Chapter 8 Disorders of Magnesium
Magnesium Deficit and Excess
Historically, magnesium has received very little attention in veterinary medicine as an electrolyte worthy of consideration. Studies conducted in animal models in the early twentieth century documented the devastating effects of dietary magnesium deficiency in dogs.25,26,117,171 Dietary magnesium became a topic of interest in the 1970s and 1980s as a potential risk factor for cats with struvite urolithiasis and urethral obstruction.31,93,127 Today, following significant study of the syndrome in cats and identification of numerous other risk factors, magnesium in the diet is no longer considered a risk factor for the formation of feline urolithiasis.23,24,56,91 Since that time, magnesium has been the focus of very little research when compared with other electrolytes.
Magnesium regulation and balance
Distribution of magnesium
The precise distribution of magnesium in the bodies of dogs and cats under differing conditions has not been well studied. The distribution of magnesium within the bodies of human beings has been documented more effectively. In humans, current estimates suggest that only about 1% of the total body magnesium is located outside the cell in the extracellular fluids and that the remaining 99% is located in intracellular stores.126 Approximately two thirds (67%) of body magnesium is stored in the bone with calcium and phosphorus, 20% is found in muscle tissue, and 11% in other soft tissues not including muscle.124 Bone and muscle account for the major intracellular stores of magnesium in humans. Exchange between intracellular magnesium and the extracellular fluid is difficult to study, but current estimates suggest that only 15% of these stores are considered to be exchangeable with the extracellular fluid. It appears that bone, muscle, and red blood cell stores of magnesium are very slow to liberate magnesium to the extracellular pool, and that soft tissues are much more able to liberate magnesium to the extracellular space in humans.126 In dogs, however, similar radioisotope studies suggest that bone magnesium is the most labile pool and will be scavenged during a magnesium deficit.16 Regulatory control of magnesium shifts between intracellular and extracellular spaces is poorly understood and is likely to be complex and multifactorial. Extracellular magnesium is present in three forms (like calcium): an ionized or free form (55%) that is believed to constitute the biologically active fraction, a protein bound form (20% to 30%) and a complexed form (15% to 25%). Unlike calcium, which is approximately 40% protein bound, magnesium is only 20% to 30% bound to protein and so is less affected by changes in albumin concentration.90,124 Inside the cell, magnesium is complexed to many organic compounds where it plays a pivotal role. Current estimates indicate that only about 1% to 2% of the intracellular magnesium is present in the ionized or free form. Presumably, magnesium also shifts between the free and complexed intracellular forms as well, but the precise regulatory mechanisms governing those shifts are not understood at this time.
Gastrointestinal handling of magnesium
The primary site of magnesium absorption appears to be the ileum, but the jejunum and colon also contribute substantially to net absorption.70,71 The mechanisms of magnesium absorption from the ileum are the most well studied at this time. Much research remains yet to completely understand the complexities of gastrointestinal magnesium absorption. Several key mechanisms are currently well understood. Two pathways for intestinal magnesium absorption exist: an unsaturable passive paracellular route and a saturable active transcellular route (Fig. 8-1).70,71,80 The paracellular movement of magnesium occurs through the tight junctions between epithelial cells. The driving forces for paracellular magnesium movement are: transepithelial magnesium concentration gradient, the transepithelial voltage gradient formed by salt and water absorption, and the permeability of the tight junctions to magnesium.81 The transepithelial concentration gradient generally favors absorption of magnesium from the gut and is influenced by the gut intraluminal ionized magnesium concentration (chelated or complexed magnesium species do not contribute). Thus, total dietary intake of magnesium and intake of dietary constituents that influence the amount of magnesium that is complexed or chelated may influence the net absorption of magnesium. The transepithelial voltage gradient is created by net movement of salt and water. A small positive intraluminal voltage results in a small force favoring transepithelial cation movement. Solvent drag created by sodium and water reabsorption will also result in transepithelial movement of magnesium and other ions. Water and salt reabsorption from the gut therefore has a significant influence on magnesium absorption.
