Table 5-7 | Number of Presumed Pathogenic, Gram-Negative Bacterial Isolates Identified in Horses With Ulcerative Bacterial Keratitis
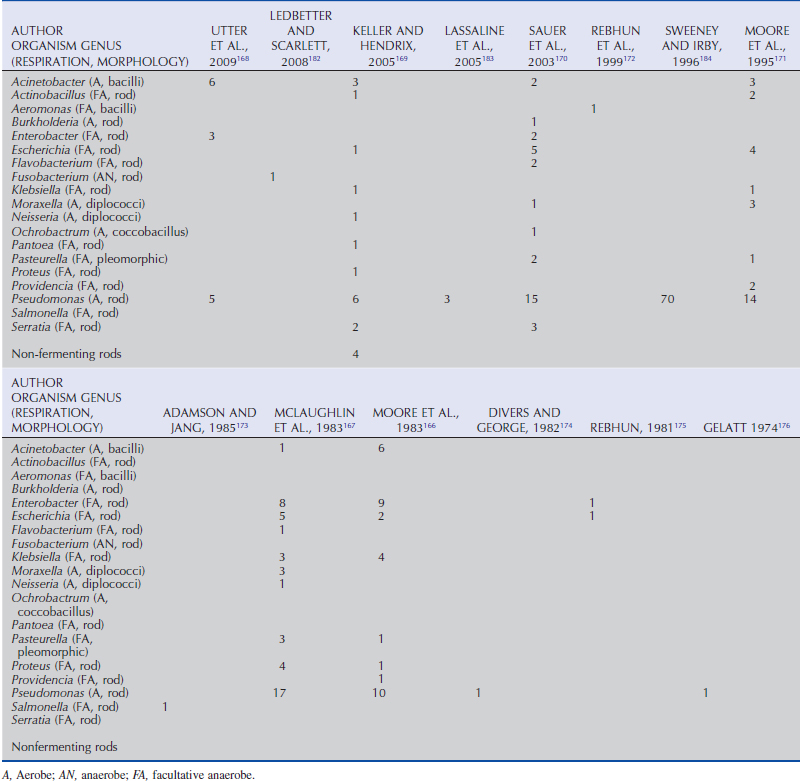
Chapter 5 Diseases and Surgery of the Cornea
Clinical Anatomy and Physiology
Anatomy
Grossly, the normal equine cornea measures 29.7 to 34.0 mm horizontally and 23.0 to 26.5 mm vertically in an adult,1,2 and 20.5 to 26.6 mm horizontally and 19.5 to 24.0 mm vertically in younger horses (Fig. 5-1).2,3 In Miniature horses, mean horizontal and vertical diameters measure 25.8 mm and 19.4 mm, respectively, and increase with age up to 7 and 5 years, respectively.4 Corneal diameter also increases with age in normal Rocky Mountain horses.2 Compared to other mammals, the equine cornea is relatively flat, with a mean corneal curvature in Rocky Mountain horses measured at 20.59 ±1.72D,2 versus a curvature of 16.46 ± 1.5D calculated for normal adult horses of other breeds.5 The large change in refractive index at the air/cornea interface makes the cornea the major refractive structure of the eye. In vivo corneal thickness measurements in normal horses, obtained via ultrasonic pachymetry, are greater peripherally than centrally, with the central cornea measuring 770 µm2 and 793 µm.6 Following enucleation, the mean central corneal thickness of normal horses measured 893 µm, being significantly thicker dorsally and ventrally relative to centrally, medially, and laterally.7 Mean central corneal thickness in normal Miniature horses was 785.6 µm.4 In healthy Rocky Mountain horses, the corneal thickness increases with age,2 whereas no variation in corneal thickness has been noted with age, gender, or endothelial cell density in Miniature horses or horses of other breeds.4,7
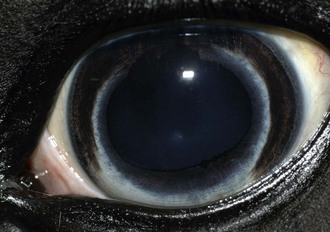
Figure 5-1 Clinical photograph of a normal equine eye, demonstrating the ocular clarity of the cornea.
