|---|---|
Grid2 Lc | Normal | Absent | Normal | ↑ | (132) |
Grid2 ho-Nancy | ↑ | Normal | Normal | Normal | (133) |
Rora sg | Normal | ↑ | Normal | ↑ | (80) |
Reln rl-Orl | Normal | ↑ | Normal | ↓ | (136) |
Regional brain metabolism of Grid2 ho-Nancy mutants differed substantially from Grid2 Lc in view of their milder cerebellar atrophy (133). COX activity in the molecular layer of Grid2 ho-Nancy mutants was higher than that of non-ataxic controls, probably due to increased neural activity of cerebellar afferents in response to defective dendritic organization of Purkinje cells. Unlike Grid2 Lc , COX activity in deep cerebellar nuclei and inferior olive was unchanged in Grid2 ho-Nancy probably because of the preservation of Purkinje cells.
Rora sg mutants share many neuropathological characteristics with Grid2 Lc , in particular massive degeneration in cerebellar cortex. Like Grid2 Lc , COX activity of Rora sg mutants was higher than that of non-ataxic controls in deep cerebellar nuclei and cerebellar target structures such as red and interpeduncular nuclei, but unchanged in granular and molecular cerebellar cortex, though elevated in Purkinje cells (80). Once again, hypermetabolism in deep nuclei is probably due to decreased GABAergic input from depleted Purkinje cells. Unlike Grid2 Lc , COX staining of Rora sg mutants was normal in inferior olive, presumably because of a stabilization in retrograde degeneration. In contrast to the previous two mutants, COX activity decreased in thalamic nuclei, probably as a direct consequence of Rora deletion, since this gene is highly expressed there in normal mouse brain (134, 135).
< div class='tao-gold-member'>
Only gold members can continue reading. Log In or Register a > to continue
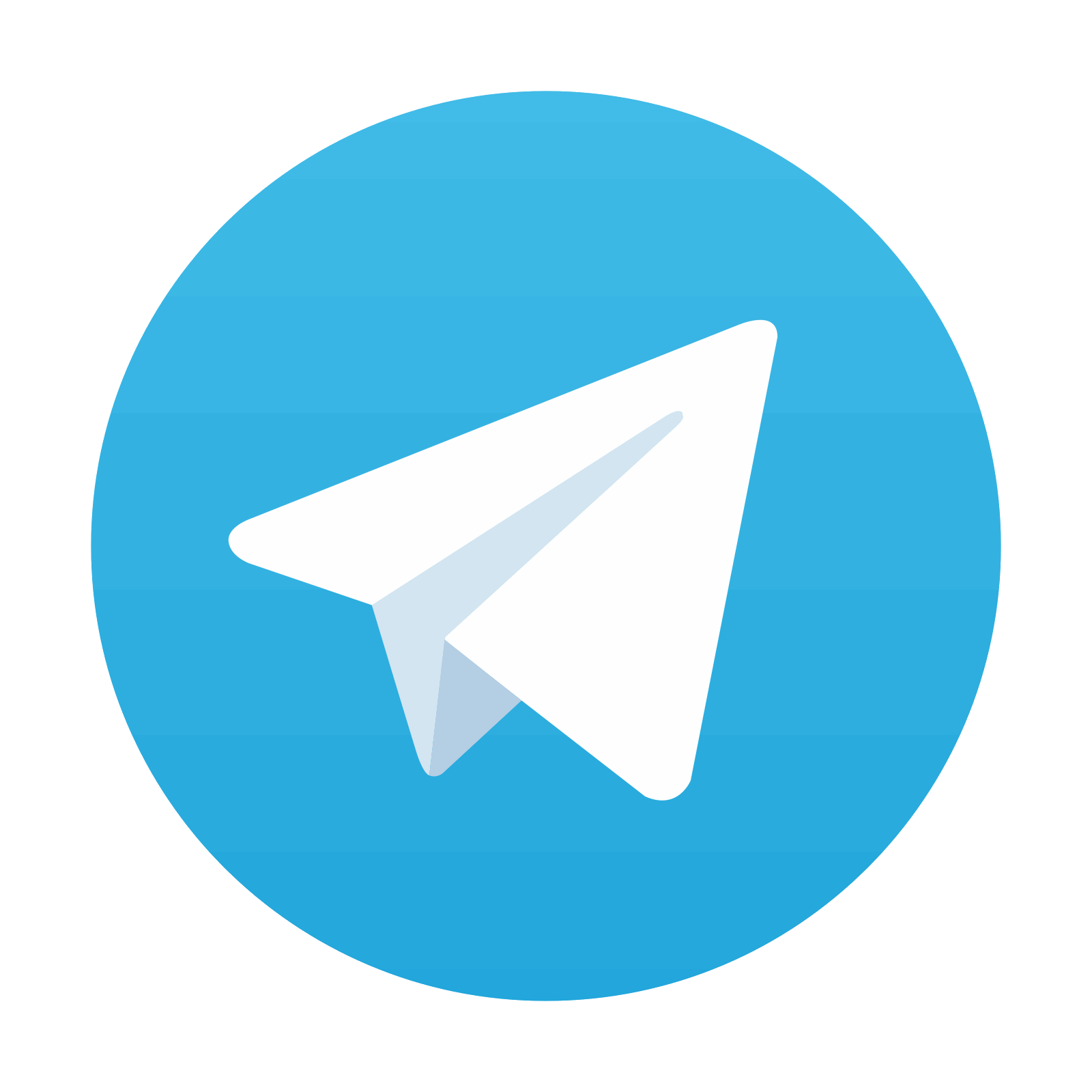
Stay updated, free articles. Join our Telegram channel

Full access? Get Clinical Tree
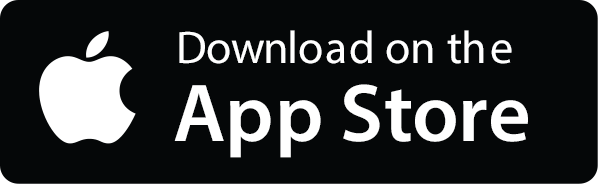
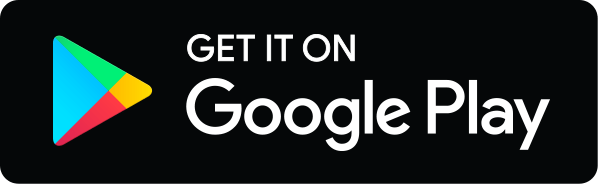