© Springer Science+Business Media New York 2015
Mónica V. Cunha and João Inácio (eds.)Veterinary Infection Biology: Molecular Diagnostics and High-Throughput StrategiesMethods in Molecular BiologyMethods and Protocols124710.1007/978-1-4939-2004-4_3232. Functional Genomics of Tick Vectors Challenged with the Cattle Parasite Babesia bigemina
(1)
Global Health and Tropical Medicine, Instituto de Higiene e Medicina Tropical, Universidade Nova de Lisboa, Lisbon, Portugal
(2)
Instituto de Higiene e Medicina Tropical, Universidade Nova de Lisboa, Lisbon, Portugal
(3)
SaBio, Instituto de Investigación en Recursos Cinegéticos IREC, UCLM-JCCM, Ciudad Real, Spain
(4)
Department of Veterinary Pathobiology, Center for Veterinary Health Sciences, Oklahoma State University, Stillwater, OK, USA
Abstract
Ticks are obligate hematophagous ectoparasites considered as vectors of animal diseases, having a huge economic impact in cattle industry. Babesia spp. are tick-borne pathogens that cause a disease called babesiosis in a wide range of animals and in humans. Control of tick infestations is mainly based on the use of acaricides, which have limited efficacy reducing tick infestations, mostly due to wrong usage, and is often accompanied by the selection of acaricide-resistant ticks, environmental contamination, and contamination of milk and meat products. Vaccines affecting both vector and pathogens constitute new control strategies for tick and tick-borne diseases and are, therefore, a good alternative to chemical control.
In this chapter we describe the identification of Rhipicephalus (Boophilus) annulatus genes differentially expressed in response to infection with B. bigemina by using suppression-subtractive hybridization (SSH), which allows the identification of differentially expressed genes. The results of the SSH studies are validated by real-time reverse transcription (RT)-PCR. Functional analyses are conducted by RNAi on selected R. annulatus genes to determine their putative role in B. bigemina–tick interactions. Gathered data may be useful for the future development of improved vaccines and vaccination strategies to control babesiosis.
Key words
TickGenomics Babesia Rhipicephalus Boophilus Subtractive hybridizationRNA interferenceVaccines1 Introduction
Tick-borne pathogens of the genus Babesia are Apicomplexan parasites responsible for a disease called babesiosis that affects a wide range of animals and, occasionally, humans. The major economic impact of babesiosis is on cattle industry, caused mainly by Babesia bovis and B. bigemina. Rhipicephalus (Boophilus) spp. ticks are their principal vectors, being considered one of the most important cattle ectoparasites due to the direct impact affecting leather quality, meat and milk production and, most importantly, pathogen transmission capacity [1].
In the last decades, new tools have been developed in the molecular biology field, leading to a better knowledge of genes involved in the control of biological functions in numerous organisms, such as vector ticks and pathogens transmitted to man and animals. Genomics continues to contribute greatly to this cause by (a) bringing new information about genomes and development of new technologies, (b) allying these new resources to traditional techniques, and (c) enabling the development of new practical interventions in the triangle vector–host–pathogen. Few complete genomes are known for either vector or pathogen but information regarding partial and complete nucleotide sequences from both organisms is being progressively more available. Moreover, sialomes and transcriptomes of different ticks are now accessible. New findings on the genomic field do not replace older strategies developed to study tick and tick-borne pathogens but rather complement them, improving research outputs. As examples, there are techniques such as artificial feeding or cellular lines that remain extremely useful to determine biological effects in cells and organisms.
Within new control strategies for tick and tick-borne diseases, vaccines arise as important alternatives to chemical control since they may affect both vector and pathogen. Molecular interactions at the tick–pathogen interface ensure survival and development of both the pathogen and tick vector. Recent studies demonstrated that tick vaccines reduce tick–pathogen infection when using antigens found to be related with pathogen infection/multiplication, illustrating the complexity of tick–pathogen coevolution [2]. Other experiments have demonstrated that tick gene expression is modified in response to pathogen infection [3–12], but information on the function of differentially expressed genes is limited [7–11, 13–15] mainly because the identification of potential vaccine candidates is not simple, being a crucial step.
In the present example, it is our aim to demonstrate how gene functional analysis can support the identification of genes that are involved in the vector–pathogen interaction using as model the vector R. annulatus and the pathogen B. bigemina.
