Fig. 1.
Illustrative LHSS rate-frequency curve indicating the defining parameters: asymptotic or maximum reinforcement rate (reinforcements per minute), and the M-50 and theta-0 reward thresholds in pulses per second (pps).
2 Materials and Procedures
2.1 Subjects
Adult male Sprague-Dawley rats are housed in a central animal facility in individual plastic cages with free access to water and laboratory chow, except when food restriction regimens are in force. The animal room is maintained on a 12:12 h light:dark cycle, with lights turned on at 0700 hours. Animals are transported to the laboratory where all behavioral testing is conducted during the light phase. All experimental procedures involving use of laboratory animals are approved by the Institutional Animal Care and Use Committee at the New York University School of Medicine and are consistent with the Principles of Laboratory Animal Care (NIH Publication no. 85-23).
2.2 Food Restriction
All rats initially have ad libitum access to pelleted rat chow with a physiological fuel value of 3.36 kcal/g, consisting of 28.5% protein, 13.5% fat, and 58% carbohydrates (LabDiet #5001). Ad libitum fed rats consume 25–35 g of this diet per 24 h period. Half of the rats in each experiment are placed on a restricted feeding regimen in which daily intake is limited to 10–12 g of the laboratory diet. The daily meal is generally delivered at 1700 hours and meal size is held constant until a 20% loss of body weight is achieved; this takes approximately 2 weeks. Daily food allotment is then adjusted to stabilize body weight at this value for the remainder of the study. Generally, food-restricted rats consume their daily ration within 1 h of its delivery which results in approximately 23 h of daily food deprivation.
2.3 Surgical Procedures
All stereotaxic surgery is performed with animals under deep anesthesia using a mixture of ketamine (100 mg/kg) and xylazine (10 mg/kg) injected intraperitoneally (i.p.). Atropine (0.4 mg/kg, i.p.) is also administered to prevent bradycardia associated with anesthesia. Before each surgery, forceps, hemostats, and other surgical instruments are washed and then sterilized in a dry glass bead sterilizer (Cole-Parmer, Vernon Hills, IL).
Once an animal is anesthetized, as confirmed by absence of corneal and pedal reflexes, the scalp is shaved and the animal is stabilized in an ear bar adaptor and positioned into the stereotaxic frame (#1404; Kopf Instruments, Tujunga CA). Initially, the incisor bar is set at −3.5 mm to approximate the skull flat plane, later to be confirmed. Corneas are coated with ophthalmic ointment, and the scalp is prepared with three applications of Betadine and 70% isopropyl alcohol in alternation. A single longitudinal scalp incision is made exposing the skull suture landmarks, bregma, and lambda. Once the head position is adjusted to ensure that bregma and lambda lie in the same horizontal plane, stereotaxically guided holes for electrode(s) and/or cannula(s) are placed using a high speed stereotaxic drill. A hand drill is used to prepare holes for three stainless steel anchoring screws and a ground screw. Following placement of electrode(s) and/or cannula(s) plus screws, all are secured to the skull by application of dental acrylic. Upon completion of surgery, antibiotic ointment is applied topically to the margins of the scalp incision. Following removal from the stereotaxic frame, rats are placed in a clean cage with a warming pad beneath. Postsurgical analgesia is achieved by administration of the nonsteroidal anti-inflammatory drug Banamine (2 mg/kg, s.c.).
2.3.1 Electrode Implantation
Commercially available (Plastics One, Roanoke, VA, USA) untwisted bipolar electrodes, 0.25 mm diameter and 12 mm length (part# MS303/1-A), are modified by removing 0.3 mm of insulation from the tip of one wire (the electrode) and all insulation from the other wire, which is bent at a 90° angle. One end of a 3.0 cm length of copper wire is soldered to the bent pole while the other is soldered to a stainless steel screw that will be placed in the anterior skull and serve as ground.
Rats are stereotaxically implanted with the monopolar stimulating electrode in the lateral hypothalamic medial forebrain bundle at coordinates: 3.0 mm posterior to bregma, 1.6 mm lateral to the sagittal suture, and 8.6 mm ventral to skull surface. Once dental acrylic is applied around the electrode cures, the ground screw is placed and additional acrylic is applied. The electrode socket is covered with a dust cap (Plastics One, part# 303DC/1) when not in use.
2.3.2 Cannula Implantation
In studies requiring acute intraventricular microinjections, rats are implanted with a single 26-gauge stainless steel guide cannula (Plastics One, part# C315G) placed 1.0 mm dorsal to the lateral ventricle contralateral to the stimulating electrode (coordinates: 1.0 mm posterior to bregma, 1.5 mm lateral, and 3.4 mm ventral to skull surface). During surgery a stainless steel wire is inserted into the guide to maintain patency. After surgery, patency is maintained using a commercially available stylet (Plastics One, part# C315DC).
