Chapter 2 Development and Congenital Abnormalities*
DEVELOPMENT
Formation of Optic Primordia
Broadly speaking, the embryonic and fetal development of the eye occurs in three stages:
The optic primordia (rudimentary eye) develops from that portion of the embryo that later forms the anterior part of the central nervous system (CNS). The first step in the embryogenesis of the future eye takes place at the embryonic plate stage, when the ectoderm invaginates along the posterior-anterior axis to form the neural groove. The two neural lips of the groove subsequently fuse, thus turning the groove into the neural tube (Figures 2-1 and 2-2). At the site of fusion, between the ectoderm and the neuroepithelium (the epithelium of the neural tube), neuroepithelial cells proliferate to form the neural crest cells and migrate sideways into the paraxial and lateral mesoderm. These neural crest cells mix with mesodermal cells and form the secondary mesenchyme; this secondary mesenchyme forms the main mesodermal structures of the eye. Therefore the eye develops from the neural ectoderm, neural crest, and surface ectoderm, with minor contributions from the mesoderm.
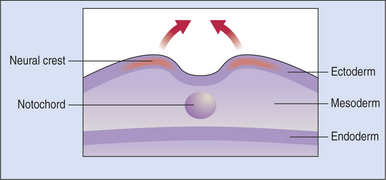
Figure 2-1 Developing embryo at the start of neural tube formation.
(From Yanoff M, Duker J [2004]: Ophthalmology, 2nd ed. Mosby, St. Louis.)
The anterior end of the neural tube enlarges and bends down to form the primordia of the CNS. On its outer surface, on both sides, appear two small pits called the optic grooves or optic pits. These pits, which appear on day 13 of gestation in the dog, are the anlage of the eyes (see Figure 2-2, G).
With the closure of the anterior end of the neural tube, intratubular fluid accumulates and its pressure causes the evagination of the optic grooves and their transformation into the two optic vesicles (Figures 2-3 and 2-4). This marks the beginning of organogenesis. In the dog this event occurs on the fifteenth day of gestation. The lumen of the neural tube remains connected to the cavities of the optic vesicles by two optic stalks (see Figure 2-4, D). Under the pressure of the intratubular (intraventricular) fluid, the optic vesicles continue to enlarge and bulge, eventually coming in contact with the surface ectoderm. At the site of contact with the optic vesicle the surface ectoderm thickens and forms the lens placode (Figures 2-3; 2-4, C; and 2-5). The contact of the optic vesicle with the surface ectoderm serves as an induction for the optic vesicle to start invaginating, thus forming the double-layered optic cup (see Figures 2-3 and 2-4, E and F).
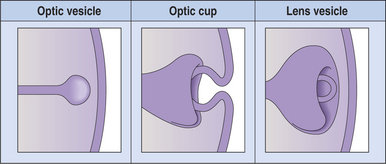
Figure 2-3 Formation of the optic vesicle, optic cup, and lens vesicle.
(From Yanoff M, Duker J [2004]: Ophthalmology, 2nd ed. Mosby, St. Louis.)
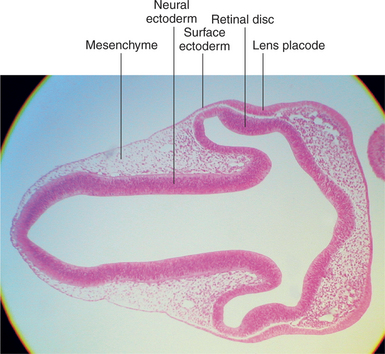
Figure 2-5 Light micrograph of 6-mm pig embryo showing thickening of lens placode.
(From Remington LA [2005]: Clinical Anatomy of the Visual System, 2nd ed. Butterworth-Heinemann, St. Louis.)
The invagination of the vesicle progresses from inferior to superior but is not completed on the ventral side of the optic cup, where a fissure, called the embryonic optic fissure, remains. The double layers of the optic cup are aligned on both sides of the fissure, which extends posteriorly under the optic stalk. This fissure allows the secondary mesenchyme present around the cup to penetrate into the cavity of the optic cup to form the hyaloid vascular system (twenty-fifth day of gestation in the dog) (see Figure 2-4, G and H). This fissure gradually closes leaving a small aperture at the anterior end of the optic stalk, through which the hyaloid artery passes (Figure 2-6). The hyaloid artery supplies the inner layers of the optic cup and developing lens vesicle. The two lips of the optic fissure fuse anteriorly to the optic stalk. The fusion process progresses anteriorly and posteriorly, eventually causing closure of the optic cup and allowing intraocular pressure to build up (see Figure 2-6).
The lens placode thickens to become the lens vesicle. Following the invagination of the optic vesicle, the lens vesicle finds itself embedded inside the cavity of the cup (see Figure 2-4, F and H). Anteriorly, the hyaloid artery gives branches, the tunica vasculosa lentis, which cover the posterior and lateral faces of the lens. This vascular network supplies the metabolic requirements of the lens during development. The hyaloid vascular system disappears at advanced stages of the development or during the postnatal period. In dogs remnants of the hyaloid system might remain visible until the fourth postnatal month, whereas in cattle they may persist until 12 months of age. In humans, but not in domestic animals, the caudal portions of the hyaloid artery and vein transform themselves into the central retinal artery and vein.
