Chapter 18 Fluid and Electrolyte Disturbances In Gastrointestinal and Pancreatic Disease
The gastrointestinal tract (GIT) is extremely well adapted to the task of assimilating a wide variety of nutrients and absorbs approximately 99% of the fluid presented to it (Figure 18-1).15 Most of the fluid absorbed in the GIT each day is derived from endogenous secretions. Exogenous fluid in the form of food and water constitutes 30 to 50 mL/kg/day, and endogenous secretions from the salivary glands, stomach, pancreas, liver, and small intestine represent two to three times this volume or 1.5 to 2 blood volumes (blood volume represents 7% of body weight; see Figure 18-1). Considering this massive flux of fluid into the GIT, it is easy to see why fluid loss from or sequestration by the GIT can alter the electrolyte and acid-base status of the patient. The causes and consequences of fluid loss or sequestration are not uniform and do not depend solely on the region of the GIT involved. For example, the presence of hypochloremic, hypokalemic metabolic alkalosis is not necessarily indicative of proximal GIT obstruction. Twelve percent (9/74) of dogs with proximal GIT obstruction and 14% (7/51) of dogs with distal GIT obstruction had a hypochloremic, hypokalemic metabolic alkalosis.11 However, gastric losses virtually always are the precipitating cause of metabolic alkalosis in human patients with serum bicarbonate concentrations >45 mmol/L.40
Normal physiology of the gastrointestinal tract
Absorption and secretion of water and electrolytes
Stomach
Unstimulated acid secretion by the stomach in dogs and cats is minimal (e.g., <0.04 mmol/kg0.75/hr in the dog).45 The “acid pump” or H+, K+-adenosinetriphosphatase (H+, K+-ATPase) is located in tubulovesicles within the cytoplasm of parietal cells (Figure 18-2).105 In the stimulated state, H+,K+-ATPase and KCl transporters are incorporated in the parietal cell canalicular membrane (Figures 18-2 and 18-3). Hydrogen ions derived from the ionization of water within the parietal cells are transported into the gastric lumen in exchange for potassium ions. Potassium and chloride transporters in the canalicular membrane allow luminal transfer of potassium and chloride ions. Carbonic anhydrase catalyzes the combination of -OH−with CO2 to form , which diffuses into the blood (so-called alkaline tide). Acid secretion in dogs has been estimated at 30 mL/kg/day.15 Stimulation with pentagastrin results in a rapid increase in fluid and hydrogen ion secretion, with pH rapidly decreasing to less than 1.0. Acid secretion in dogs reaches a peak of 28 mL/kg0.75/hr or 4.1 mmol HCl/kg0.75/hr during this time. The surge in acid secretion transiently increases serum bicarbonate concentration by 1 to 2 mmol/L (in humans), a phenomenon referred to as the alkaline tide, and once used as a test of gastric acid secretion.40 Potassium transport reaches a peak of 0.34 mmol/kg0.75/hr and sodium transport a peak of 0.09 mmol/kg0.75/hr.45 The concentrations of K+ (10 to 20 mEq/L) and Cl− (120 to 160 mEq/L) in gastric juice are higher than those of plasma (approximately 4 mEq/L and 110 mEq/L, respectively, in the dog).
Acid secretion by parietal cells is regulated by a variety of neurochemical and neurohumoral stimuli.70,121 Luminal peptides, digested protein, acetylcholine, and gastrin-releasing peptide stimulate gastrin secretion from G cells and cause histamine release from enterochromaffin-like cells (see Figure 18-2). Histamine is also released from mast cells. Acetylcholine and gastrin can also directly stimulate parietal cells. Somatostatin acts to decrease gastrin, histamine, and acid secretion. Acid secretion can be decreased by blocking H2 (e.g., cimetidine, ranitidine, famotidine), gastrin (e.g., proglumide), and acetylcholine (e.g., atropine) receptors and by inhibiting adenyl cyclase (e.g., prostaglandin E analogs) and H+, K+-ATPase (e.g., omeprazole, pantoprazole, lansoprazole).
Pancreas
The exocrine pancreas plays a major role in the digestion of food. It secretes enzymes that digest a wide variety of foodstuffs and bicarbonate, which serves to solubilize secreted enzymes and neutralize gastric acid so that optimal enzyme activity is maintained. The anatomy of the communication between the exocrine pancreas and GIT differs between dogs and cats. The common bile duct (CBD) of the dog opens near the minor pancreatic duct at the major duodenal papilla, and the accessory pancreatic duct opens a few inches distally. In the cat, the CBD fuses with the pancreatic duct just before entering the duodenal papilla 3 cm caudal to the pylorus.36 However, the major pancreatic duct opens separately, but immediately adjacent to the CBD in some cats. In 20% of cats, the accessory pancreatic duct enters the duodenum (minor duodenal papilla) approximately 2 cm caudal to the major duodenal papilla.36 As a consequence of the close proximity of the pancreas and CBD, pancreatitis commonly influences bile flow through the major bile duct, causing obstruction to flow and jaundice. In the cat, inflammatory, neoplastic, or obstructive disorders involving the distal CBD can affect both the biliary tree and pancreas.18 Pancreatic secretions also play an important role in the absorption of cobalamin (vitamin B12) and in the regulation of the bacterial flora of the small intestine, and they directly influence small intestinal function by modifying certain enzymes on the intestinal brush border and exerting trophic effects on the mucosa.