For microinjections in the NAc shell, rats are implanted with two chronically indwelling 26-gauge stainless steel guide cannulas (Plastics One, part# C315G) placed bilaterally 2.0 mm dorsal to intended injection sites (1.6 mm anterior to bregma; 2.1 mm lateral with tips angled 8° toward the midline, 5.8 mm ventral). Guide cannula patency is maintained using stylets.
For continuous infusion of drug into the ventricular system, a 22-gauge guide cannula with a horizontal access port (Plastics One, part# C322OP) is implanted in the lateral ventricle (coordinates: 1.0 mm posterior, 1.5 mm lateral, and 4.5 mm ventral). The guide cannula is positioned so that the horizontal port faces rear to enable connection to an osmotic minipump. The horizontal port is covered with a short length of polyvinyl tubing melted at the tip as a temporary seal.
2.3.3 Minipump Installation and Removal
Following recovery from cannula/electrode surgery and initial behavioral training, a second surgery may be performed to install a subcutaneous “14-day” osmotic minipump (Alzet model 2002; Durect, Cupertino CA) and connect it to the horizontal port of the intraventricular cannula.
Minipumps and fill solutions are prepared the day before surgery using sterile procedures. In general it is necessary to prepare 0.3–0.4 mL of solution to fill each minipump. The filling of minipumps consists of (1) attaching a 4 cm length of gas-sterilized polyvinyl tubing to the minipump flow moderator; (2) loading a syringe with the prepared solution, connecting it to a Millex filter unit (Millipore, Billerica, MA), and attaching a sterile needle (23 g); (3) filling the tubing and flow moderator, removing the needle from the filter and attaching the filter to a filling tube; (4) inserting the filling tube into the minipump and delivering the solution slowly until overflow (at least 0.3 mL); (5) inserting the previously filled flow moderator into the filled minipump. Once a minipump is filled, it is placed in a sterile container in sterile 0.9% saline to incubate overnight at 36°C.
For surgery, rats are briefly anesthetized by 5-min exposure to isoflurane vapor and oxygen delivered at 1 L/min in an enclosed chamber, using a mobile laboratory animal anesthesia system (VetEquip Inc., Pleasanton, CA). Once rats are anesthetized, they are removed from the enclosed chamber but continue to receive the isoflurane mix through a tube connected to a nose cone. Rats are placed on a flat surface covered with a sterile drape. The temporary polyvinyl cap is removed from the intraventricular cannula port and the cannula is flushed with 10 μL sterile 0.9% saline. After shaving and disinfecting the surgical site, a longitudinal incision is made slightly posterior to the scapulae. A sterilized hemostat is inserted into the incision to create a subcutaneous pocket. The primed minipump is inserted into the subcutaneous channel and the attached polyvinyl tubing is connected to the intraventricular cannula port. The incision is closed with suture or surgical wound clips, the horizontal port and attached tubing are covered with dental acrylic, and the area around the incision is disinfected and swabbed with an antibiotic ointment.
Following the 2-week infusion period, rats are again briefly anesthetized with isoflurane. The minipumps are disconnected and removed by cutting the polyvinyl tubing, extracting the minipump and flushing the subcutaneous channel with sterile 0.9% saline. The portion of the tubing attached to the cannula is sealed by melting and is then covered with dental acrylic. The incision is closed with a wound clip and the area is swabbed with Betadine followed by antibiotic ointment.
2.3.4 Histological Verification of Cannula and Electrode Placements
Upon completion of behavioral testing, rats are euthanized with CO2 and decapitated. Brains are removed and placed in 10% buffered formalin for at least 48 h. Using a cryostat (Reichert Jung, Frigocut 2800), 40 μm frozen coronal sections are cut, thaw mounted on gelatin-coated glass slides, and stained with cresyl violet. Cannula and electrode placements are determined by visual inspection of sections under an Olympus (#343750) dissecting microscope.
2.4 Acute Microinjection Procedures
For microinjections, solutions are loaded into 30 cm lengths of polyethylene tubing (PE-50; Intramedic Clay Adams No 427411, Becton Dickinson, Sparks, MD) attached at one end to a glass microsyringe (Hamilton, Bonaduz, Switzerland) filled with distilled water, and at the other end to a 33-gauge injector needle, which extends 1.0 mm (ventricular) or 2.0 mm (NAc) beyond the implanted guide. For ventricular injections, a 250-mL syringe is mounted on a Harvard 2272 microliter syringe pump delivering the 5.0 μL injection volume over a period of 95 s. For intra-NAc injections, two 25-μL Hamilton are mounted on the twin holders of the syringe pump for simultaneous delivery of the 0.5 μL injection volumes over a period of 100 s. Alternatively, a smaller volume syringe may be used to slowly deliver the solution manually, through one (or both cannulas simultaneously) over a period of 2 min. One minute following the injections, the injector needle is removed, the stylet replaced, and the animal is placed in either a waiting cage or the test chamber depending on the interval to the postinjection test.