Active transcellular magnesium movement from the gut is an area of very recent and exciting discovery. Study of numerous inherited conditions of impaired magnesium handling in humans led to an improved understanding of magnesium transport across the gut epithelium and a hypothesis that several magnesium transport proteins exist in both the luminal and basolateral cell membranes of gut epithelial cells.35 Identification of two very unique ion channels has only recently occurred. A unique family of genes called the transient receptor potential (TRP) family codes for both proteins. Both proteins are in the M subfamily and are labeled TRPM6 and TRPM7, respectively. TRPM6 and TRPM7 are found extensively in membrane surfaces of the small intestine, colon, and distal collecting tubules of the kidneys, all sites that are involved in magnesium regulation.* These two proteins are unique because they are the only known ion channels that combine a protein channel with an intracellular protein kinase or enzyme. As a result, much speculation has occurred about the role of the attached enzyme in magnesium homeostasis.86,105,149 Magnesium-adenosine-triphosphate (Mg-ATP) appears to be the substrate for the enzyme portion of these channels resulting in inhibition of magnesium entry through the channel into the cell.106 Physiologists generally agree that increasing intracellular levels of magnesium are able to thus inhibit intracellular entry of magnesium through this mechanism and that this constitutes the energy requiring active transport mechanism of transepithelial magnesium transport.5,32,148
In the kidneys, transcellular and paracellular magnesium transport mechanisms are influenced by calcium and several additional hormones. It is likely that similar control mechanisms influence magnesium reabsorption in the gut. The specific actions of each of these influences and the precise mechanisms of action have yet to be established. The influence of calcium and hormones on the renal handling of magnesium is much better studied and understood. Some evidence does exist, however, to support the positive influence of parathyroid hormone (PTH) and 1,25-dihydroxycholecalciferol (1,25[OH]2D3) levels on reabsorption of magnesium from the gut.69,88
Renal handling of magnesium
Proximal Tubule
Approximately 80% of total serum magnesium is filtered by the glomerulus and enters the proximal tubule. Studies in numerous mammalian species have documented that approximately 10% to 15% of magnesium is reabsorbed within the proximal tubule.123 This is in sharp contrast to most other major cations, where at least 60% of reabsorption occurs in the proximal tubule. The reabsorption process in this segment of the nephron appears to occur via passive and unsaturable mechanisms and is unchanged by numerous other factors that play a role in other nephron segments. Based on available data, absorption of magnesium in this tubular segment appears to occur through paracellular transport but the precise mechanism is not known.
Loop of Henle
The loop of Henle is the site of the majority of magnesium absorption from the kidneys. Approximately 60% to 70% of filtered magnesium is reabsorbed in the cortical thick ascending limb of the loop of Henle.123,144 The medullary thick ascending limb does not appear to participate in magnesium balance.153 Evidence gathered to date indicates that magnesium absorption in this segment occurs via the paracellular pathway through tight junctions between renal epithelial cells. Numerous factors may influence the transport of magnesium (Fig. 8-2). The principle force allowing magnesium transport in the loop, as in the gut, appears to be the electropositive luminal environment created by the movement of sodium and chloride from the lumen to the interstitial space.123 In addition, magnesium movement through the tight junctions occurs due to “solvent drag” created by the salt and water movement. The positive intraluminal charge facilitates movement of magnesium (and calcium) from the lumen to the interstitium through a paracellular “pore” or channel. Recently, a tight junction protein called PCLN-1 or claudin-16 was discovered that is the primary divalent cation channel permitting paracellular movement of magnesium and calcium in the thick ascending limb.20,35,66,144,157 A study in humans with inherited defects in this protein has demonstrated significant impairment of magnesium and calcium reabsorption in the thick ascending limb with no change in sodium and chloride reabsorption.20 A similar genetic anomaly has been documented in Japanese Black cattle that develop early renal failure.72,111,142 When compared with each other, renal handling of magnesium and calcium appear to be similar in both the bovine and human conditions.112