To infer about the pressure of infection in the vector, first, two different tick populations had to be produced: a B. bigemina-infected population and an uninfected one. Factors like gender, time point in the life cycle, and similar feeding conditions have to be considered and controlled. The only feature that should be different in these samples is the presence of infection that eventually will induce a differential expression of the genes reflecting the effect of the pathogen in the tick organism. Secondly, the differences between these populations have to be identified and measured. The subtractive suppression hybridization (SSH) method allows the detection of transcripts differently expressed in two related mRNA populations with no prior knowledge of these mRNA. This technique relies on the hybridization of equal mRNA present on two different populations, followed by the enrichment of different mRNA (present in only one of the populations) by traditional PCR. When using SSH, the differentially expressed genes need to be quantitatively validated in order to point which are the most relevant transcripts in the picture. Microarrays can appear as a valid option, but due to the costs linked to this technique, the gain must be well weighted. For example, if the objective of the study is to analyze gene expression profiles in different situations, a microarray chip can be a solution, but if the target is the punctual identification of relevant genes, then the real-time PCR technique is a more suitable and simpler way to quantify mRNA.
Another conceptually similar technique to SSH is the direct sequencing of mRNA also known as RNA-Seq. This high-throughput technique offers a complete image of what is happening in the transcriptome in a determined time point, so when comparing an mRNA population corresponding to infected and healthy populations, the transcriptome differences will arise. An extremely important task in the identification of transcripts is the sequence analysis steps. Various tools are accessible online in which a comparison to available datasets is done. The cDNA Annotation System software (dCAS) [16] is a good option for performing an automated sequence clean-up, assembly, and BLAST analysis against the nonredundant (nr) sequence database. Manual curation is imperative after the automated analysis to complete this step. From this step on, it is possible to identify differentially expressed genes that can be further characterized.
The third, and last step, on finding new antigens involved in infection processes, within the example of B. bigemina-infected R. annulatus ticks, concerns to the functional analysis. These assays allow demonstrating the active role of antigens in a specific process. RNAi or posttranscriptional gene silencing is a conserved and natural process that cells use to turn down, or silence, specific genes [17, 18]. Small interfering RNAs (siRNAs) are the effector molecules of the RNAi pathway that is initiated by double-stranded RNA (dsRNA) and results in a potent sequence-specific degradation of cytoplasmic mRNAs containing the same sequence as the dsRNA trigger [8, 19]. RNAi revealed to be a valuable tool for studying tick gene function, the characterization of the tick–pathogen interface and the screening and characterization of tick-protective antigens [20]. RNAi process in ticks has not yet been revealed but a model has been proposed based in the information available for Drosophila melanogaster and Anopheles gambiae [21]. This technique has been used to study the function of tick proteins at the tick–pathogen interface in a number of tick species [12, 22–25]. In ticks, depending on the experimental design and targets, four different techniques of dsRNA delivery are known: (a) injection, the most common method; (b) soaking of isolated tissues; (c) the RNAi-inducing capillary feeding; and (d) virus production of dsRNA. Herein, the selected method was the injection of dsRNA directly in the hemolymph in adult female ticks since it was vital that ticks would feed normally after gene silencing. The evaluation of gene knockdown in biological parameters like weight and reproduction success, or even, as in the present case, the infection level, elucidates on the effect gene disruption.
2 Materials
2.1 Organisms
1.
Rhipicephalus annulatus ticks.
2.
Babesia bigemina (Moledet strain, provided by Kimron Veterinary Institute, Israel).
3.
Holstein–Friesian calves.
2.2 General Laboratory Consumables, Materials, and Equipment
1.
Sterile 1 ml syringes and needles with different gauges.
2.
Sterile 1.5 ml and 2 ml microcentrifuge tubes.
3.
96 wells plates for PCR.
4.
Micropipettes and filter tips.
5.
Agar plates (LB Agar, 20 g/L, with appropriate antibiotics and supplements).
6.
1× TAE (Tris-acetate buffer): 40 mM Tris–HCl, 20 mM acetic acid, and 1 mM EDTA.
7.
PBS (phosphate buffered saline): 0.027 M potassium chloride, 0.137 M sodium chloride, and 1.76 mM potassium phosphate.
8.
0.5× TBE (Tris-borate-EDTA buffer): 45 mM Tris, 45 mM boric acid, 1 mM EDTA, pH 8.3.
9.
Agarose.
10.
Nuclease-free water.
11.
Refrigerated centrifuge.
12.