Accuracy of ventricular cannula placements is verified prior to drug testing by demonstrating an immediate (i.e., <60 s latency) and sustained (i.e., >30 s) drinking response to a 5 μL injection of 50 ng of angiotensin II.
2.5 LHSS Procedures
2.5.1 Apparatus
Brain stimulation training and testing are conducted in eight standard operant test chambers (26 × 26 × 21 cm; Med-Associates, Georgia, VT) placed within sound attenuating cubicles (Med-Associates, ENV 018 MD). Each chamber has a retractable lever mounted on one wall and a house light mounted on the opposite wall. Eight constant current stimulators (Med-Associates, PHM 152B/2) are used to deliver trains of 0.1-ms cathodal pulses, which are conducted to implanted electrodes by way of commutators and flexible cables. The eight chambers are separated into two groups of four and each group is connected to a Dell computer and an interface (Med-Associates, DIG-700P2) that controls electrical stimulation, contingencies, and data recording. All stimulation parameters are monitored on two Tektronix (TAS 455) oscilloscopes.
2.5.2 LHSS Rate-Frequency Training
After 1 week of postsurgical recovery, rats are exposed to the operant chamber and trained to lever press for 0.5 s trains of electrical stimulation consisting of cathodal square wave pulses of 0.1-ms duration at a frequency of 100 pulses per second (pps). The stimulation is delivered to the implanted electrode via cable and commutator (Plastics One, part# SL2C), allowing the animal to move freely around the chamber. The initial stimulation intensity of 120 μA is systematically varied to locate, for each rat, the lowest intensity that will maintain a high rate of lever pressing without signs of motor impairment or aversive side effects. Once lever pressing is acquired, rate-frequency training starts with sessions consisting of 36 or 24 sixty-second trials. Extension of the response lever and a 2-s train of “priming” stimulation initiates each trial. Each trial is terminated by retraction of the lever and is followed by a 10-s intertrial interval. Each lever press produces a 1-s train of stimulation, except for those presses emitted during the stimulation train, which do not increase reinforcement density. The number of lever presses and stimulations delivered (reinforcements) is recorded for each trial. Rate-frequency curves are generated by presenting twelve trials in which the brain stimulation frequency decreases over successive trials (approximately 0.05 log units each trial) from an initial frequency of 100 pps to a terminal frequency of 28 pps. At least three such series are presented in each training session. The first series in any given session is always considered to be a “warm-up” and data are discarded. Training continues for approximately 2 weeks until rate-frequency curves stabilize. During the final few training sessions rats are habituated to the injection procedures to be used in future test sessions.
2.5.3 LHSS Test Sessions
Test sessions begin with a preinjection test. Rats are then removed from the chamber for acute intracerebral or systemic drug injection. Injection is followed by an interval that varies depending upon the drug injected and route of administration and is followed by the postinjection test. For each rate-frequency series, the number of reinforcements obtained at each brain stimulation frequency is recorded. Two series from each test are averaged to yield a single rate-frequency function per test, per rat.
2.5.4 Data Analysis
For each test, the rate-frequency function is used to derive four independent parameters. The asymptotic reinforcement rate or maximum rate (described by a line that parallels the x-axis) is defined as the mean of all consecutive values within 10% of the highest rate for the curve. All remaining values comprise the descending portion of the curve, with the lowest point being at the highest frequency to produce fewer than 2.5 reinforcements per minute. Regression analysis of the descending portion of the curve is used to calculate three additional parameters: the stimulation frequency sustaining half the maximum reinforcement rate (M-50 threshold), the minimum stimulation frequency that maintains responding (theta-0 threshold), and the slope of the regression line. Antilog transformations are then applied and natural frequencies are used in subsequent calculations. Acute drug effects are expressed as the percentage change in a parameter in the postinjection test relative to the preinjection test. Treatment effects on each parameter are analyzed separately by two-way mixed design ANOVA with drug treatment as the within subjects factor and diet as the between subjects factor. If a study includes osmotic minipump-driven intraventricular infusions, results are analyzed by three-way mixed design ANOVA with osmotic minipump treatment as an additional between subjects factor.