Changes in the transepithelial voltage and paracellular permeability to magnesium strongly influence magnesium absorption from the thick ascending limb.35,66 Increases in salt movement from the lumen will concurrently elevate the transepithelial electrical potential and facilitate magnesium absorption. Numerous factors can influence both of these properties resulting in an increase or decrease in magnesium absorption. Hormones such as parathyroid hormone, calcitonin, glucagon, antidiuretic hormone, aldosterone, and insulin all act to increase magnesium absorption from the lumen.35 Conversely, prostaglandin E2, hypokalemia, hypophosphatemia, and acidosis can all act to decrease magnesium absorption.35
In addition to the above influences on paracellular absorption of magnesium, a basolateral extracellular receptor, termed the calcium/magnesium sensing receptor or cation-sensing receptor (CASR) also appears to play a crucial role.35,62,153 The CASR senses extracellular calcium and magnesium concentrations at the basolateral membrane and is coupled to intracellular inhibitory G proteins, which will inhibit and neutralize the effect of other hormonal influences mentioned above.35,37,62,153 Activation of the CASR in the loop appears to decrease salt absorption (sodium, magnesium, and calcium).37,62 To what effect, if any, the CASR may play a role in altering the permeability of PCLN-1 to magnesium transport is not known. Some researchers have suspected that there is a selective effect on paracellular permeability to magnesium that cannot adequately be explained by changes in voltage and hormonal influences, leading to speculation that the CASR may influence PCLN-1 permeability.38 The CASR is also found throughout the gut, and although its function there, related to magnesium balance, is not completely understood, it likely plays a very similar role in both organs.81
Distal Convoluted Tubule
The distal convoluted tubule (DCT) does not appear to act as a mass transporter of magnesium as the ascending loop does, but instead is the site for many complex influences to determine the final magnesium excretion (Fig. 8-3). The DCT normally reabsorbs approximately 10% to 15% of the filtered magnesium.37,153 When necessary it can be very efficient at reabsorbing magnesium, reabsorbing as much as 70% to 80% of the magnesium that is delivered from the thick ascending limb.37,153 There does not appear to be any ability to further reabsorb or secrete magnesium in nephron segments distal to the DCT, thus the final concentration of magnesium in the urine is principally determined by the DCT.
Reabsorption of magnesium in the DCT appears to occur only through active transcellular routes. Passive paracellular transport does not appear to occur to any significant degree. As a result, the absorption of magnesium via this route is an energy-requiring saturable process. The transcellular transport of magnesium is dependent on favorable transepithelial concentration and voltage gradients, similar to the gut.37 Evidence suggests that the principle entry for magnesium into the cell is through the unique transient receptor protein TRPM6.190
While there is evidence that the influx of magnesium into the cell occurs via TRPM6, there is not yet a documented mechanism of magnesium efflux from the basolateral cell membrane. Several authors speculate, based on available evidence, that a sodium/magnesium countertransporter is likely to exist.37,153,190 The presence of a sodium/magnesium countertransporter appears to exist in human red blood cells and insulin appears to be at least one of the regulating influences on its function.55
Although the precise mechanisms for magnesium entry and exit from the DCT cells remain to be completely identified and described, the influence of other factors upon magnesium transport in DCT cells has been more completely studied. Through complex intracellular signaling pathways, numerous hormones play a role in regulating magnesium transport. Parathyroid hormone, glucagon, antidiuretic hormone, insulin, aldosterone, 1,25-dihydroxycholecalciferol, prostaglandin E2, ß-adrenergic agonists, and increased sympathetic stimulation via the renal nerve have all been shown to play a role in increasing magnesium conservation.37,190 The intracellular signaling pathways that occur following activation of the receptor by any of these hormones/factors are complex and interrelated. As a result, the role of each individual factor, particularly amid the “noise” of multiple simultaneous influences, is difficult to predict. Regardless, however, the sheer number of regulatory signals being sent to this portion of the nephron underscores the importance of magnesium balance within the organism.