Histologically, the cornea comprises three primary layers of alternating lipophilicity: the superficial epithelium (lipophilic), central stroma (hydrophilic), and deep endothelium (lipophilic) (Fig. 5-2). The stratified squamous epithelium, consisting of 8 to 12 layers of nonkeratinized squamous cells, wing cells, and basal cells, is anchored to its basement membrane by hemidesmosomes. Anchoring fibrils attached to the hemidesmosomes penetrate the basement membrane to end in anchoring plaques in the anterior stroma, creating a stable epithelium/basement membrane/anterior stromal adhesion complex.8 The poorly cellular stroma, which is 90% of the corneal thickness, is composed of water (75% to 80%), regularly arranged collagen fibers, and a proteoglycan matrix with the glycosaminoglycans (GAGs) dermatan sulfate, chondroitin sulfate, and keratan sulfate.8 Arrangement of GAGs within the cornea varies by region and depth, with greater levels of chondroitin-4 sulfate in the deep cornea relative to the superficial regions, which have a higher proportion of chondroitin-6 sulfate.9 This variation may impact corneal water retention and wound healing, ultimately affecting corneal clarity.9 Deep to the stroma is Descemet’s membrane, the basement membrane of the innermost endothelial layer. Due to continued secretion throughout life, Descemet’s membrane is thicker in older individuals.8 The endothelium is a single layer of closely interdigitating hexagonal cells that forms a physical barrier between the cornea and aqueous humor and also utilizes an active pumping mechanism to keep water and solutes out of the cornea (Fig. 5-3).8 The efficiency of the barrier and pump functions is determined by endothelial cell size, shape, and density, as well as the presence of certain cofactors (i.e., calcium, glutathione, bicarbonate) within the aqueous humor.8 Mean endothelial cell density in normal horses is 3155 cells/mm2, showing no variation with gender but declining with increasing age.7 Interestingly, no correlation was noted between endothelial cell density and corneal thickness in this study, seemingly counter to the effect of endothelial cell density on barrier and pump functions.7 In this study, however, the measured thickness included Descemet’s membranes of variable thicknesses and did not include any corneas with endothelial cell loss below the critical value of 400 to 700 cells/mm2 necessary for effective endothelial function.7,8
Physiology
Nutrition
Oxygen is supplied to the cornea by the external atmosphere and precorneal tear film, with lesser amounts provided by the aqueous humor and limbal blood vessels.8 When the eyelids are closed, the palpebral conjunctiva provides smaller amounts of oxygen.8 Glucose, amino acids, and vitamins are predominantly obtained from the aqueous humor, with smaller amounts from the precorneal tear film and limbal blood vessels.8 Additional glucose may be obtained from subepithelial glycogen stores in times of increased demand such as wound healing, either via aerobic or anaerobic (glycolysis) metabolism.8
Microflora
The normal corneal and conjunctival surface microflora are primarily gram-positive bacteria and fungal organisms, but gram-negative organisms are present as well (Tables 5-1 to 5-3). Consistent with previous reports,10–12 recent evaluations of horses in the United States identified Staphylococcus spp. (22% to 50%),13,14 Bacillus spp. (17% to 32%),13,14 and Corynebacterium spp. (29%),13 as common isolates, in addition to a possible increasing prevalence of Streptomyces spp. (66%).14 The most common gram-negative isolates from horses in the United States are Moraxella spp. (28%), Escherichia spp. (24%), Acinetobacter spp. (18%), and Enterobacter spp. (14%).14 Fungal organisms isolated from normal horses in the greatest frequency include Aspergillus spp., Cladosporium spp., Alternaria spp., and Penicillium spp.12,14–16
Table 5-1 | Gram-Positive Bacterial Isolates from Conjunctival/Corneal Samples from Healthy Horses (2003-2005)
AUTHOR COUNTRY (STATE) ORGANISM % (NUMBER/TOTAL) | ANDREW ET AL.13 US (FL) | GEMENSKY-METZLER ET AL.14 US (OH) |
---|---|---|
Actinomyces | 6 (135/137) | |
Bacillus | 17 (398/2357) | 32 |
Corynebacterium | 29 (689/2357) | 4 |
Dermatophilus | <1 (3/2357) | |
Diphtheroid | 10 | |
Enterococcus | 2 | |
Lactobacillus | <1 (1/2357) | |
Micrococcus | 2 (53/2357) | 8 |
Rhodococcus | <1 (5/2357) | |
Staphylococcus | 22 (513/2357) | 52 |
Streptococcus | 4 (87/2357) | 36 |
Streptomyces | <1 (19/2357) | 66 |
Unidentified | <1 (6/2357) |
Table 5-2 | Gram-Negative Bacterial Isolates from Conjunctival/Corneal Samples from Healthy Horses (2003-2005)
AUTHOR COUNTRY (STATE) ORGANISM % (NUMBER/TOTAL) | ANDREW ET AL.13 US (FL) | GEMENSKY-METZLER ET AL.14 US (OH) |
---|---|---|
Achromobacter | ||
Acinetobacter | 18 | |
Actinobacillus | 2 | |
Alcaligenes | <1 (2/2357) | |
Citrobacter | 8 | |
Enterobacter | 14 | |
Escherichia | <1 (1/2357) | 24 |
Klebsiella | <1 (5/2357) | |
Moraxella | 10 (225/2357) | 28 |
Neisseria | ||
Pasteurella | <1 (4/2357) | |
Proteus | <1 (1/2357) | |
Pseudomonas | <1 (1/2357) | 8 |
Sphingomonas | <1 (1/2357) | |