Retina
The optic cup is lined by two layers of epithelium of neuroectodermal origin (see Figure 2-4, H). The inner layer, facing the vitreous, is nonpigmented, but the outer layer, facing the (future) sclera, is pigmented. The anterior rim of the cup will form the anterior uvea (ciliary body and iris), and the posterior part of the cup will form the retina. The two epithelial layers of the optic cup will form the two epithelial layers of the retina and the anterior uvea.
The outer epithelial layer of the posterior optic cup forms the pigment epithelium of the retina. The inner epithelial layer forms the sensory retina (Figure 2-7). The two layers of the posterior optic cup are separated by the intraretinal space representing the cavity of the optic vesicle, which has gradually been obliterated during the invagination of the optic vesicle. Diseases of the posterior segment of the eye cause retinal detachment in this space as the sensory retina separates from the pigment epithelium.
The common neuroblastic layer, which is the nuclear portion of the sensory retina, divides into an outer neuroblastic layer and an inner neuroblastic layer (see Figure 2-7). These outer and inner neuroblastic layers are separated by the fiber layer of Chievitz. The cells of the outer neuroblastic layer differentiate into cones and rods externally and horizontal cells internally. The cells of the inner neuroblastic layer differentiate into ganglion cells, amacrine cells, bipolar cells, and Müller’s cells. The rods and cones (i.e., the photoreceptors) form the outer retina and are adjacent to the choroid and sclera (Figure 2-8). The ganglion cell layer, which originated in the inner neuroblastic layer, is called the inner retina because it is adjacent to the vitreous. The resulting retina is called an inverted retina because the photoreceptors are close to the outer layers of the eye and light must pass through all of the retinal layers to reach the photoreceptors. The reason for this arrangement is to place the photoreceptors next to the choroid, thus giving these cells, which have very high metabolic requirements, their own “private” blood supply.
Optic Nerve
Axons from the ganglion cells grow toward the optic stalk, thus forming the nerve fiber layer, the innermost layer of the retina (see Figures 2-6, B and D; and 2-8). Axons from throughout the entire retina converge on the optic disc, where they form into bundles collectively known as the optic nerve (cranial nerve II) (Figure 2-9). The axons of optic nerve extend posteriorly to form the optic chiasm and optic tracts before making their first synapse at the lateral geniculate nucleus.
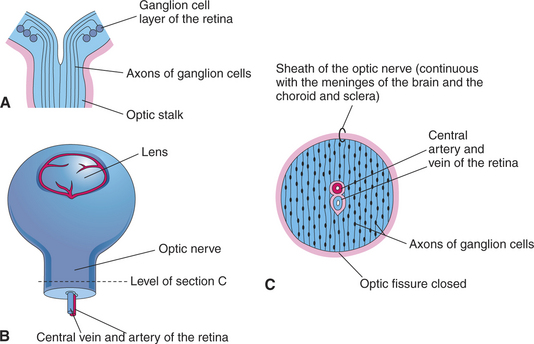
Figure 2-9 Formation of optic nerve. A, Axons of ganglion cells of the retina grow through optic stalk to brain. B, Transverse section through the optic stalk, showing the formation of the optic nerve. The optic nerve is formed after closure of the optic fissure (this stage follows the stage shown in Figure 2-6, C). C, The lumen of the optic stalk is obliterated as axons of ganglion cells accumulate in the inner layer of the stalk.
(From Remington LA [2005]: Clinical Anatomy of the Visual System, 2nd ed. Butterworth-Heinemann, St. Louis.)
As the ganglion cell axons collect at the optic disc they displace primitive neuroectodermal cells forward, into the vitreous cavity. These displaced cells form a glial sheath around the hyaloid artery. At the disc, the same cells may form an agglomeration called Bergmeister’s papilla, which protrudes into the vitreous. The papilla may persist into adult life (especially in ruminants), or it may atrophy, thus forming a depression, known as the physiologic optic cup, in the optic disc (Figure 2-10). In newborns this physiologic optic cup may be confused with a coloboma of the optic disc. In patients with glaucoma the physiologic optic cup may enlarge owing to the forces of the increased intraocular pressure on this region of the eye.
Vitreous
The embryonic vitreous consists of the primary, secondary, and tertiary vitreous (Figure 2-11). The primary vitreous develops with the hyaloid vasculature (Figure 2-12). It has mesenchymal, neuroectodermal, and ectodermal components. The mesenchymal elements enter posteriorly with the hyaloid vessels, and anteriorly through the space between the anterior rim of the optic cup and the lens vesicle. The ectodermal elements are the fibrils produced by the posterior face of the lens. The primary vitreous also contains neuroectodermal elements, which consist of the fibrils produced by the inner limiting membrane of the retina. The secondary vitreous is the “definitive” vitreous that will persist into adulthood. It is denser, is more homogeneous and avascular, and is laid down around the primary vitreous (see Figures 2-11, B, and 2-13). It is also secreted by the inner limiting membrane of the retina. The tertiary vitreous is secreted by the ciliary epithelium. Bundles of fibers extend from the ciliary epithelium toward the lens equator, covering the secondary vitreous anteriorly (see Figure 2-11, C). In the adult they persist as lens zonules (suspensory ligament of the lens).