Like the gut and the thick ascending limb, the DCT also possesses significant concentrations of the CASR. Further study of this receptor has confirmed separate binding sites for both calcium and magnesium.37,169 Thus, the receptor may be able to sense the extracellular concentrations of both ions and send independent signals to intracellular regulators (preferentially for calcium), allowing for completely separate control of magnesium and calcium balance.169 Once activated with magnesium, however, the CASR sends a strong inhibitory signal via G proteins, which negate the positive influence of peptide and steroid hormones and vitamin D3 on magnesium transport.37 How it accomplishes this is not yet clear. Possible sites of action include the purported basolateral sodium-magnesium countertransporter or gating of the TRMP6 or TRPM7 ion channels.37
The influence of other electrolyte and acid-base abnormalities also can exert a powerful negative effect on magnesium transport in the DCT. As in the loop of Henle, hypokalemia, hypophosphatemia, and acidosis all decrease magnesium reabsorption.37 The precise mechanism of how these factors influence magnesium transport is not known, and it is likely that each has a different mechanism. Their combined effect can therefore be additive because they influence magnesium transport in different ways.
Effects of lactation
The nutrient composition of both dog and cat milk has been recently studied.1,2 Magnesium content of both cat and dog milk appears to be modestly higher during the first 2 to 3 days of lactation, or in the colostrum of both of these species, then tends to remain at a constant level throughout the remainder of the lactation period.1,2 This is in sharp contrast to calcium levels, which rise consistently throughout lactation.1,2
The contrasting presentation of postparturient hypocalcemia among species is an area that has not been completely explained and is thus an area where further study is needed. The classic tetanic presentation of post-parturient hypocalcemia in dogs is markedly different from the paretic presentation of cattle. In addition, the tetanic presentation of hypomagnesemia or “grass tetany” in cattle adds further evidence to suggest that neuromuscular transmission is affected by complex interactions between calcium and magnesium. A case report of a lactating bitch with significant hypocalcemia and hypomagnesemia that presented with paresis rather than tetany adds fuel to the speculation that the type of presentation is dependent on numerous electrolyte factors and interactions among them.11 A follow-up retrospective study of serum electrolyte concentrations in 27 bitches with eclampsia or post-parturient hypocalcemia revealed that 12 (44%) were concurrently hypomagnesemic, further suggesting that that magnesium concentrations may play a role in the pathophysiology of eclampsia in the dog and that magnesium concentrations should be assessed in bitches with eclampsia.12
Manifestations of magnesium deficiency
A large body of research is present in the scientific literature evaluating the effects of magnesium deficiency induced in animal models. Rodent models have been most commonly used, but early literature also evaluated the effects of magnesium deficient diets in young growing dogs. Several of these early canine studies showed dramatic changes that occurred within 2 weeks of introduction of the diet with increasing severity until 5 to 7 weeks when the animals were euthanized.16,25,26,117,171 Poor growth rates and numerous dermatologic and soft tissue problems, such as dry brittle hair and nails, peripheral vasodilation and swelling, and splaying of the paws, were identified in the early weeks of these trials. After 5 to 7 weeks, however, neuromuscular signs, including seizure activity, were noted and postulated to contribute to the death of these animals.117,171 In two studies, significant myocardial necrosis with associated fibrosis and calcification were also noted after euthanasia.160,178 Results of these trials assisted researchers in understanding magnesium’s physiologic role in the organism. As technology has improved, so too has our understanding of the central role magnesium plays in the maintenance of healthy organisms.
Magnesium’s importance to living organisms can be traced through the early development of life.4 Primeval oceans contained large quantities of magnesium, and the earth’s crust at the time was predominantly composed of iron-magnesium silicate. As early plants perfected photosynthesis, magnesium played a core role in energy production as a component of chlorophyll. As animal life developed, magnesium played another core role in the production of ATP. In fact, as life has developed on earth, magnesium and calcium appear to have played complementary roles to each other, with magnesium being involved in energy production and cell metabolism, and calcium’s role defined more by the essential role it plays in structural stability (bone) and movement (neuromuscular activity). Magnesium’s evolution as a “behind the scenes” ion that keeps the inner machinery of the cell running smoothly and supplied with ample amounts of energy has perhaps contributed to the long period of time in which it received little clinical attention. Recently, however, this electrolyte has been placed under greater scrutiny and it has been the focus of research activity.