Pancreatic acinar cells are responsible for the synthesis of digestive enzymes, whereas the cells lining the ductular system are the major source of fluid and electrolyte secretion. Entry of the acid gastric secretions into the duodenum signals the pancreas to secrete bicarbonate into the gut. The anion transporter primarily responsible for this process is a luminal membrane Cl− / exchanger (see Figure 18-3).40 The activity of this ion exchanger is regulated by the cystic fibrosis transmembrane conductance regulator (CFTR) Cl−channel, which recycles Cl−across the luminal membrane.40,89 Under maximal stimulation, some secreted
seems to enter the lumen directly via the CFTR channel as well as by Cl−/
exchange.89 The electrolyte composition of pancreatic secretion changes in response to stimulation. At low rates of secretion, the chloride concentration exceeds that of bicarbonate, whereas at higher rates, the bicarbonate concentration is higher than the chloride concentration (Figure 18-4). Entry of acid gastric secretions into the duodenum signals the pancreas to secrete its alkaline solution into the gut.40 In the stimulated cat pancreas, the
concentration increases from 70 to 145 mEq/ L and the Cl− concentration decreases from 100 to 30 mEq/L. Concentrations of Na+ and K+ in pancreatic secretions are similar to those of plasma. The concentrations of electrolytes also change within the pancreatic ductular system. A decrease in Cl− concentration from the intralobular ducts to the main ducts is thought to arise through the exchange of Cl− for
. Secretin-releasing factor (SRF) found in canine pancreatic juice increases plasma secretin concentration and stimulates pancreatic secretion in dogs, suggesting that a positive feedback mechanism may be involved in the regulation of pancreatic secretion.122 Secretin is the principal mediator of pancreatic fluid and electrolyte secretion (Figure 18-5) and is released in response to acidification of the proximal small intestine. Secretin and cholecystokinin have synergistic effects on fluid and electrolyte secretion. Bicarbonate is responsible for solubilizing zymogens within the pancreatic ductular system and neutralizing gastric acid in the duodenum to provide an optimal pH for pancreatic enzyme activity. Feeding is the most important factor controlling pancreatic secretions. Some drugs (e.g., morphine) have been implicated in halting pancreatic bicarbonate secretion and protein response to feeding.64 Acetylcholine inhibition due to morphine administration has been one of the mechanisms implicated in these effects. Morphine also may cause increased muscular tone in the sphincter of Oddi and a similar effect also may occur in the pancreatic duct.135
Pancreatic duct cells also produce intrinsic factor (IF), which is a protein necessary for the absorption of cobalamin (vitamin B12).6 Dogs, unlike cats, also produce IF in the stomach.5,6,39,116 Although the IF that originates in the stomach is considered partially responsible for mediating the absorption of cobalamin,5 the amount of IF produced by the stomach of dogs is considered minimal compared with the amount produced by the pancreas (possibly fivefold to tenfold less).6
Intestine
Net absorption of fluid and electrolytes in the intestine reflects a balance between absorption and secretion, and the final outcome in the healthy intestine represents a victory for absorption. The ability of the intestine to absorb fluid and electrolytes varies according to site. In a 20-kg dog, approximately 2.70 L of fluid (oral intake, stomach juice, saliva, pancreatic juice, and bile) is presented to the small intestine each day. Approximately 1.35 L is absorbed in the jejunum, 1 L in the ileum, and 300 mL in the colon, with 50 mL remaining in the feces.123 From these figures, it can be calculated that the jejunum absorbs 50%, the ileum 38%, and the colon 11% of the fluid presented to the intestinal tract (Figure 18-6). Approximately 1% of the water presented to the GIT is lost in feces. The variation in absorptive efficiency along the intestinal tract is a function of enterocyte pore size, membrane potential difference, and the type of transport processes associated with each intestinal segment.20,82,120 Whereas the jejunal epithelium is “leaky” and transfers a large amount of fluid (isotonic absorption), the tight epithelial junctions of the distal colon allow a high transepithelial voltage gradient to develop, and net solute transfer occurs against this gradient.120
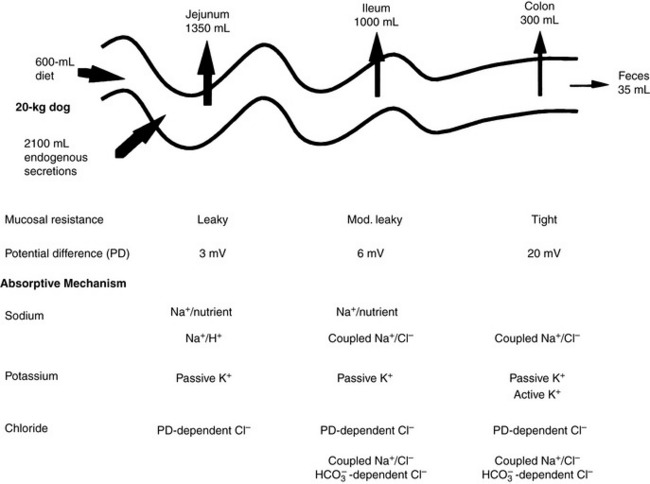
Figure 18-6 Fluid and electrolyte absorption in the gastrointestinal tract.