Stenotrophomonas | <1 (2/2357) | |
Unidentified |
Innervation
The highly sensitive cornea receives much of its innervation via the ophthalmic branch of cranial nerve (CN) V (trigeminal nerve). As determined in other species, ciliary nerve branches enter the limbus at the level of the midstroma and course toward the superficial cornea, where they form anterior stromal and subepithelial plexi.8 Termination of the nerve fibers occurs in the superficial layers of the epithelium,17 so deeper corneal layers are comparatively poorly innervated.8 In normal adult horses, corneal sensitivity, quantified using the corneal touch threshold measured by Cochet-Bonnet esthesiometry, is greatest in the central, ventral, and lateral quadrants relative to the nasal and dorsal quadrants.18,19 In sick foals, corneal sensitivity is significantly decreased in all quadrants relative to normal foals and adults.18 Pharmacologic corneal anesthesia is important in the diagnosis and treatment of surface ocular disease. Evaluation of the duration of effect of topical ocular proparacaine (0.5%) in normal horses indicated that a single 0.2-mL instillation significantly decreased corneal sensitivity within 5 minutes, with peak effect lasting 25 minutes.20
The extensive corneal innervation serves two main functions: protection of the eye and stimulation of wound healing. Activation of nociceptive fibers by mechanical, thermal, and chemical stimuli is extremely important to corneal and overall ocular protection, while neuropeptides such as substance P and calcitonin gene-related peptide may exert a trophic influence, stimulating epithelial healing.21 The importance of these functions may be particularly evident in animals with decreased corneal innervation, potentially predisposing them to development of ulcers (i.e., neurotrophic ulcers) and delaying appropriate wound healing.
Corneal Immunology
The cornea is an immunologically privileged tissue, anatomically contiguous with two immunologically privileged sites, the ocular surface and the anterior chamber. Immune privilege (IP) is an evolutionary adaptation that downregulates the immune-driven ocular inflammatory response to protect vulnerable structures and preserve vision. It is counterbalanced by the threat of unchecked infection due to inadequate immunoresponsiveness. Corneal IP is governed primarily by normal endothelial cell function,22 in addition to the absence of blood and lymphatic vessels, the expression of complement regulatory proteins in the aqueous humor and on corneal cells, the expression of Fas ligand on corneal cells, and the relative paucity of professional antigen-presenting cells (APC) constitutively expressing MHC class II antigen (e.g., dendritic cells and Langerhans cells).23–25 Proinflammatory cytokines, in particular interleukin 1 (IL-1) and tumor necrosis factor α (TNF-α), are responsible for activation and recruitment of these APCs into the cornea and inducing expression of MHC II antigen on native corneal cells, ultimately resulting in loss of corneal IP. Loss of corneal IP, coupled with corneal lymphangiogenesis in the presence of inflammation, permits lymphocyte trafficking into afferent lymphatics,26,27 creating an effectively “normal” adaptive immune response in inflamed or injured corneas. Corneal allograft rejection and exacerbation of herpetic keratitis in humans may be explained in part by this immunopathogenesis. For more information on ocular surface immunology, please see a recent review article in Veterinary Clinics of North America.28
Wound Healing
Epithelium
The epithelium prevents microbial invasion of the cornea and limits fluid uptake by the hydrophilic stroma.8 Superficial epithelial cells lost in a constant cycle of apoptotic shedding are replaced by anterior migration of cells generated by the mitotically active basal layer, which is in turn replaced by centripetal migration of limbal stem cells.8 With a basal mitotic rate of 10% to 15% per day, the entire epithelium turns over every 7 days.29
When epithelial cells are lost through corneal abrasion or ulceration, without damage to the underlying basement membrane, basal epithelial cell mitosis ceases, cells lining the wound edge retract and thicken, and epithelial cell/basement membrane hemidesmosomal attachments are disrupted (Fig. 5-4).8 Centripetal amoeboid migration of surrounding basal cells along a protein scaffolding overlying the basement membrane ultimately (i.e., within hours of injury) results in coverage of the wound bed, at which time basal cell mitosis resumes, restoring the epithelium to its normal structure.30 In contrast to wounds limited to the epithelial cell layers, those in which the basement membrane is damaged reepithelialize relatively rapidly; however, complete healing may take months owing to necessary reformation of basement membrane and associated adhesion complexes.31 In horses, experimentally induced corneal ulcers in which the epithelium, basement membrane, and anterior third of the stroma were surgically removed had a median time to reepithelialization of 11 days, or a rate of 0.6 mm/day.32
Stroma