Magnesium plays a pivotal role in many cellular metabolic processes. Although magnesium is the second most abundant intracellular cation, most of the intracellular magnesium is bound to numerous molecules that regulate energy production, storage, and use.6 Magnesium plays a vital role in the mitochondria during oxidative phosphorylation and during anaerobic metabolism of glucose.6 In addition, magnesium participates in a number of other important intracellular events, such as the synthesis and degradation of DNA, the binding of ribosomes to RNA, adenine nucleotide synthesis, and the production of important intracellular second messengers such as cyclic AMP.6,118 Perhaps most well known is magnesium’s function as a cofactor with ATP as the driving force behind intracellular ion pumping activity. Significant ion pumps, such as the membrane bound Na+-K+ ATPase, HCO3– ATPase, and Ca2+ ATPase, all require Mg2+-ATP to maintain effective ionic gradients within and outside the cell.6,100 As a result, magnesium has an important function in maintaining appropriate intracellular potassium concentrations and serves to regulate cytoplasmic calcium concentrations by stimulating the sequestration of calcium into the endoplasmic and sarcoplasmic reticula. The importance of magnesium’s intracellular role becomes apparent clinically in several conditions.
Cardiovascular system
Contraction of both cardiac and smooth muscle is a complex sequence of events that is orchestrated by many factors and requires rapid shifting of intracellular ions to maintain appropriate concentration gradients. Intracellular calcium, released from the sarcoplasmic reticulum or entering the cell from the extracellular space, is the initiating factor in muscle contraction. Magnesium (both intracellular ionized magnesium level and extracellular level) plays an important regulatory role in the intracellular cycling of calcium in muscle cells. It is a cofactor for the Ca2+ ATPase that rapidly shunts intracellular calcium back into the sarcoplasmic reticulum after the contraction cycle is complete. In addition, there is some evidence to suggest that extracellular magnesium may act as a calcium channel blocker for some cell membrane bound calcium channels, limiting the influx of extracellular calcium into the cytosol.77,100 Intracellular and extracellular magnesium levels thus play an important role in cardiac excitability, contraction, and conduction through their regulatory effects on calcium movement.
Cardiac conduction electrophysiology is complex and involves finely orchestrated movement of sodium and calcium ions into and potassium out of the myocytes to propagate an action potential and depolarize the cell. Rapid restoration of these electrolytes against their normal electrochemical gradients occurs to allow the cell to repolarize itself and prepare for the next action potential to occur. Magnesium has several roles in this process. First, magnesium is a cofactor for the ionic pumps that rapidly pump sodium out of the cell, potassium back into the cell, and calcium out of the cell or back into the sarcoplasmic reticulum. In addition, magnesium serves as an important gating mechanism to control the movement of intracellular calcium as described above, and it also acts to prevent the leak of potassium from inside the cell. Intracellular calcium overload triggered during myocardial ischemia by mediators such as lysophosphatidylcholine (LPC) has been implicated as an important cause of ventricular arrhythmias that result from ischemic conditions.122 Magnesium may act as an antiarrhythmic agent by limiting intracellular calcium overload in such conditions.122
Cardiac arrhythmias are clinical manifestations that can arise from derangements of intracellular or extracellular electrolyte concentrations of magnesium, potassium, and calcium.76,129 Common arrhythmias documented in humans in which magnesium deficiency has been implicated as a cause of, or contributing to the severity of, include: atrial fibrillation, supraventricular tachycardia, torsades de pointes, ventricular ectopy, ventricular tachycardias, and toxic digitalis arrhythmias.126,137,182 Some but not all of these arrhythmias may have an association with hypomagnesemia in veterinary patients, but no definitive studies have documented the prevalence of various pathophysiologic causes of arrhythmias.