(Adapted from Burrows CF. Chronic diarrhea in the dog. Vet Clin North Am 1983;13:521; Chang EB, Rao MC. Intestinal water and electrolyte transport: mechanisms of physiological and adaptive responses. In: Johnson LR, editor. Physiology of the gastrointestinal tract, 3rd ed. New York: Raven Press, 1994: 2027–2081; and Sellin JH. Intestinal electrolyte absorption and secretion. In: Sleisinger MH, Fordtran JS, Feldman M, et al, editors. Gastrointestinal and liver disease pathophysiology, diagnosis and management, 6th ed, Vol 2. Philadelphia: WB Saunders, 1998: 1451–1471.)
The absorption of water is passive in the small and large intestines and follows the transport of solutes across the intestinal epithelium.20 Passive absorption of water or electrolytes can be transcellular (i.e., through the cytoplasm of the cells) or paracellular (i.e., via the lateral intercellular spaces and tight junctions between enterocytes), and transfer occurs down a chemical or electrical gradient (e.g., passive transport of Na+ and Cl− in the jejunum and ileum).
Active transport involves transport against a concentration gradient and requires energy input (e.g., Na+ transport driven by the Na+, K+ pump). The Na+, K+-ATPase is present in all enterocytes (see Figures 18-3 and 18-6) and maintains the electrochemical Na+ gradient required not only for net transepithelial Na+ movement but also for the transport of many other solutes.120 Solvent drag is the term used to describe solute movement secondary to water flow (e.g., NaCl transport in the jejunum via the paracellular route). The relative importance of each transport system is site dependent (see Figures 18-3 and 18-6), and the location of the enterocyte in the villus or crypt is also important: villus enterocytes absorb, whereas crypt enterocytes secrete.
Jejunum
Because of the high permeability of the jejunum, passive transport processes make a major contribution to overall Na+ and Cl−movement in this segment of the intestinal tract. Absorption is driven by Na+ and Cl− uptake (which in turn drives water uptake) via two linked transporters, a Na+-H+ exchanger and a Cl−– exchanger (see Figure 18-3).40 These two transporters take up Na+ and Cl− from the gut lumen and secrete H+ and
into the lumen.40 In humans, the Cl−–
exchanger in the small intestine is the “downregulated in adenoma” (DRA) gene product, which generates an alkaline solution. DRA is now known to be abundantly expressed in colonocytes, but not enterocytes, and transports more than 2 Cl− ions to 1
ion.120 Transport of many hydrophilic nutrients including glucose, amino acids, and some vitamins occurs against concentration gradients by secondary active transport across the luminal membrane and by facilitated diffusion across the basolateral membrane.120 The luminal membranes of the epithelial cells in this region contain sodium-dependent transporters for hexose sugars and amino acids (see Figure 18-6). Sodium enters the epithelial cell down its concentration gradient and is the driving force for accumulation of the nutrient intracellularly. Glucose and glutamine supply energy. The entry of sodium and glucose promotes water absorption. Sodium then is extruded from the cell at the basolateral membrane by Na+, K+-ATPase while the hexose sugar or amino acid diffuses out of the cell at the basolateral membrane down a favorable concentration gradient. Therefore Na+, K+-ATPase drives net absorption of both Na+ and the sugar or amino acid. Proteins that function as Na+-H+ exchangers are present in the luminal membranes of the enterocytes in the jejunum and allow absorbed Na+ to be exchanged for intracellular H+ (see Figures 18-3 and 18-6). This exchange is driven by both the electrochemical gradient for Na+ and a pH gradient that results from a moderately acidic intracellular environment.120 As Na+ is extruded at the basolateral membrane by Na+ K+-ATPase,
also moves out of the cell, resulting in net absorption of sodium bicarbonate. Although Na+-nutrient absorption and Na+–
absorption occur in the jejunum as already described, solvent drag-mediated Na+ absorption secondary to monosaccharide absorption is the major mechanism for Na+ absorption in this segment.