Healing of corneal stromal wounds is significantly more complex, beginning with infiltration of polymorphonuclear cells at the wound edge within hours of injury, followed soon after by monocytes (see Fig. 5-4).8 Surrounding stromal keratocytes undergo fibroblastic transformation, proliferate, and begin to synthesize collagen and extracellular matrix components such as proteoglycans. Fibronectin is also produced following wounding, stimulating cell adhesion and migration, protein synthesis, and fibroblast migration, ultimately promoting wound healing.33 Remodeling of the stroma by reformation of cell processes and gap junctions and reorganization of collagen fibrils is initially associated with decreased corneal transparency, but continued remodeling over months to years restores normal tensile strength and variable degrees of transparency.33
Endothelium
In contrast to the remarkable healing and regenerative capacities of the corneal epithelium and stroma in response to injury, that of the endothelium is much more limited. Loss of endothelial cells due to injury/disease, surgical trauma, or aging, leads to replacement by enlargement and migration of adjacent endothelial cells rather than replication. Overall cell number is reduced, leading to abnormally large (polymegathism) and abnormally shaped (pleomorphism) endothelial cells. Tight junctions are maintained among cells during this migration, and following wound closure, sodium/potassium–adenosine triphosphate (Na+/K+-ATPase) pump sites are increased, maintaining the effective barrier and pump functions of the endothelium,34 provided the cell density is greater than the critical density of 400 to 700 cells/mm2.35
Growth Factors
As in other tissues, healing of corneal wounds is enhanced by peptide growth factors, which, following local and systemic production, reach the avascular cornea via the aqueous humor, tears, and limbal blood vessels. Epidermal growth factor (EGF), platelet-derived growth factor (PDGF), transforming growth factor β (TGF-β), connective tissue growth factor (CTGF), keratinocyte growth factor (KGF), insulin-like growth factor (IGF), and hepatocyte growth factor (HGF) perform varied functions, such as promotion or inhibition of corneal epithelial cell proliferation or migration, keratocyte proliferation, and inflammatory cell influx (Table 5-4).36 The complex and incompletely understood interactions and potential species differences among growth factors make definitive statements about their functions difficult. In other species, EGF has been documented to increase normal cell turnover, promote vascularization, and stimulate healing of epithelial and endothelial wounds.37 PDGF enhances tissue repair by influencing inflammatory reactions, vascularization, proliferation, chemotaxis, and extracellular matrix production.38 TGF-β increases collagen synthesis, possibly due to increasing production of CTGF, which stimulates fibroblast and collagen production39; however, it also inhibits epithelial cell proliferation and keratocyte migration,36 producing varied effects on overall corneal wound healing.
Evaluation of growth factors in horses has been more limited. Using cultured equine epithelial cells and keratocytes, increased proliferation of epithelial cells and keratocytes in response to EGF and PDGF was noted, while TGF-β decreased proliferation of both.40 Use of high-dose (50 mcg/mL) and low-dose (5 mcg/mL) EGF in horses with experimentally induced corneal ulcers identified a trend toward more rapid healing in the high-dose group. However, both doses of EGF were associated with increased corneal edema, vascularization, melanosis, and scarring, side effects which likely negate any potential benefit of administration of EGF as performed in that study.41 Additionally, CTGF has been identified in the tear fluid of both normal and ulcerated equine eyes, with lower levels in ulcerated eyes postulated to be associated with exhausted production of CTGF by lacrimal and third eyelid glands, and/or increased utilization of CTGF by ocular surface receptors.42
Opioid growth factor (OGF) works in a receptor-mediated fashion in normal corneal homeostasis as a tonic inhibitor of cell division.43 Experimental studies in rats, rabbits, and human corneal cell cultures have identified an increased rate of reepithelialization following administration of or incubation with the strong OGF inhibitor, naltrexone.44–46 OGF and its receptor have been immunohistochemically localized to the equine corneal epithelium,47 but its specific role in the equine cornea related to epithelial wound healing has not been investigated.
Proteases
Both maintenance of normal corneal health and repair during disease or wound healing depend upon proteolytic enzymes and their inhibitors for proper tissue turnover.48–52 In normal corneas, enzyme inhibition balances degradative activity to promote corneal homeostasis. Disease may result in excessive proteolytic activity by enzymes derived from the precorneal tear film, corneal epithelial cells, keratocytes, infiltrating inflammatory cells, and infectious organisms.49,50,53–57 This excess proteolytic activity manifests as corneal stromal “melting,” or keratomalacia.