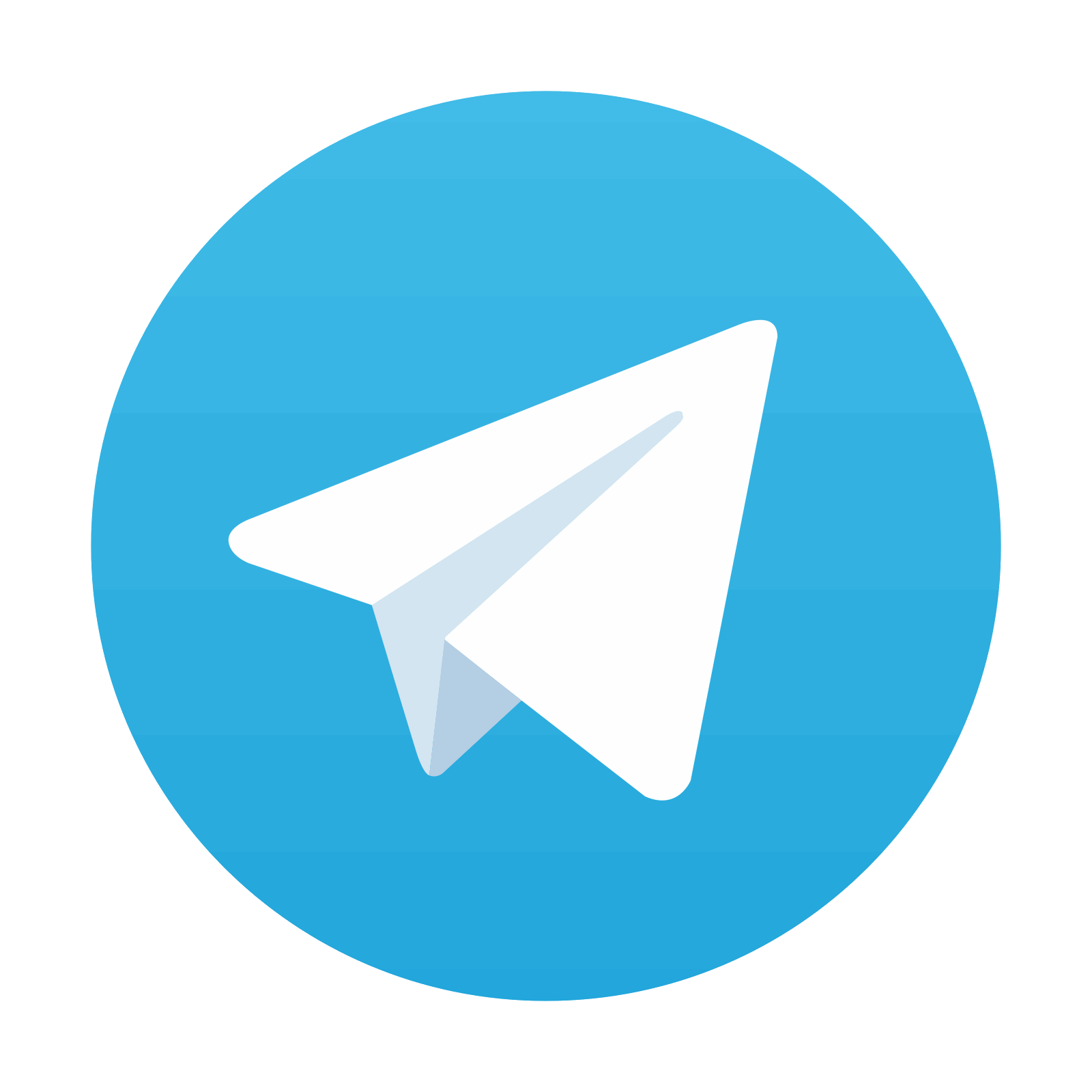
Stay updated, free articles. Join our Telegram channel

Full access? Get Clinical Tree
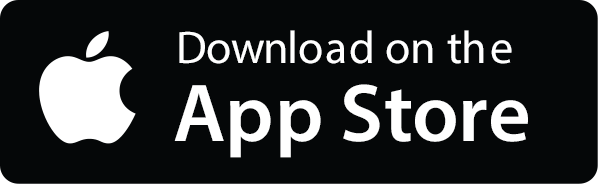
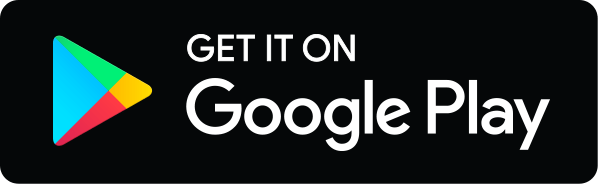