Movement of K+ in the intestinal tract follows its electrochemical gradient, and secretion generally predominates.120 In the small intestine, most K+ secretion is passive (a luminal K+ channel is lacking) and results from the generation of a lumen-negative potential difference across the epithelium.120 This negative potential difference attracts K+ into the intestinal lumen, and consequently the concentration of K+ is always higher in intestinal contents than in plasma. In the jejunum, solvent drag created by glucose and amino acid transport causes passive absorption of Cl− and .40
Ileum
The predominant form of Na+ absorption in the ileum is neutral NaCl absorption (see Figure 18-6) with some contribution by Na+-nutrient absorption. The contents of the ileum and colon are normally alkaline.33,123 Solvent drag-mediated passive absorption of Cl− and does not occur in the ileum and colon, but a Cl− –
exchange mechanism is present.40 Bicarbonate is secreted by electrogenic and electroneutral processes in the duodenum, ileum, and colon. Being a metabolic product, intracellular
can arise from intracellular metabolism, diffusion of CO2 or the action of transporters such as the basolateral Na+–
cotransporter.120
Colon
Absorption of Na+ in the colon is achieved against a large electrochemical gradient (see Figure 18-6) and is principally a result of active Na+ transport.38 Colonic Na+ absorption is also influenced by mineralocorticoids (e.g., aldosterone).40 Mineralocorticoids increase the activity of Na+ channels in the luminal membranes of colonic epithelial cells and may increase Na+, K+-ATPase in the basolateral membranes. The colonic epithelium contains K+ channels and is capable of active potassium transport (see Figure 18-3).40 Absorption of potassium is thought to be accomplished by means of a K+-ATPase with characteristics similar to those of both basolateral membrane Na+, K+-ATPase and parietal cell H+, K+-ATPase.10,120 The concentration of K+ in colonic contents is high because of the high potential difference generated and can approach 90 mEq/L.38 Active K+ secretion is mediated by Na+, K+-ATPase or by Na+-K+-2 Cl− cotransport. Aldosterone and cyclic adenosine monophosphate (cAMP) increase luminal K+ conductance and stimulate secretion of K+ (see Figure 18-6).10 Colonic absorption is important in small-intestinal disease because the colon may compensate for fluid losses associated with small-bowel dysfunction. Alternatively, patients with small-bowel dysfunction may have signs of large-bowel disease. This is thought to result from impairment of colonic absorption or stimulation of colonic secretion by products of abnormal small-intestinal function, such as hydroxylated fatty acids or deconjugated bile acids.
The primary anions in the colon are short-chain fatty acids, which are generated by bacterial metabolism of carbohydrate and protein.120 Some of the organic anions are absorbed by a linked exchange transporter (see Figure 18-3). These short-chain fatty acids include acetate, propionate, and butyrate, which are the preferred metabolic substrates for colonic cells. They are known to stimulate Na+, water, and K+ absorption by the colon, but the exact mechanism of this process has not been defined.104
Intestinal secretion is a function of villus crypt cells. It is thought the electrogenic transport of Cl− across the basolateral membrane into the enterocyte and Cl− efflux through Cl− channels in the microvillus membrane into the intestinal lumen (Figure 18-7) cause intestinal secretion.
Control of absorption and secretion of water and electrolytes
Control of absorption and secretion is an autonomous process that is regulated by the neurocrine systems located in the submucosal plexus.21,22,82,120 Acetylcholine and vasoactive intestinal polypeptide (VIP) are the major mediators of gastrointestinal secretion, whereas norepinephrine, somatostatin, and opioids are the principal regulators of absorption. At the cellular level, acetylcholine and VIP cause an increase in intracellular calcium and cAMP that inhibits neutral NaCl absorption and facilitates transcellular Cl− efflux. Many bacterial agents exert their effects by increasing the intracellular concentration of cAMP in enterocytes. Norepinephrine, somatostatin, and opioids lower intracellular cAMP and calcium concentrations and stimulate neutral NaCl absorption (see Figure 18-7).