The two most extensively studied groups of corneal proteinases are the matrix metalloproteinases (MMPs) and the serine proteases, specifically neutrophil elastase (NE).48 NE, produced by leukocytes, digests collagen types III and IV, laminin, fibronectin, and heparan sulfate53,58–60 and has been identified in significantly higher levels in the preocular tear film of horses with ulcerative keratitis than in normal controls.53 MMP-2 and MMP-9 are both gelatinases but appear to play different roles in corneal physiology. Using immunohistochemistry, MMP-2 was found to be constitutively present in healthy equine cornea, primarily in the epithelium and to lesser degrees in the stroma and endothelium, while MMP-9 was not identified in healthy equine cornea.48 In diseased equine cornea, MMP-2 expression was increased throughout the equine cornea, with concurrent MMP-9 expression also throughout.48 The use of gelatin zymography to identify proteolytic activity of MMP-2 and -9 documented an increase in total tear film proteolytic activity in ulcerated equine eyes relative to the contralateral healthy eye, with a decrease in activity to that of the contralateral healthy eye by the day of ulcer healing.61 These findings support the role of MMP-2 in corneal homeostasis, becoming locally activated in times of minor wound repair,57,62 whereas MMP-9 is expressed by epithelial cells and PMNs following more extensive corneal wounding.62–64 Control of this proteolytic activity therefore constitutes a significant component of therapy in such conditions.
Sequelae to Corneal Wounding
Vascularization
The avascularity of normal cornea is important for maintaining optical clarity; however, many disease processes promote corneal vascularization in an effort to augment healing (Fig. 5-5). Inflammatory cells incited by the initial injury and inflammatory mediators from the cyclooxygenase and lipoxygenase pathways stimulate angiogenesis from the perilimbal blood vessels.8,65,66 Depending upon the disease process, and potentially the species involved, growth factors implicated in stimulating angiogenesis include basic fibroblast growth factor (bFGF),67 vascular endothelial growth factor (VEGF),67 and TGF-β,68 which may exert an inhibitory effect.69 The interactions among various cytokines, growth factors, and other components of the inflammatory process have yet to be fully elucidated.
Pigmentation
Chronic ocular surface irritation induces pigment migration onto the corneal surface from limbal and perilimbal tissues (Fig. 5-6).70 Histologically, migrating superficial pigment is located within the basal epithelial cells. Stromal pigment is associated with deeper wounds involving inflammatory cells and granulation tissue. Endothelial pigment may come from uveal tissue in anterior segment dysgenesis, persistent pupillary membranes, ruptured iris cysts, anterior synechiae, chronic uveitis, or uveal melanoma.70 Pigmentation may not progress once the inciting cause is eliminated, but it is also unlikely to regress.
Edema
In the healthy cornea, stromal water content is maintained at a stable 78% primarily by the endothelium, with contributions from the epithelium and stromal proteoglycans.8 The lipophilic epithelium limits fluid uptake from the precorneal tear film, while sulfation, diameter, and spacing of stromal proteoglycans determine the water retention and transparency properties of the stroma.8 Far more important to the maintenance of stable corneal hydration, and therefore transparency, are the barrier and pump functions of the endothelium. Active endothelial pumping mechanisms for ions, amino acids, and sugars create osmotic gradients which ultimately lead to movement of 6 to 8 mL/hr of water from the stroma to the AH.8
In a cornea with healthy epithelium, stroma, and endothelium, corneal thickness (as determined by corneal hydration) is constant up to an intraocular pressure (IOP) of 50 mm Hg, after which point edema and increased thickness result.71 When corneal thickness increases due to fluid imbibition associated with underlying epithelial or endothelial dysfunction, smaller increases in IOP lead to significantly greater increases in corneal fluid uptake. For this reason, a slightly elevated IOP in the presence of preexisting mild corneal edema may lead to an apparently disproportionately greater increase in corneal thickness. The clinical manifestations of this increased stromal hydration include loss of corneal transparency (Figs. 5-7 and 5-8) and decreased visual function, as well as bullous keratopathy with predisposition to corneal ulceration (Fig. 5-9).
Congenital Diseases
Megalocornea/Corneal Globosa
Prevalence
Megalocornea, an abnormally large cornea present at birth, appears most commonly in Rocky Mountain horses as a component of a syndrome of multiple congenital ocular anomalies (MCOA) in which temporal iridal, ciliary, and retinal cysts, iridal hypoplasia, cataracts, and retinal dysplasia may also be present.72 A previous study identified 43 of 71 horses with multiple ocular anomalies as affected with megalocornea, all bilaterally73; a separate report describes two unilaterally affected foals.74 A recent study measuring corneal size and thickness suggests that the corneal appearance may in fact be corneal globosa, or an abnormally shaped cornea, rather than true enlargement.73 Horses with the Silver (also known as Chocolate) coat color are more frequently affected.72,73,75
Clinical Appearance
Affected horses have a clear cornea with normal central topography, grossly observable increased optical corneal diameter, short radius of corneal curvature, and a globular, protruding corneal contour (Fig. 5-10).73 The anterior chamber in these horses is excessively deep, while the lens-iris diaphragm appears normal (Fig. 5-11). Additionally, affected individuals may have macropalpebral fissure, miosis, congenital cataract, uveal or peripheral retinal cysts, and retinal anomalies (dysplasia, detachment).