Thermocycler (e.g., GeneAmp® PCR System 2700, Applied Biosystems Life Technologies, Foster City, CA, USA).
13.
Real-time thermal detection system (e.g., IQ5 thermo-cycler, BioRad, Hercules, CA, USA).
14.
NanoDrop ND-1000 (Thermo Fischer Scientific, Waltham, MA, USA).
15.
Stereo microscope.
16.
Humidity chamber.
2.3 Suppression Subtractive Hybridization (SSH) Library Construction
DNA and RNA Extraction
1.
RNAlater™ solution (Ambion Life Technologies, Carlsbad, CA, USA).
2.
TRI Reagent® (Sigma-Aldrich, St. Louis, MO, USA). Additional reagents needed: chloroform, isopropanol, absolute ethanol, 75 % ethanol, 1 mM sodium citrate in 10 % ethanol, and 8 mM NaOH.
Detection of Babesia bigemina Infection in Ticks
1.
R. annulatus DNA.
2.
PCR Master Mix (Promega, Fitchburg, WI, USA).
3.
Specific primers for B. bigemina (e.g., 5′-AGC TTG CTT TCA CAA CTC GCC-3′ and 5′-TTG GTG CTT TGA CCG ACG ACA T-3).
SSH Library Construction
1.
Infected and uninfected tick total RNA.
2.
FastTrack® 2.0 mRNA Isolation Kit (Invitrogen Life Technologies, Carlsbad, CA, USA).
3.
PCR-Select™ cDNA Subtraction Kit (Clontech Laboratories Inc., Mountain View, CA, USA).
4.
Advantage® 2 PCR polymerase mix with TITANIUM Taq DNA Polymerase (Clontech-Takara, Mountain View, CA, USA).
cDNA Library Cloning
1.
TOPO TA Cloning® Kit for sequencing (Invitrogen Life Technologies, Carlsbad, CA, USA).
2.
One Shot® TOP10 competent cells (Invitrogen Life Technologies, Carlsbad, CA, USA).
3.
PCR Master Mix (Promega, Fitchburg, WI, USA).
4.
Universal T3 and T7 primers.
5.
Illustra plasmidPrep Mini Spin Kit (GE Healthcare, Buckinghamshire, UK).
2.4 Sequence Analysis and Database Search
1.
Sequences from SSH clones.
2.
cDNA Annotation System software (dCAS; Bioinformatics and Scientific IT Program (BSIP), Office of Technology Information Systems (OTIS), National Institute of Allergy and Infectious Diseases (NIAID), Bethesda, MD, USA) (http://exon.niaid.nih.gov).
3.
AlignX (included in the Vector NTI Suite V 5.5 software, Invitrogen Life technologies, North Bethesda, MD, USA).
2.5 Validation of SSH Results
1.
Infected and uninfected R. annulatus RNA.
2.
iScript™ cDNA Synthesis Kit (BioRad, Hercules, CA, USA).
3.
iQ SYBR Green Supermix (BioRad, Hercules, CA, USA).
4.
Specific and housekeeping primers.
2.6 Functional Tick Gene Analysis by RNA Interference
dsRNA Synthesis
1.
R. annulatus RNA.
2.
Specific primers for target genes with T7 extension.
3.
Access RT-PCR system (Promega, Madison, USA).
4.
PCR products purification kit (e.g., PureLink™ PCR Purification Kit, Life Technologies, Carlsbad, CA, USA).
5.
MEGAscript® T7 Kit (Ambion Life Technologies, Carlsbad, CA, USA).
Injection in Ticks
1.
Specific dsRNA.
2.
Hamilton syringe, with 1 in., 33 G needle.
3.
Forceps/tweezers.
4.
Double face duck tape.
5.
Dental wax plates.
6.
Control buffer: 10 mM Tris–HCl, pH 7, 1 mM EDTA.
Tick Feeding
1.
Infected B. bigemina calf.
2.
Freshly molted female R. annulatus.
3.
Contact glue.
4.
Cotton sleeves.
5.
Razor blade.
Gene Knockdown Assessment and Infection Assessment
1.
R. annulatus ticks treated with dsRNA.
2.
iScript™ One-Step RT-PCR Kit with SYBR® Green (Biorad, Hercules, CA, USA).
3.
Gene-specific and housekeeping primers.
3 Methods
3.1 Ticks Processing
3.1.1 Uninfected and Babesia bigemina-Infected Ticks for SSH Library Construction
Obtain B. bigemina-infected and uninfected R. annulatus female ticks from experimentally infected and babesiosis-free 3 to 4-month-old male Holstein–Friesian calves, respectively (see Note 1 ).