Volume status and intestinal blood flow also influence ion transport. Systemic volume expansion results in an increase in intestinal secretion, whereas volume contraction results in adrenergic stimulation and increased absorption.20 Osmotic forces are also important in the regulation of electrolyte and fluid transport. Luminal osmolality is normally maintained close to plasma osmolality.20 After intake of hypertonic foods and liquids, rapid equilibration is accomplished by movement of water into the intestinal lumen. In particular, the duodenum and upper jejunum are subject to major fluid shifts. As intestinal chyme moves distally, absorptive processes steadily decrease luminal Na+, Cl−, and water. Osmotic diarrhea results if nonabsorbable solutes such as disaccharides remain in the lumen. Increased fluid absorption in the colon can compensate to some extent for fluid lost into the lumen of the small bowel, but eventually colon absorptive capacity is overwhelmed. Cations such as magnesium and anions such as sulfate are poorly absorbed and can also lead to osmotic diarrhea.
In response to inflammation, the number of immune cells in the lamina propria increases. Inflammation can lead to mucosal ulceration, exudation of protein, motility dysfunction, and loss of absorptive surface area, all of which can result in intestinal fluid loss. Many secretagogues associated with inflammation have been identified. Adenosine, serotonin, and histamine have both direct effects on epithelial cells and indirect effects via neural pathways. Other secretagogues include oxidants (e.g., superoxides, hydrogen peroxide, and OH− released from neutrophils) that stimulate Cl− secretion, cytokines (e.g., interleukin-1, interleukin-3), arachidonic acid, platelet-activating factor, substance P, kallikreins, and bradykinin.120Escherichia coli heat-labile enterotoxin and enterotoxins produced by Vibrio cholerae, Salmonella sp., Campylobacter jejuni, Pseudomonas aeruginosa, and Shigella sp. activate adenylate cyclase, producing cAMP and augmenting secretion in the intestine (see Figure 18-7).44 The eicosanoids, especially the lipoxygenase metabolites of arachidonic acid, are central to the secretory response associated with inflammation. Kinins stimulate secretion in both the small and large intestines, where they stimulate production of prostaglandin E2.85
Acid-base balance may also affect intestinal electrolyte transport. In the rat, metabolic acidosis is a potent stimulus for ileal Na+ absorption (possibly in exchange for H+), whereas metabolic alkalosis decreases Na+ absorption but increases secretion.20
Pathophysiology of the gastrointestinal tract
Vomiting
Vomiting is a reflex act that is initiated by stimulation of the vomiting center in the reticular formation of the medulla oblongata. The vomiting center can be stimulated directly or indirectly via the chemoreceptor trigger zone (CRTZ), which is situated in the area postrema near the floor of the fourth ventricle (Figure 18-8).14,34,35,61 The blood-brain barrier is limited at this point, enabling blood-borne substances such as toxins or drugs to stimulate the CRTZ, causing a reflex action to expel ingested toxins, and this reflex represents a long-preserved evolutionary survival technique for many species.14 Neurologic input from the vestibular nucleus can also stimulate the CRTZ in the dog or the vomiting center. 14,34,35,61 Disease or irritation of the GIT, abdominal organs, or peritoneum and cerebral diseases can directly stimulate the vomiting center via visceral receptors and vagal afferents (Figure 18-8). 14,34,35,61
Once the vomiting center is adequately stimulated, a series of visceral events is initiated. This sequence of events includes inhibition of proximal gastrointestinal motility, a retrograde power contraction in the small intestine, and antral relaxation, which enables the transfer of intestinal contents to the stomach. These events are followed by moderate-amplitude contractions in the gastric antrum and intestine, and shortening of the intraabdominal esophagus. Dilatation of the cardia and lower esophageal sphincter enables transfer of gastric contents to the esophagus during retching and vomiting. Retching often precedes vomiting and is characterized by rhythmic inspiratory movements against a closed glottis. Negative intrathoracic pressure during retching prevents expulsion of esophageal contents. During vomiting, the abdominal muscles contract and intrathoracic and intraabdominal pressures are positive, resulting in forceful expulsion of gastric contents from the mouth.34
Vomiting is associated with a wide range of disease processes and, when frequent or severe, can have major effects on fluid, electrolyte, and acid-base balance. The consequences of vomiting depend to some extent on the cause. Vomiting of gastric and intestinal contents usually involves loss of fluid containing Cl−, K+, Na+, and , and dehydration is accompanied to a variable extent by hypochloremia, hypokalemia, and hyponatremia.34,127 Metabolic acidosis is generally more common than metabolic alkalosis in dogs with gastrointestinal disease.23