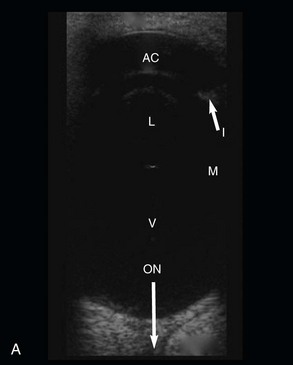
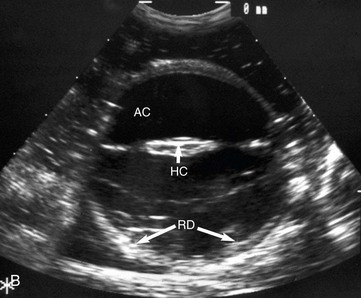
Figure 5-11 A, B-mode ultrasonographic image of a normal equine eye, showing appropriate proportions for anterior chamber length (AC), lens size (L), and vitreal chamber length (VC) (7.5-MHz probe). B, B-mode ultrasonography of one eye of the foal in Fig. 5-12. In comparison with Fig. 5-11, A, note the deep anterior chamber (AC), hypermature cataract (HC), and retinal detachment (RD).
(A, Photograph courtesy Dr. Marc Cronau.)
Pathogenesis
Megalocornea in association with multiple anterior segment anomalies is an embryologic defect, the development of which is poorly understood. In humans, optic cup growth may be decreased, resulting in successive waves of migration of mesenchymal tissue over an excessive large, broad ciliary region, ultimately producing an enlarged corneal diameter.76 This abnormal growth may originate with a neuroectodermal abnormality within the anterior optic cup.76,77 Pedigree analysis of Rocky and Kentucky Mountain horses suggests either a dominant mode of inheritance with incomplete penetrance and linkage to coat color,72 or a codominant mode of inheritance involving the MCOA locus on the ECA6q gene.75 Further, it is suggested that individuals with more severe clinical manifestations are homozygous for the mutation involving the ECA6q gene, while those with less severe signs are heterozygous.75
Microcornea
Prevalence
Microcornea, or congenitally small cornea, is infrequently reported in horses; however, severely affected individuals with corneal diameters of less than 10 mm and marked visual impairment have been described.78 Microphthalmos or other ocular anomalies may also be present.79 It is not uncommon for horses to have the appearance of an undersized cornea with an otherwise normal globe, without visual impairment.79
Clinical Appearance
In individuals with normally sized globes and palpebral fissures, microcornea allows increased visualization of the sclera. When accompanied by microphthalmos and micropalpebral fissure, a proportionally normal amount of sclera is visible.80 Cataracts and coloboma of the iris, lens, or optic nerve have also been reported in horses with microcornea.79,80
Pathogenesis
An early developmental deficiency in the optic vesicle may result in microcornea with microphthalmos, whereas a later failure of normal growth and expansion of the optic cup with premature or delayed optic vesicle/ectoderm contact may produce microcornea.80
Dermoids
Prevalence
Corneal dermoids, or choristomas, are nonprogressive, unilateral or bilateral, congenital lesions consisting of epithelial and dermis-like components found in an abnormal location.81 They have been reported in a group of related Quarter Horses in association with iridal hypoplasia and cataracts,82 and in a Standardbred colt with no other ocular anomalies.83
Clinical Appearance
As a congenital defect, corneal dermoids are generally noticed shortly after birth. They frequently arise at the dorsal limbus82 and are variably pigmented and potentially haired corneal masses (Fig. 5-12). Irritation may be caused if large lesions impair eyelid closure or if hairs from the lesion cause trichiasis.
Differential Diagnoses
Variation in the presence of pigment and hairs, the overall appearance of a dermoid, and the age at which the lesion is noticed affect possible differential diagnoses. Other types of neoplasia, reactive lymphoid hyperplasia, nodular episcleritis, and parasitic granulomas should be considered. Definitive diagnosis is based on clinical appearance and histopathologic evaluation, which identifies keratinized stratified squamous epithelium (versus the nonkeratinized epithelium of the cornea) overlying an irregular dermis with hair follicles, sweat glands, and sebaceous glands (Fig. 5-13).84 At the axial margin, collagen within the dermis blends with that of the corneal stroma, and the epidermis becomes contiguous with the corneal epithelium. The presence of cartilage or bone within a dermoid is rare.