3 Notes
3.1 Examples of Studies and Results Obtained
3.1.1 Acute Intracerebral Microinjections
Lateral ventricular injection of d-amphetamine or the D-1 DA receptor agonist, SKF-82958, activated neurons in NAc as indicated by immunostaining for the protein product of the immediate-early gene c-fos. Both drugs produced stronger activation in food-restricted than in ad libitum fed rats (96, 97). Corresponding increases in drug rewarding effects were predicted. These predictions were confirmed by demonstrations that microinjection of d-amphetamine and SKF-82958 into the lateral ventricle or NAc shell lowered the LHSS threshold and did so to a greater extent in food-restricted than ad libitum fed subjects (14, 89). Figure 2 displays actual rate-frequency curves for single ad libitum fed and food-restricted rats injected with d-amphetamine, and regression lines obtained from group parameters.
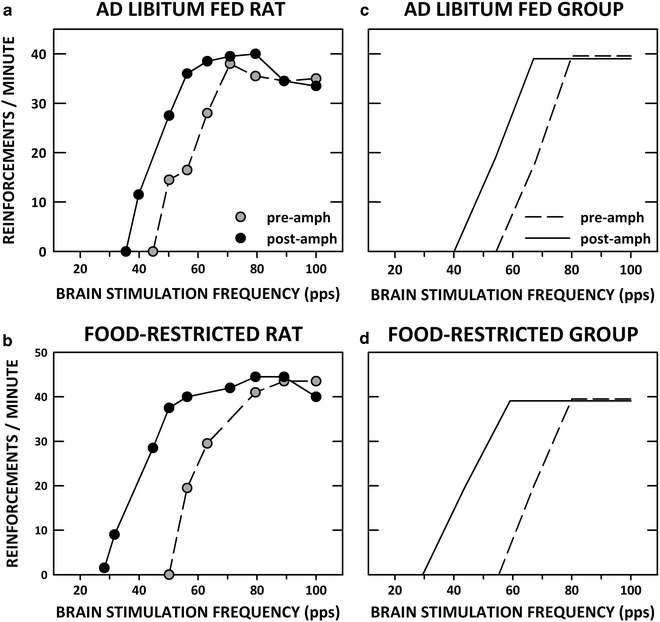
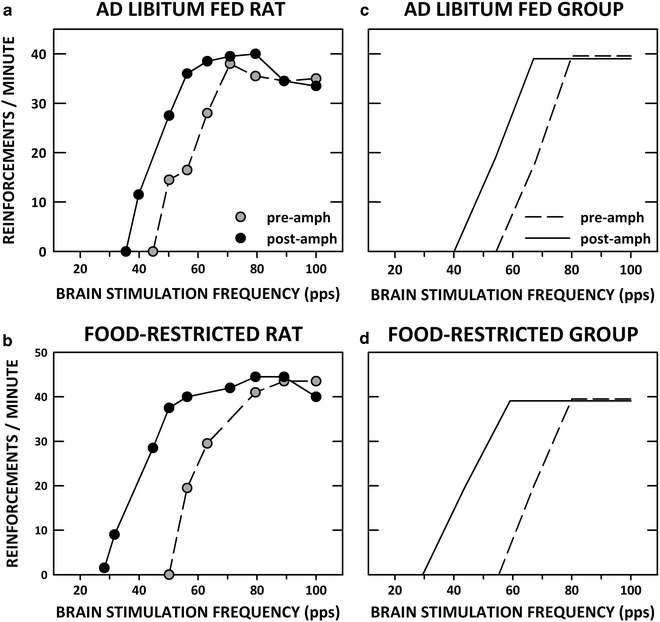
Fig. 2.
LHSS rate-frequency curves before and after lateral ventricular microinjection of d-amphetamine (100 μg/5.0 μl). (a) and (b) display actual rate-frequency curves from one ad libitum fed (a) and one food-restricted rat (b) before (pre-amph) and after (post-amph) microinjection. (c) and (d) display rate-frequency regression lines obtained from the group parameters.
3.2 Investigations of Reward Modulation Based on a Biochemical Finding
3.2.1 Negative Case: ERK 1/2 Phosphorylation
Lateral ventricular injection of SKF-82958 produced a markedly greater increase in activation of ERK 1/2 /MAP kinase in NAc of food-restricted relative to ad libitum fed rats (98). Yet, inhibitors of the kinase upstream of ERK (i.e., MEK inhibitors: SL-327, U0126) injected systemically or directly into NAc shell did not alter the rewarding effect of d-amphetamine in food-restricted rats, despite the fact that MEK inhibition blocked activation of the downstream nuclear transcription factor, CREB, and the immediate-early gene, c-fos (99, 100). While this result will be instructive in pursuing altered D-1 DA receptor-mediated synaptic plasticity in food-restricted rats (101, 102), it did not illuminate the enhanced unconditioned rewarding effect of a DA-releasing psychostimulant drug.