With obstruction of the gastric outflow tract or proximal duodenum, loss of Cl− can exceed that of , and hypochloremia, hypokalemia, and metabolic alkalosis result.23,100,127 In human patients with serum bicarbonate concentrations greater than 45 mmol/L, gastric losses are virtually always the precipitating cause of the metabolic alkalosis.40 However, the presence of hypochloremic metabolic alkalosis is not always an indication of proximal GIT obstruction, and it may develop just as often in distal GIT obstruction.11 Metabolic alkalosis is self-perpetuating because of increased renal reabsorption of NaHCO3 in the presence of volume, chloride, and potassium depletion.102 These metabolic disturbances arise as a result of preferential conservation of volume at the expense of extracellular pH. The renal reabsorption of almost all filtered
and the exchange of sodium for hydrogen ions in the distal tubule promote an acidic urine pH despite extracellular alkalemia (so-called paradoxical aciduria).102,129
Metabolic alkalosis in patients with gastrointestinal signs is not invariably associated with outflow obstruction and has been encountered in dogs with parvovirus enteritis and acute pancreatitis.48 Diseases characterized by hypersecretion of acid, such as gastrinoma, may also be associated with metabolic alkalosis and aciduria. Basal gastric acid secretion in two dogs with gastrin-producing tumors (1.7 and 2.7 mmol/hr/kg0.75 HCl) was maximal in the unstimulated state.115 In this situation, hypochloremia, hypokalemia, metabolic alkalosis, and dehydration are probably caused by gastric hypersecretion of acid.115
Diarrhea
The pathomechanisms in patients with diarrhea include increased intestinal secretion, decreased intestinal absorption, rapid transit of intestinal contents, and mesenteric, vascular, or lymphatic disease.91
Secretory agents include neuropeptides of the enteric system (found in neuroendocrine tumors), cholinergic agonists, gastrointestinal hormones, bacterial enterotoxins, deconjugated bile acids, and hydroxy fatty acids.91 Secretory diarrhea results when prosecretory stimulation overwhelms absorptive forces. Secretory diarrhea is manifested by increased colonic secretion of sodium-rich fluid. Volume depletion resulting from sodium and water loss stimulates antidiuretic hormone release, which in turn stimulates water retention by the kidneys and dilutional hyponatremia.
Decreased intestinal absorption may result from decreased intestinal surface area as a consequence of damage by infectious agents (e.g., parvovirus), cellular infiltration, or surgery. Damage to the intestinal epithelial barrier may also increase intestinal permeability, disrupting paracellular and transcellular absorptive pathways. An increase in the osmolality of intestinal contents may also decrease absorption. Specific causes of osmotic diarrhea include overeating, sudden dietary change, osmotic laxative ingestion, maldigestion, or malabsorption. Absorption of water and electrolytes is retarded by accumulation of nonabsorbable solutes in the gut lumen, and there is net water movement from plasma to the gut lumen. In osmotic diarrhea, the concentration of sodium in the stool may remain below that of plasma, leading to water loss in excess of sodium, dehydration, and hypernatremia, especially when water intake is inadequate.38 This finding has been observed in dogs and people with hepatic encephalopathy (HE) treated with lactulose.88 Intestinal absorption may also be affected by diseases that cause increased venous pressure, lymphatic pressure, decreased interstitial osmotic pressure (hypoalbuminemia), and increased epithelial permeability. Disorders of intestinal motility result in decreased intestinal fluid absorption because of decreased contact time between luminal contents and the enterocytes.
Fluid and Electrolyte Abnormalities in Diarrhea
The fluid and electrolyte abnormalities associated with diarrhea include volume depletion, hyponatremia or hypernatremia, hypokalemia, and metabolic acidosis.23,82,127 The metabolic acidosis that develops is characterized by hyperchloremia and a normal anion gap caused by loss of diarrheal fluid with relatively low chloride and high bicarbonate concentrations. Serious electrolyte and acid-base abnormalities are relatively uncommon in patients with diarrhea as a sole complaint. When diarrhea is severe and protracted or is accompanied by vomiting, acid-base and electrolyte disturbances are more likely, but it is difficult to predict which abnormalities will be present. For example, decreased total CO2 concentrations were identified in less than 17% of 134 dogs with parvovirus enteritis in one study,55 whereas metabolic alkalosis and hypochloremia were more common than metabolic acidosis in another study of dogs with parvovirus enteritis.48 Another study of 22 dogs infected with parvovirus identified lower plasma concentrations of sodium, potassium, chloride, and bicarbonate than controls. Serum L-lactate concentrations were increased in some puppies with canine parvoviral enteritis, but most affected puppies developed only mild compensated metabolic acidosis.87 Antiinflammatory and immunosuppressive dosages of corticosteroids can result in type B hyperlactatemia (secondary to increased gluconeogenesis from protein).12 Therefore, caution must be taken when interpreting blood lactate concentrations in dogs with hyperadrenocorticism, in those receiving corticosteroids, or in those affected by chronic illness (i.e., due to endogenous hypercortisolemia) because these situations may lead to type B hyperlactatemia that does not require aggressive treatment as does hyperlactatemia caused by hypoperfusion (type A hyperlactatemia).