1.
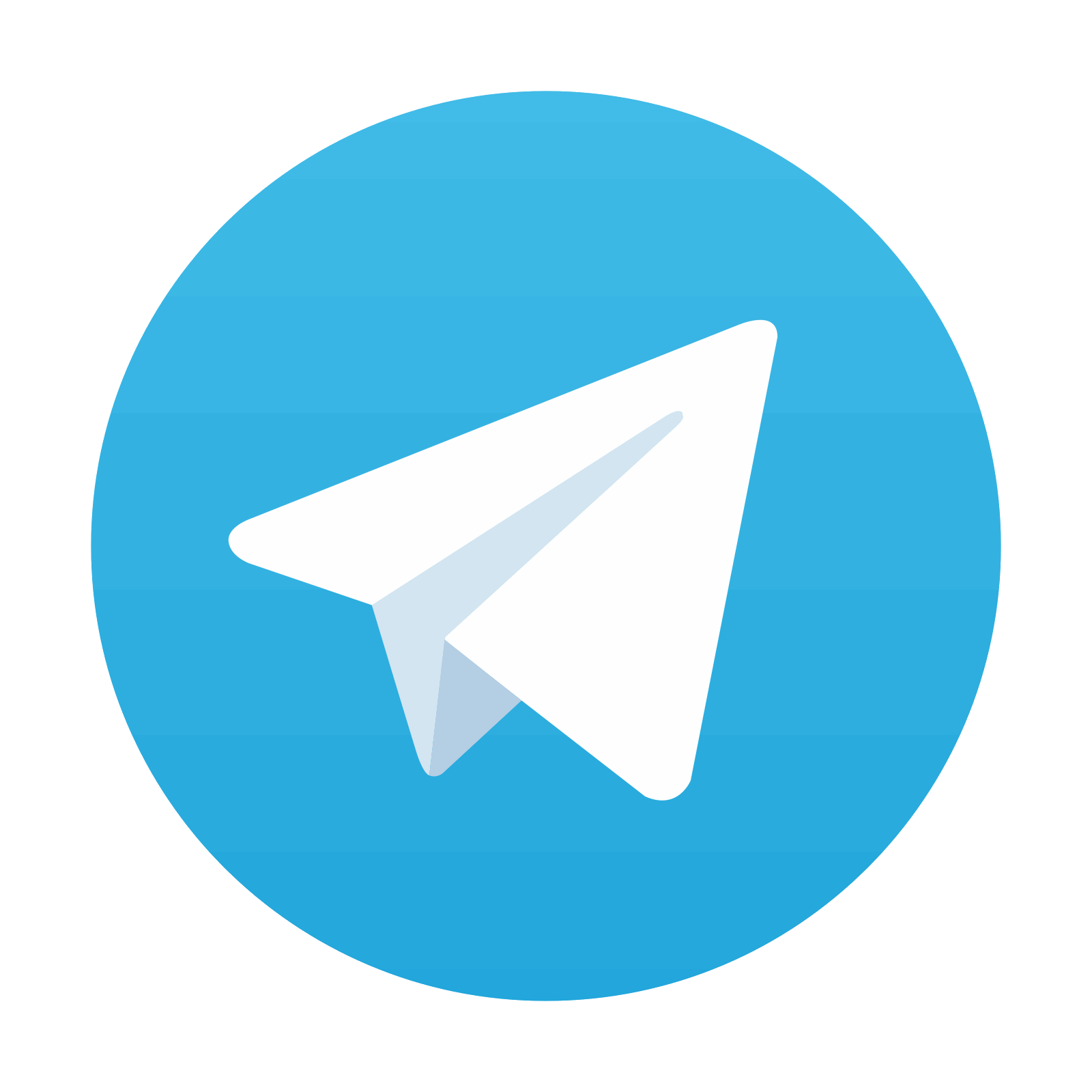
Prior to tick infestation, test calves for antibodies against Babesia spp. infection, maintained under strict tick-free conditions, using an indirect fluorescent antibody (IFA) assay [26].