3.2.2 Positive Case: GluA1 Phosphorylation
SKF-82958 and cocaine were found to increase phosphorylation of the AMPA receptor GluA1 subunit on Serine-845 with a greater effect in food-restricted than ad libitum fed rats (103, 104). GluA1 phosphorylation on Ser845 increases channel open probability and mediates activity-dependent trafficking of cytoplasmic GluA1 to the extrasynaptic membrane as a first step in a two-step process that leads to synaptic insertion (105–111). Phosphorylation and trafficking of GluA1 are involved in synaptic strengthening and have been shown to produce both transient and enduring changes in neuronal excitability and behavior (112, 113). The relevance of this finding to ingestion of palatable food by food-restricted subjects was supported by the finding that brief intake of a 10% sucrose solution increased phosphorylation of GluA1 on Ser845 in food-restricted but not ad libitum fed rats (103) and increased AMPA receptor trafficking to the NAc postsynaptic density (81). To test whether D-1 DA receptor stimulation increases GluA1 involvement in acute rewarding effects, SKF-82958 microinjection in NAc was combined with 1-NA-spermine, a polyamine antagonist of Ca++-permeable AMPA receptors (presumed to be GluA1 homomers). 1-NA-spermine selectively decreased the rewarding effect of SKF-82958 in food-restricted rats (103). This finding suggests that increased GluA1 function downstream of D-1 DA receptor stimulation accounts, at least partly, for the increased rewarding effect of NAc D-1 receptor stimulation in food-restricted rats. Figure 3 displays rate-frequency regression lines derived from group parameters of ad libitum fed and food-restricted subjects tested in this study.
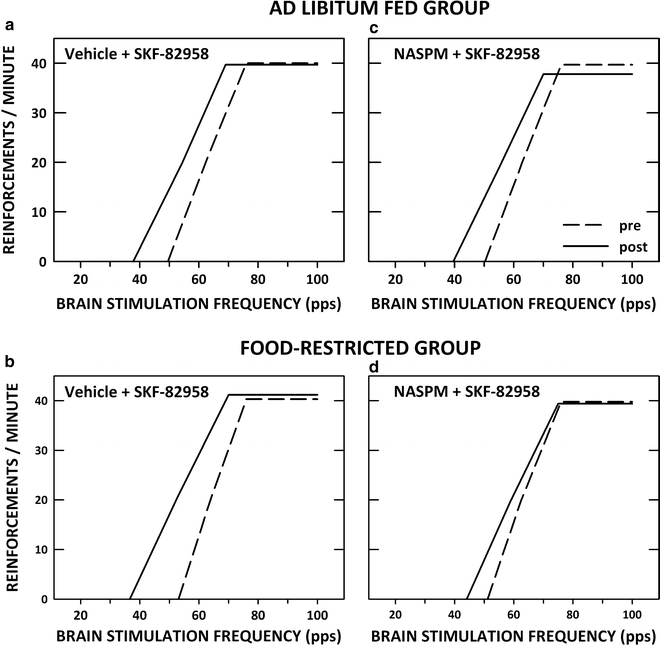
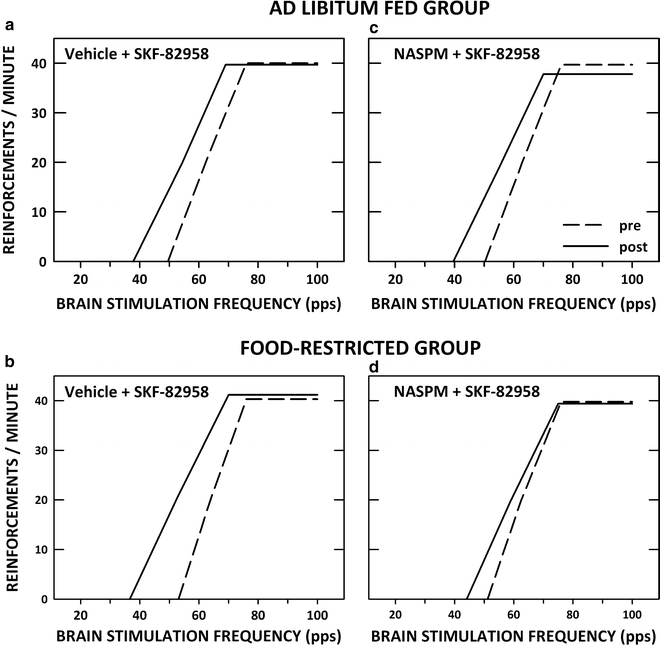
Fig. 3.