Hypoadrenocorticism should be ruled out whenever hyperkalemia and hyponatremia are present, but up to 26% of dogs with hypoadrenocorticism may have normal mineralocorticoid function and normal Na+/K+ ratios.68 Therefore a history of intermittent signs of gastrointestinal disease may warrant an adrenocorticotropic hormone stimulation test despite a normal Na+/K+ ratio. Clinicopathologic findings in dogs with primary gastrointestinal disease may mimic those of hypoadrenocorticism with Na+/K+ ratios less than 27:1 and metabolic acidosis.30,73,74 Gastrointestinal diseases reported to mimic primary hypoadrenocorticism include trichuriasis, ancylostomiasis, salmonellosis, and perforated duodenal ulcer. Postulated mechanisms of these electrolyte derangements include metabolic acidosis secondary to volume depletion and fecal loss of bicarbonate with subsequent translocation of potassium from intracellular to extracellular fluid. Selective aldosterone deficiency does not appear to be responsible for hyperkalemia and hyponatremia in dogs with trichuriasis that have laboratory abnormalities that mimic those of hypoadrenocorticism.41 Hypocalcemia and hypomagnesemia occur uncommonly in veterinary patients with diarrhea and are most often encountered in animals with protein-losing enteropathy. Ionized hypocalcemia and hypomagnesemia have been described in Yorkshire terriers with diarrhea, abdominal effusion, leukocytosis, neutrophilia, hypoproteinemia, hypoalbuminemia, hypocholesterolemia, and increased serum activity of aspartate aminotransferase. The hypomagnesemia and hypocalcemia potentially were the result of intestinal loss, malabsorption, and abnormalities of vitamin D and parathyroid hormone metabolism.59 Another two dogs with protein-losing enteropathies were reported to have low ionized calcium, low 25-hydroxyvitamin D, low 1,25-dihydroxyvitamin D, and high parathyroid hormone concentrations. These abnormalities were thought to be a result of decreased intestinal absorption of 25-hydroxyvitamin D.76 Furthermore, ionized hypocalcemia has been noted in critically ill dogs admitted to intensive care units and these dogs were predicted to have longer intensive care and hospitalization.52 These dogs had a wide variety of gastrointestinal diseases (e.g., gastric dilatation-volvulus [5], septic peritonitis [2], gastrointestinal perforation [2], hemorrhagic gastroenteritis [1], gastrointestinal granuloma [1], gastrointestinal ulceration [1], and esophageal foreign body [1]).52
Normal stool water contains a higher concentration of potassium than sodium.38 As stool volumes increase in human patients with diarrhea, there is a progressive increase in the sodium and chloride concentrations and a decrease in potassium concentration, and the electrolyte composition of the stool approaches that of plasma.38 In cases of diarrhea, there is a linear relationship between fluid and sodium loss.38 In human patients, measurements of fecal electrolyte concentrations and osmolality are used to calculate an osmolality gap, which aids in the differentiation of osmotic and secretory diarrhea. The osmolality, sodium concentration, and potassium concentration of feces from four normal cats were reported as 622 to 927 mOsm/kg, 27 to 57 mEq/L, and 19 to 46 mEq/L, respectively.42 Stool normally contains high concentrations of potassium, and fecal potassium loss can become severe in protracted diarrheal states.38 Stimulation of the renin-aldosterone axis as a result of volume depletion has been suggested as one potential cause of potassium loss.82
Dogs with gastrin-secreting tumors may be presented for evaluation of intermittent or profuse watery diarrhea, vomiting, and weight loss. In this setting, diarrhea and vomiting are probably a result of the increased volume and acidity of gastric secretions, which cause gastrointestinal ulceration and destruction of pancreatic enzymes.115 The high concentrations of gastrin may also adversely affect intestinal function.
In humans, profuse watery intermittent or fulminant diarrhea can be caused by tumors secreting VIP (VIPomas).65 The majority of these tumors are located in the pancreas, although the VIPoma syndrome has been associated with tumors at a number of other sites in humans. Additional findings include severe hypokalemia, metabolic acidosis, and occasionally hypochlorhydria. The profound secretory diarrhea results from the stimulatory action of VIP on intestinal secretion. The VIPoma syndrome differs from gastrinoma in that patients with VIPoma have normal serum gastrin concentrations and lack gastric acid hypersecretion and secondary upper GIT ulceration. Increased concentrations of VIP and motilin (VIP, 927 pg/mL; normal range, 0 to 84 pg/mL; motilin, 341 pg/mL; normal range, 0 to 125 pg/ mL) have been documented in a dog with severe diarrhea and a heart base tumor. The dog had metabolic acidosis, hypokalemia, a normal anion gap, and no evidence of renal potassium wasting (Richter and Simpson, unpublished observations).