Pathogenesis
Although the pathogenesis is unknown, abnormal differentiation of a group of cells early in development may be involved. Alternately, defective induction of surface ectoderm by the optic vesicle, producing skin rather than corneal epithelium, may be involved.85
Treatment
Most corneal dermoids are superficial, and removal by keratectomy provides complete resolution. Postoperative care is as for an uncomplicated superficial ulcer (Table 5-5), including prophylactic topical antibiotic administration until the keratectomy site has reepithelialized, and topical atropine and systemic analgesics (nonsteroidal antiinflammatory drugs [NSAIDs]) if reflex anterior uveitis is present. Dermoids may extend into the stroma, so thorough evaluation with magnification and surgical removal with appropriate instrumentation and magnification is necessary for safe removal.
Corneal Vascularization
Prevalence
Congenital corneal vascularization has been reported in a Thoroughbred foal examined at 16 hours of age86 and in a foal associated with congenital corneal melanosis.87 Temporary partial corneal vascularization and cloudiness that resolved spontaneously was reported in a group of foals.88
Clinical Appearance
Clinical signs reported in previous cases of congenital corneal vascularization include bulbar and palpebral conjunctivitis, hyperemia, and vascular congestion.86 Short, straight, superficial perilimbal vessels extended from the conjunctiva onto the dorsal cornea.86 No other ocular abnormalities or signs of ocular pain were present.
Pathogenesis
In the reported cases, pathogenesis is unknown but believed to be associated with late-term intrauterine influences (such as trauma or inflammation). Late in gestation, the eyelids are no longer attached to each other, allowing exposure of the corneas and conjunctiva to amniotic fluid and any irritants within it.86
Acquired Diseases
Ulcerative Keratitis: Noninfectious
Superficial Corneal Ulceration
Clinical Appearance
Owing to the extensive sensory innervation of the superficial cornea, horses with ulcers are generally in significant discomfort. Blepharospasm, blepharoedema, epiphora, and conjunctival hyperemia are common. Mild edema may be present in the involved area only, and fluorescein dye uptake will be clearly demarcated (Fig. 5-14). No cellular infiltrate, visible as yellowish opacification of the lesion, is present in an uncomplicated ulcer. As an isolated process, superficial ulceration should not be accompanied by vascularization of the cornea; however, mild secondary anterior uveitis manifesting as miosis, mild aqueous flare, and/or hypotony may be present.
Pathogenesis
Corneal ulcerations generally occur secondary to trauma, either exogenous (i.e., sticks, fences, hay, foreign bodies) or endogenous (i.e., entropion, trichiasis). Provided the inciting cause is eliminated and infection is avoided, simple loss of the corneal epithelium should heal within 5 to 7 days. As detailed previously, healing occurs through a process of cessation of basal cell mitosis, migration of a sheet of cells to cover the wound bed, and restoration of mitotic activity to regenerate normal epithelial architecture.8 In humans, this process is completed at a rate of 60 to 80 µm/hr, corresponding to healing a 6-mm defect within 48 hours.8 Horses reepithelialize at a rate of 0.6 mm/day.32 If the basement membrane is damaged, complete healing may be prolonged as reestablishment of basement membrane and associated adhesion complexes must occur.
Treatment
The primary components of therapy for an uncomplicated superficial ulcer are topical antibiotic therapy as prophylaxis for infection, a therapeutic mydriatic-cycloplegic to treat the secondary anterior uveitis that occurs with corneal ulceration, and a systemic NSAID for the uveitis and analgesia (see Table 5-5). An appropriate topical ophthalmic antibiotic should be broad-spectrum and minimally epithelial-toxic, such as neomycin/polymyxin B/bacitracin, erythromycin, or oxytetracycline ointments. Tetracyclines have anti-MMP activity, which may help stabilize the protein matrix involved in healing an epithelial wound, and therefore may have added benefit in treatment of superficial ulcerations beyond their antibacterial effect.89,90 Topical antibiotics are administered ideally every 6 to 8 hours, while the therapeutic mydriatic-cycloplegic is generally needed once every 24 to 48 hours.