LHSS rate-frequency regression lines derived from group parameters in ad libitum fed (a) and (c) and food-restricted (b) and (d) rats before (pre) and after (post) bilateral intra-NAc shell microinjection of the D-1 dopamine receptor agonist SKF-82958 (3.0 μg/0.5 μL/side) alone (a and b) or combined with 1-naphthyl acetyl spermine (25.0 μg; c and d).
3.3 Assessment of Hypoleptinemia Involvement in the Modulation of Reward by Food Restriction
Leptin receptors are expressed by VTA DA neurons and modulate DA neuronal function (114–116). To assess whether hypoleptinemia underlies the enhancing effect of food restriction on drug reward, we continuously infused mouse recombinant leptin (0.5 μg/0.5 μL/h) via lateral ventricular cannulas for 12 days in ad libitum fed and food-restricted rats (117). Leptin infusion in ad libitum fed rats decreased food intake and body weight to levels comparable to those of vehicle-infused animals that were subjected to experimenter-imposed food restriction. Moreover, the leptin-infused subjects displayed enhanced rewarding effects of d-amphetamine, which reversed in tandem with body weight recovery during the 2 weeks following removal of the leptin-containing osmotic minipump. Thus, subjects that decreased intake and lost body weight as a result of chronic leptin infusion displayed a pattern of altered responsiveness to d-amphetamine similar to subjects undergoing and recovering from experimenter-imposed food restriction (118). Leptin infusions had no effect on intake, body weight, or the rewarding effect of d-amphetamine in food-restricted rats. The latter result suggests that hypoleptinemia is not involved in the enhancing effect of food restriction on drug reward and the former result suggests that decreased food intake and body weight can enhance drug reward in the absence of hunger and deprivation. Figure 4 displays rate-frequency regression lines derived from group parameters of ad libitum fed rats challenged with d-amphetamine before and during operation of vehicle- and leptin-filled osmotic minipumps.
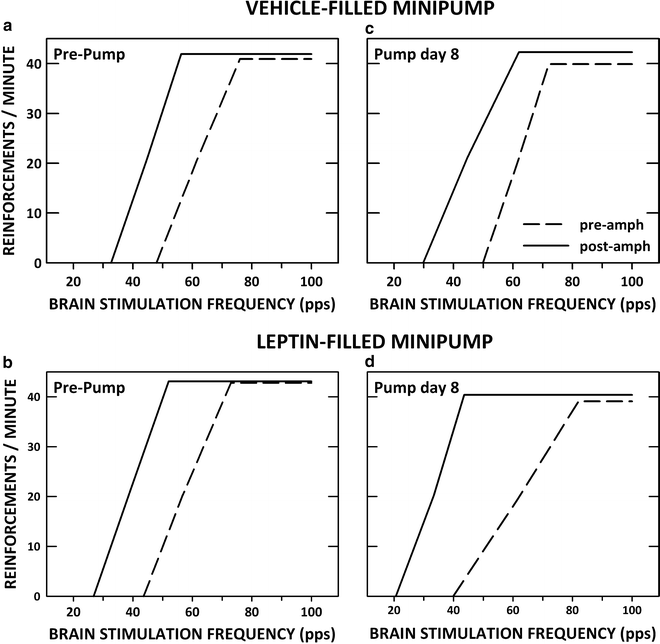
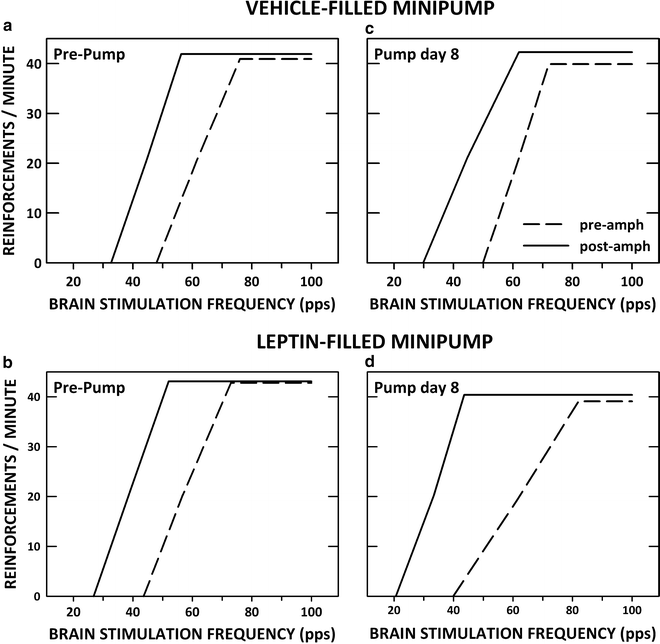
Fig. 4.