Magnesium’s effect on the peripheral vasculature is also significant. Magnesium appears to control or exert a powerful role in calcium cycling in the smooth muscle of the peripheral vasculature, with higher intracellular concentrations of magnesium producing a relaxing or vasodilating effect.90,100,152 Low concentrations of intracellular magnesium appear to have the opposite or vasoconstricting effect. As a result, magnesium deficiency has been implicated as a potential contributing cause in the constellation of causes of systemic hypertension.90,152 The recent discovery of TRPM6 and TRPM7 channels in vascular smooth muscle cells further implicates the important role magnesium has to play in the complex control of vascular smooth muscle.166
Research has also shown that magnesium plays an interesting role in the production of inflammatory cytokines and reactive oxygen species that have been postulated to play an important role in many common diseases of the cardiovascular system of humans.90,152,174–177 The origin of this research interest was the cardiac necrosis and lesions in the myocytes of animal models fed magnesium deficient diets.174 The cause of these lesions and cardiac dysfunction appears to be the reactive oxygen species that originate from neuropeptide Substance P induced activation of macrophages, neutrophils, and mast cells and an increase in important up-regulating inflammatory cytokines, such as tumor necrosis factor-α and interleukin-1.174–177 A study investigating a potential link between feline cardiomyopathy and magnesium status has been reported.59 It was not successful in showing any link between magnesium and feline hypertrophic cardiomyopathy, but included only a small number of cats and did not robustly evaluate magnesium status. Animal models evaluating cardiac effects of magnesium deficiency have also shown an increased susceptibility to ischemic and reperfusion injury, indicating that magnesium also has a protective antioxidant effect.100,176
The effect of magnesium on the electrocardiograms of dogs fed a magnesium deficient diet has been reported several times with very conflicting results.115,160,178 One study reported a concurrently developing hypokalemia with an increase in peaked T waves and slight depression of the ST segment in addition to various arrhythmias.115 A second study reported a decrease in the PQ and QRS distances and an increased incidence of negative T waves but did not evaluate concurrent electrolyte disturbances.160 A third study reported yet another set of findings that included an increased incidence of mild hypocalcemia, but normokalemia and transient RST segment and T wave changes, which were not consistent or frequent enough to allow the authors to make any definitive conclusions about electrocardiographic changes associated with hypomagnesemia.178