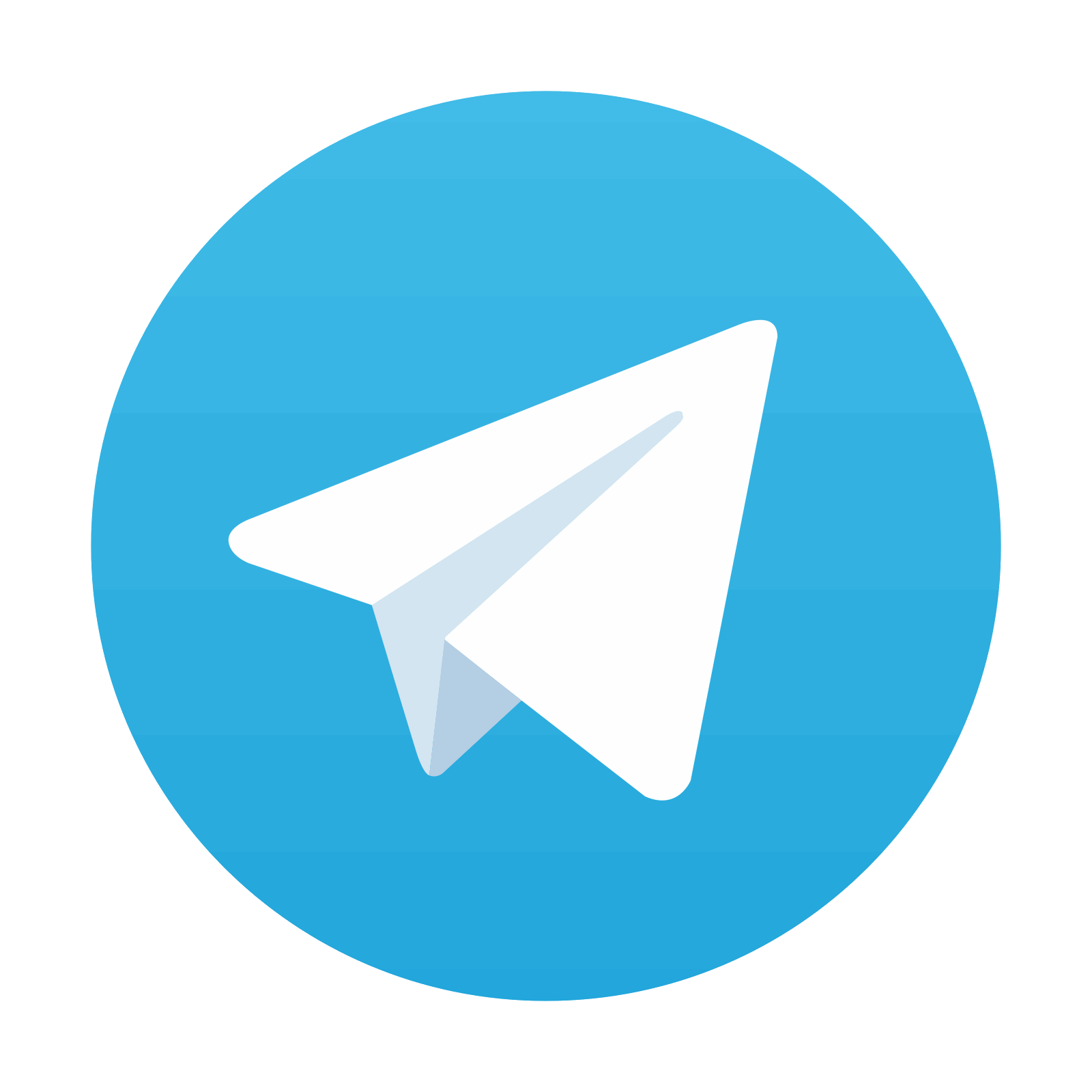
Stay updated, free articles. Join our Telegram channel

Full access? Get Clinical Tree
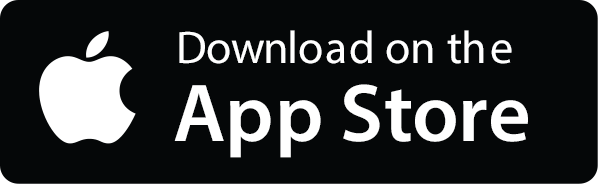
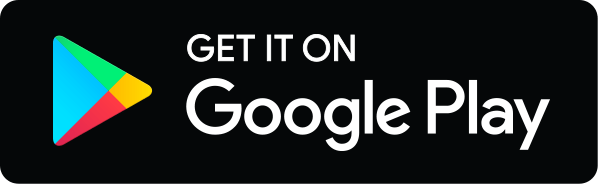