LHSS rate-frequency regression lines for two groups of ad libitum fed rats before (pre-amph) and after (post-amph) systemic injection of d-amphetamine (0.5 mg/kg, i.p.). (a) and (b) display results from the test session that preceded insertion of subcutaneous osmotic minipumps (Pre-Pump), while (c) and (d) show results obtained on the eighth day (Pump day 8) of continuous lateral ventricular infusion of vehicle (0.5 μL/h; a and c) or leptin (12.5 μg/0.5 μL/h; b and d).
3.4 Assessment of “Deprivation” Involvement in the Modulation of Reward by Food Restriction
To assess whether deprivation without weight loss alters the rewarding effect of d-amphetamine, ad libitum fed subjects were continuously infused in the lateral ventricle with the orexigenic melanocortin receptor antagonist SHU9119 (0.02 μg/0.5 μL/h) for 12 days (119). Half of the subjects had unlimited access to food and half were pair-fed to vehicle-infused controls. SHU9119 more than doubled daily food intake. Subjects that were pair-fed to vehicle-infused controls maintained body weights that were virtually identical to controls. Testing of d-amphetamine reward on days 3, 7, and 12 of the infusion revealed no alteration in SHU9119-infused rats that were pair-fed to controls. Thus, maintenance of baseline intake and body weight was associated with stable rewarding effects of d-amphetamine despite the fact that subjects were “deprived” relative to free-feeding SHU9119-infused subjects.
Although Sect. 3.3 casts doubt on the involvement of leptin in the modulation of reward by food restriction, there are numerous peripheral signals, known to interact with the brain DA system that could plausibly play a role in the mesoaccumbens neuroadaptations and increased drug reward sensitivity observed in food-restricted subjects. For example, when blood is sampled from food-restricted rats on a day and time when brains are typically harvested for biochemical assay, and within the time frame when behavioral data are collected, plasma corticosterone levels are elevated 5–10-fold, activated ghrelin levels are elevated by 75%, IGF-1 levels are decreased by 50%, and insulin levels are decreased by 80%, relative to ad libitum fed rats (Zheng et al., in preparation). The relationship between these sustained alterations, the mesoaccumbens DA system, and drug reward sensitivity, remain to be fully explored and are amenable to the strategies described for examining leptin and melanocortin involvement.
The representative findings outlined above suggest that a sustained decrease in food intake enhances the rewarding effects of psychostimulant drugs and D-1 DA receptor stimulation, and the effect may not require that the animal be hungry or deprived. In addition, downstream effects of NAc D-1 DA receptor stimulation on GluA1 phosphorylation and AMPA receptor trafficking may play a role in the enhanced rewarding effect with potential to mediate enduring changes in synaptic strength and behavior. Relevance of these findings to palatable food intake and the role of food restriction as a risk factor for binge pathology is suggested by findings that brief intake of sucrose also increases GluA1 phosphorylation and AMPA receptor abundance in the NAc postsynaptic density with a greater effect in food-restricted than ad libitum fed rats.
4 Conclusion
The experimental approaches described above are time consuming and labor intensive. However, they offer the possibility of identifying basal and receptor-stimulated molecular changes induced by food restriction that modulate reward. When used to illuminate results of biochemical studies, these behavioral assays may assist in the sorting of neuroadaptations that modulate responsiveness to acute reward stimuli versus those that may modulate synaptic plasticity and have the potential to ingrain maladaptive behavior, although the two categories are not mutually exclusive. To be clear, neuroadaptations induced by food restriction in the laboratory are assumed to be among those that facilitate adaptive behavior and survival when food is scarce in the wild. The potential for maladaptive outcome is hypothesized to be context dependent, as when severe dieting takes place in the presence of abundant, highly palatable, energy-dense food, when psychostimulant drugs are used as diet aids, or when drug abuse significantly displaces eating behavior. Mimicry or blockade of hormonal changes associated with chronic food restriction, using subcutaneous osmotic minipumps, may assist in the identification of physiological responses that are antecedent to CNS changes of interest. Of course, site-specific conditional gene modifications offer a range of powerful alternative approaches should mouse models be substituted for the rat model. The ultimate goals, on the basic research side, are to specify brain regional mechanisms that facilitate reward and synaptic plasticity during chronic food restriction, assign strong candidacy to those that have known functional involvement in drug addiction, and investigate their role in the genesis and maintenance of disordered eating behavior in animal models.
Acknowledgments
This research was supported by DA03956 from NIDA/NIH and a NARSAD Award to KDC.
References
2.
3.
4.
5.