Neuromuscular system
In small animal patients, neuromuscular signs of hypomagnesemia are rare. Perhaps the most instructive example of acute central nervous system magnesium deficiency is grass tetany or grass staggers of cattle. In this condition, increased neuronal hyperexcitability and neuromuscular transmission occurs, causing severe muscle tetany and seizure activity that frequently results in death. Chronic forms of magnesium deficit in humans have also been implicated in any number of neurologic and neuromuscular conditions, including migraine headache, sudden infant death syndrome, age-related dementias, chronic fatigue syndrome, and many other psychiatric and sleep-related disorders.46–48,50,52,53 An acute neurologic condition similar to grass tetany and suspected to have been caused by magnesium deficiency has also been described in a high school football team.89 The pathophysiology of the acute and chronic clinical forms of magnesium deficit are likely to be multifactorial, but several contributing causes have been postulated. A decrease in neuronal magnesium concentration is believed to increase the likelihood of calcium binding to prejunctional acetylcholine vesicles increasing release of acetylcholine into the neuromuscular cleft and increasing the likelihood of muscle contractions.63 In addition, magnesium has been shown to block N-methyl-D-aspartate (NMDA) receptors within the central nervous system. NMDA receptors are involved in numerous central nervous system functions, including pain sensation and excitatory neurotransmitter activities.44 Some researchers have also speculated that NMDA receptor blockade by magnesium may play a role in bronchial smooth muscle relaxation.143 Other causes that have been identified as potential contributing factors to neuromuscular effects of magnesium deficit include increased excitatory neurotransmitter release, decreased inhibitory neurotransmitter release, production of inflammatory neuropeptides (Substance P), antioxidant reserves, and the important influence of magnesium on numerous intracellular second messenger systems.46,48
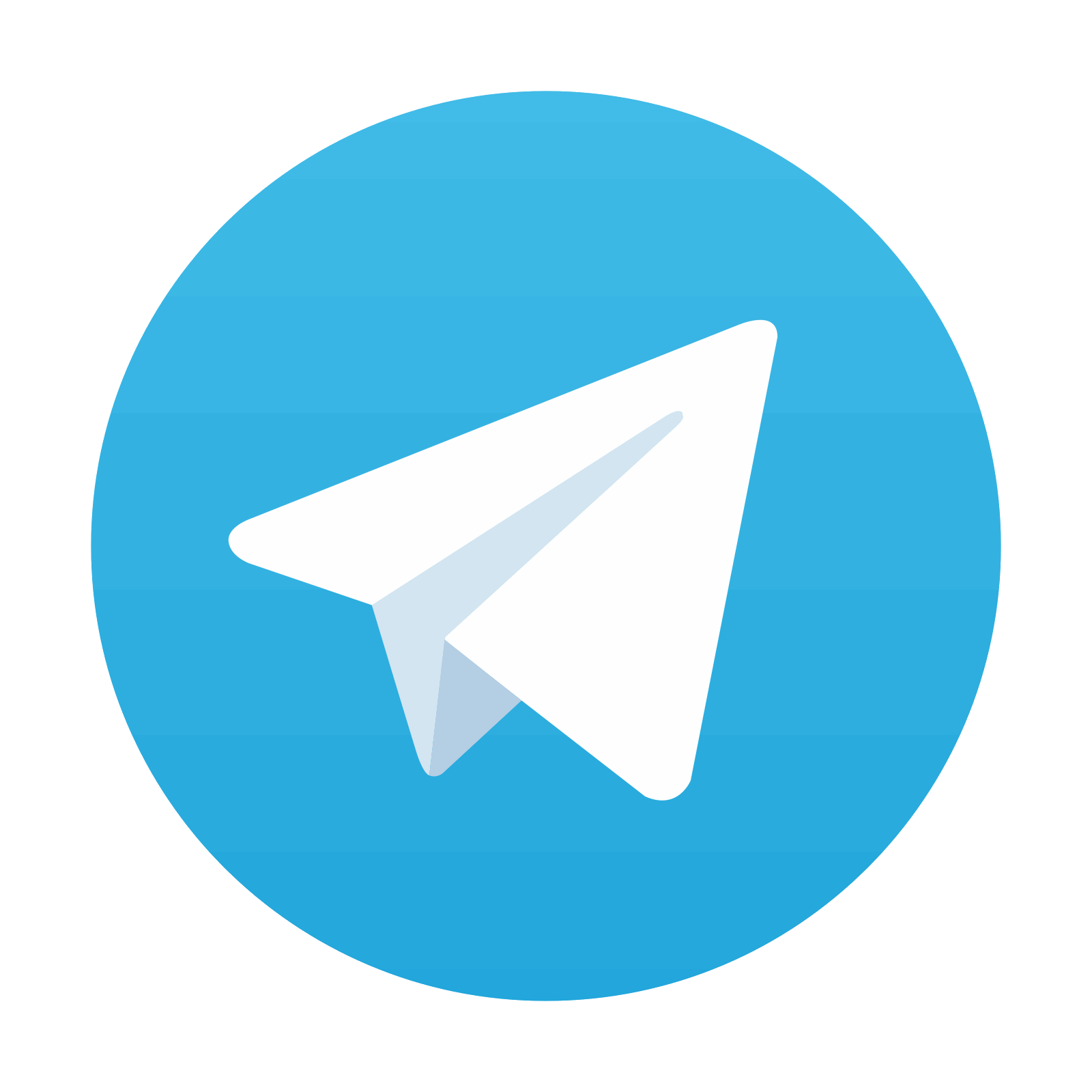
Stay updated, free articles. Join our Telegram channel

Full access? Get Clinical Tree
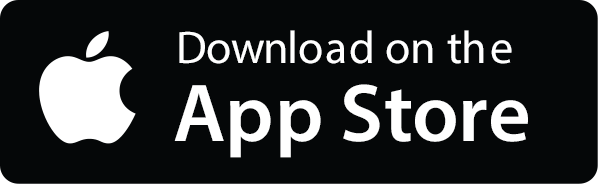
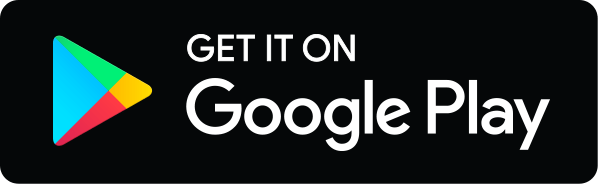