Nonhealing Corneal Ulceration
Prevalence
Nonhealing corneal ulcers are refractory to healing in the absence of a persistent underlying cause or infection.91–93 Their prevalence is unknown, but numerous reports of such ulcers exist in veterinary literature.91–96 The reported mean ages have been 12 years in a group of 10 horses95 and 13.7 years in a group of 23 horses,93 although affected horses have ranged from 2 to 38 years.91,94,96 No gender or breed predilection has been identified, with breeds affected including Thoroughbreds, Quarter Horses, Arabians, Warmbloods, Appaloosas, and others.91,93,95,96
Clinical Appearance
Nonhealing ulcers are chronic, with mean duration prior to referral reported from 17 days93 to 54 days.95 Variable signs of discomfort are present, independent of duration. Involvement is limited to the corneal epithelium, and neither ongoing mechanical trauma nor evidence of infection is present. Nonadherent epithelial edges, identified by leakage of fluorescein underneath the epithelium surrounding a well-defined area of ulceration, are a consistent finding (Fig. 5-15).93,95
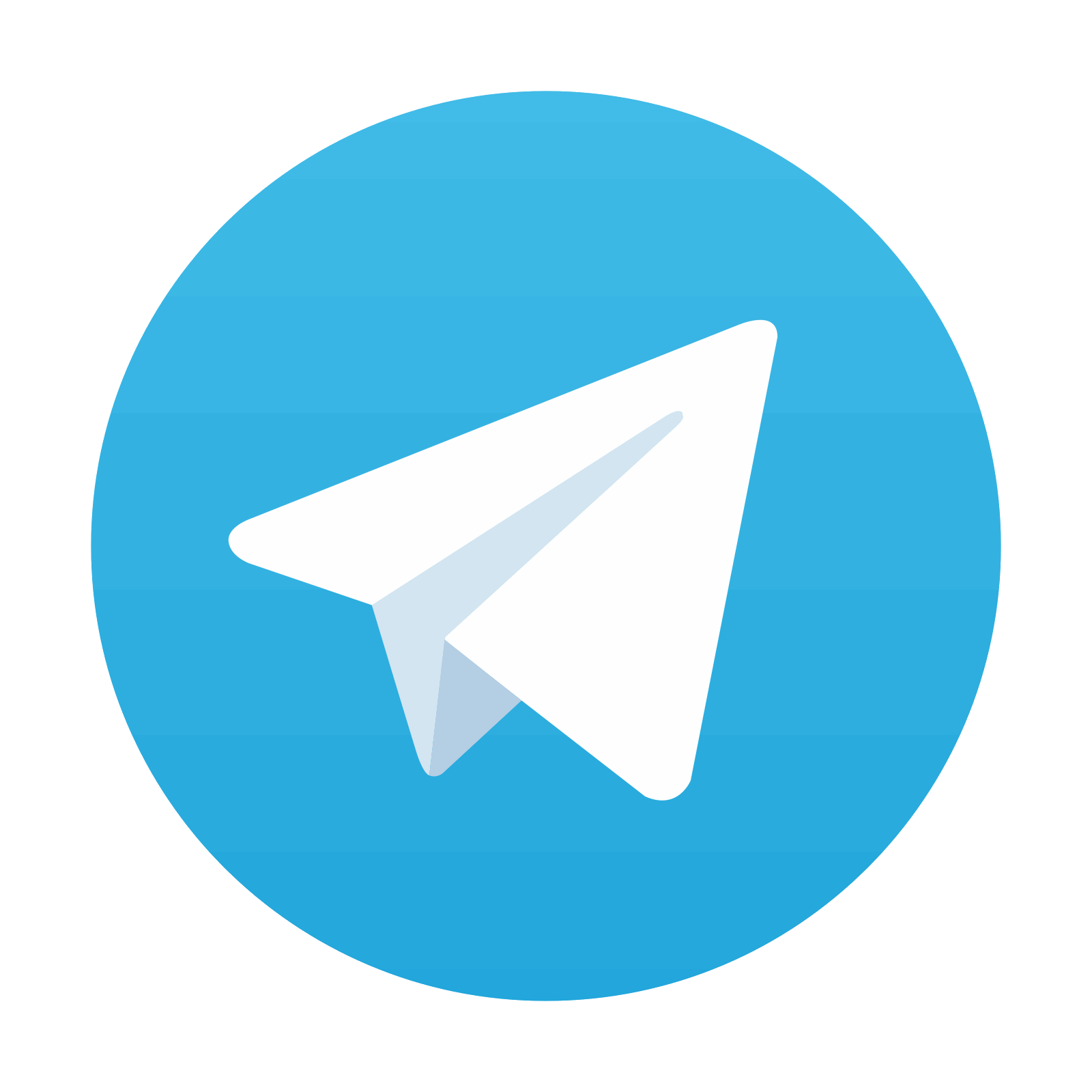
Stay updated, free articles. Join our Telegram channel

Full access? Get Clinical Tree
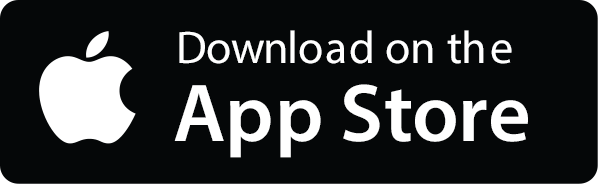
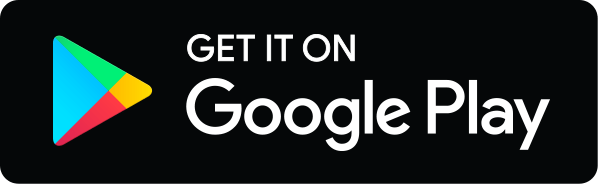