Coelho JS et al (2010) Inaccessible food cues affect stress and weight gain in calorically-restricted and ad lib fed rats. Appetite 54:229–232PubMedCrossRef
< div class='tao-gold-member'>
Only gold members can continue reading. Log In or Register a > to continue
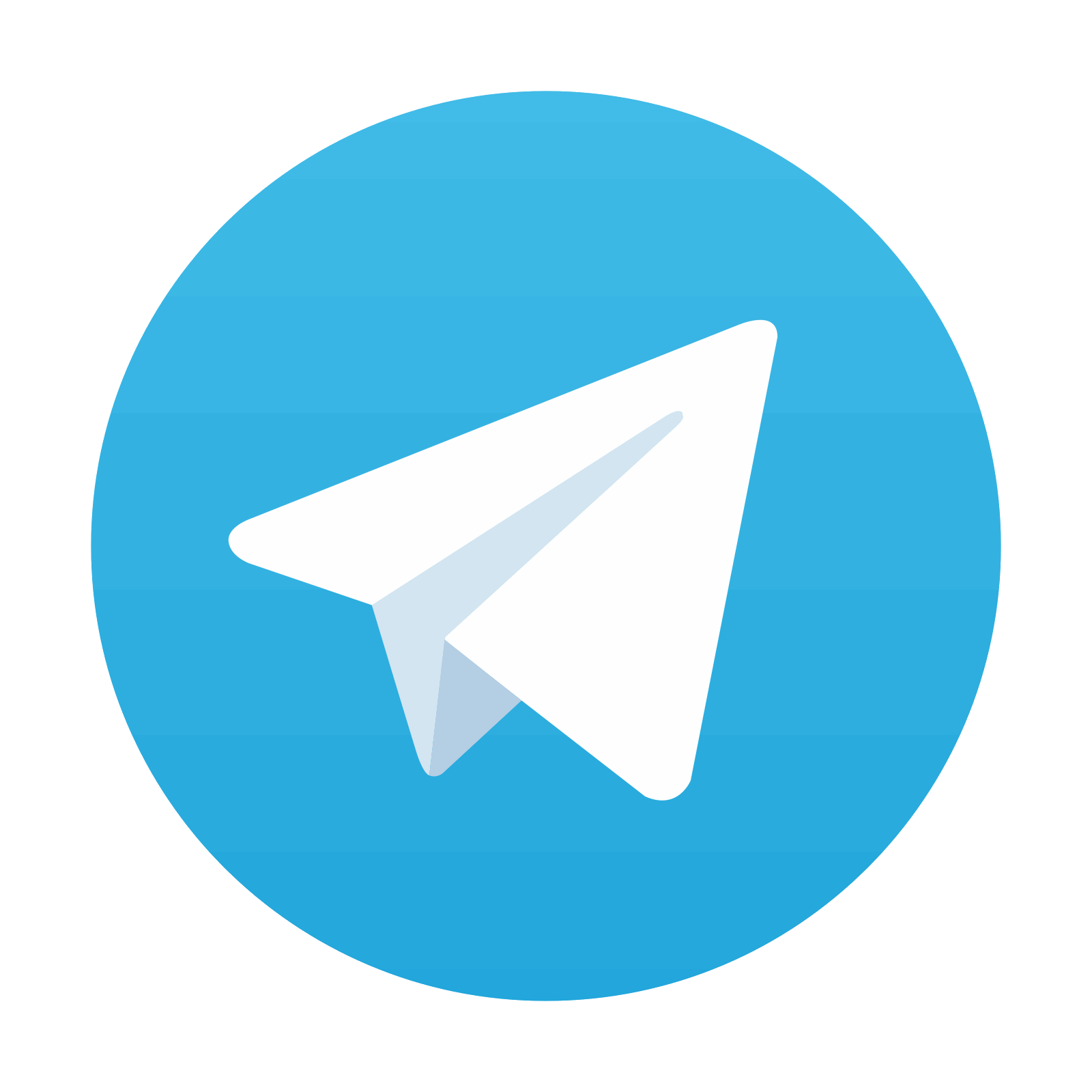
Stay updated, free articles. Join our Telegram channel

Full access? Get Clinical Tree
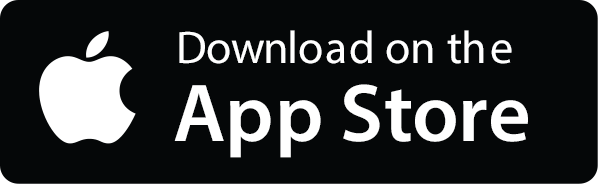
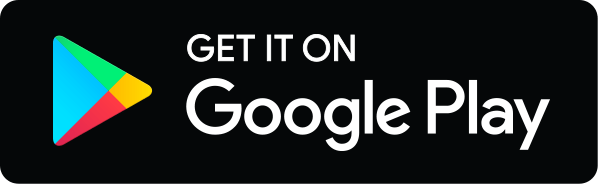