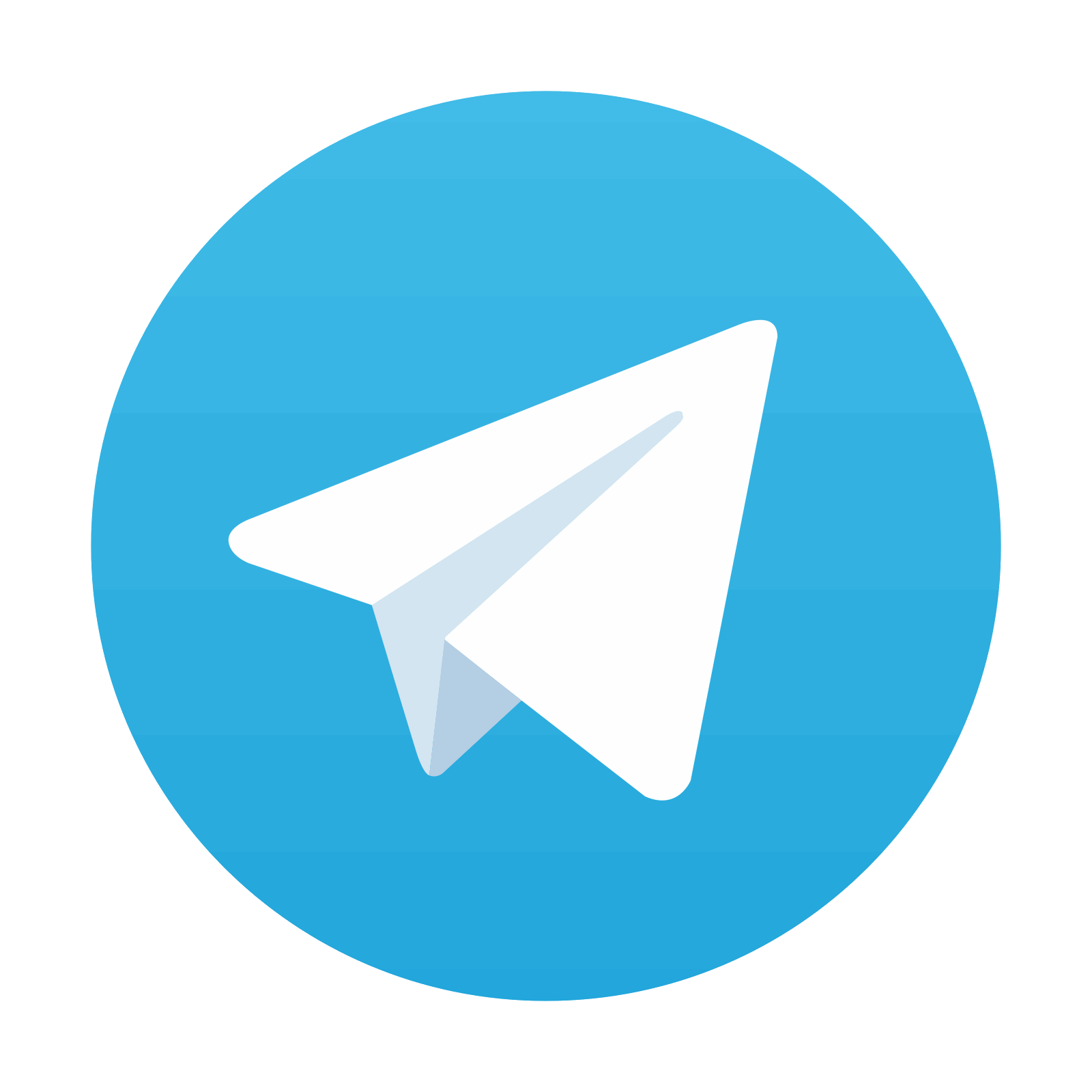
Stay updated, free articles. Join our Telegram channel

Full access? Get Clinical Tree
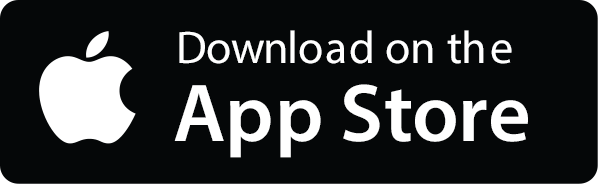
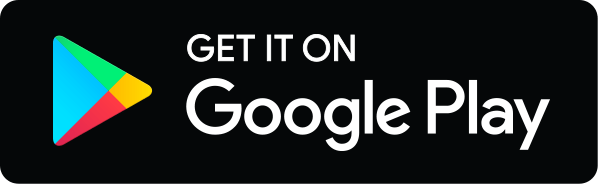