Chapter 10 Cranial nerves
Functional classification of cranial nerve nuclei
Auditory reflexes, and the brainstem auditory evoked response
Balance and the vestibular system
Taste and sensory input from the pharynx and viscera
Parasympathetic innervation of the eye, head and body
Innervation of the pharynx, larynx and oesophagus
Key points
Twelve pairs of cranial nerves innervate the head and extend into the body.
Individual nerves have specific sensory and/or motor, somatic and/or autonomic functions.
Knowledge of the location and action of individual cranial nerves is critical for the interpretation of the neurological examination (Tables 10.1, 10.2).
Cranial nerves are numbered sequentially from rostral to caudal based on the site of their attachment to the brain. CNN I and II attach to the forebrain; CNN III and IV are associated with the midbrain (rostral brainstem); CN V to XII are associated with the mid and caudal brainstem.
Cranial nerves III–XII neurons are arranged in nuclei in the brainstem.
Cranial nerves and their CNS components are bilaterally paired.
Most cranial nerves, excluding the optic and olfactory nerves, have a peripheral portion that is ensheathed/myelinated by Schwann cells.
The vast majority of vertebrates have 12 pairs of cranial nerves (CNN), but there may be only ten pairs in amphibians and fish. Cranial nerves are numbered, using Roman numerals, for their point of attachment to the brain. The first cranial nerve (CN I) attaches to the most rostral aspect of the forebrain as the olfactory bulb, whilst CN XII is attached to the caudal medulla oblongata (Fig. 10.1). The others attach, in sequence, between these two end points.
Named cranial nerve nuclei III–XII are found in the brainstem; they are bilaterally paired (Fig. 10.2). Their location relative to the sulcus limitans determines their function. Sensory cranial nerve nuclei are located dorsal to the sulcus limitans, parasympathetic (autonomic) nuclei are located lateral to it, and motor nuclei are ventral to it (Figs. 1.7, 2.3A) (Note: an exception to this is the location of the vestibular nuclei, which are sited dorsal and lateral to the sulcus limitans, despite having both sensory and motor functions). Remembering this anatomical arrangement is useful, as when the clinician is looking at a cross-section of the brainstem (e.g. MR image or tissue section), they can make an educated assumption about function of grey matter based on its dorsoventral position.
Functional classification of cranial nerve nuclei
Cranial nerves may have one type of function only, or have several functions. It should be noted that even the ‘motor only’ nerves may contain proprioceptive afferent fibres. Motor fibres of cranial nerves form the lower motor neurons (LMNs) of the head region (Table 10.3). Note that CN VIII has traditionally been described as purely sensory, however efferent fibres have been described in both the vestibular and cochlear portions of CN VIII (Chapter 10, olivocochlear reflex).
Table 10.3 General functions of cranial nerves
Sensory only | I, II, |
Motor only | III, IV, VI, XI, XII |
Mixed sensory and motor | V, VII, VIII*, IX, X, |
Autonomic (parasympathetic) | III, VII, IX, X |
* efferent components have been described in CN VIII
Rather than considering the 12 cranial nerves in isolation, it is useful to group them by function.
Olfaction
Key points
Odoriferous substances stimulate olfactory neurons. Action potentials pass caudally into the cranial vault, synapsing in the olfactory bulb. Olfactory tract axons project to both sides of the forebrain for olfactory perception, stimulation of behavioural responses and to the brainstem for reflex function.
Input from the vomeronasal organ is via the olfactory nerve and may stimulate the Flehmen reaction during sexual activity in some species.
The name olfactory ‘nerve’ is actually a misnomer since it consists entirely of CNS tissue (see p1), however in humans the olfactory bulb is so diminutive as to resemble a nerve. In veterinary species, the olfactory bulb is prominent (Figs. 10.3, A2, A3, A10).
Axons from the olfactory mucosa and the vomeronasal organ pass through the cribiform plate into the cranial vault and synapse on the olfactory bulb neurons. Post-synaptic axons travel caudally in the olfactory tract of the olfactory peduncle. The tract splits into lateral, intermediate and medial stria (see Fig. A11). The axons of the lateral stria synapse in the olfactory tubercle and pass to the cortex of the piriform lobe for olfactory perception. Fibres also connect to the limbic system (see Chapter 11). More medial fibres pass to the septal nuclei, which are located between the rostral aspects of the lateral ventricles, and to the hypothalamus and reticular formation of the brainstem. Fibres also decussate via the rostral commissure and pass to the contralateral olfactory bulb. The rostral commissure also interconnects the two piriform lobes. Through the connections to the limbic system, cerebral cortex and hypothalamus, olfaction can be a potent stimulator of behaviour and emotional states. Connections to the brainstem permit olfacto-visceral reflexes such as salivation in response to olfactory stimulation (Fig. 10.4).
Vision and CN II functions
Key points
The optic nerve is myelinated by oligodendrocytes. It is the only part of the CNS that can be observed on clinical examination.
Photoreceptors in the deepest part of the retina convert light into action potentials. The action potentials are modified by superficial layers of neurons, and exit the retina as axons in the optic nerve.
The majority of optic nerve axons decussate, but the degree of decussation depends on the type of animal and correlates inversely with the degree of binocular vision.
Caudal to the optic chiasm the majority of optic nerve axons travel to the lateral geniculate nucleus of the thalamus and then to the visual cortex for visual perception. The remaining axons course to the midbrain for reflex function.
General anatomy
The optic chiasm and decussation
The optic nerve extends caudally from the retina, through the optic foramen in the presphenoid bone into the neurocranium. It joins the ventral aspect of the diencephalon at the optic chiasm, just rostral to the hypophysis (pituitary gland). In general, the majority of axons decussate, but the degree of decussation depends on the type of animal. In fishes and birds, all fibres decussate (Fig. 10.10). In mammals, there is partial decussation (ungulates about 80–90%, dogs 75%, cats 65%, primates 50%).
Light also crosses in the lens of the eye, thus axons decussating at the optic chiasm come from the nasal retina and temporal field of view, while the temporal retinal axons receiving the nasal field of view, remain ipsilateral (see Fig. 10.5).
The visual pathway continues caudal to the optic chiasm as the optic tracts. In animals in which there is partial decussation at the optic chiasm, each optic tract contains axons from the contralateral nasal field and the ipsilateral temporal field. Thus the optic tract contains axons from retinal areas of the two eyes that are receiving input from the same part of the visual field (Fig. 10.5A).
The placement of the eyes and decussation of the optic chiasm is functionally significant. Animals with laterally placed eyes, e.g. ungulates/prey animals (Fig. 10.5B) have wide fields of view and a high percentage of fibres decussate at the optic chiasm. Thus the majority of visual input is processed in the contralateral visual cortex. A wide field of view permits these animals to see behind them; this is useful to detect danger such as stalking predators. But depth of field/binocular vision requires processing of visual input from both eyes in the same visual cortex, thus prey species have poor binocular vision. Conversely, animals with eyes that face forward, like the cat, have smaller fields of view, but have better binocular vision due to decreased decussation at the optic chiasm. This results in the animal receiving input from each eye on both sides of the brain. For example in Fig. 10.5A, input from the orange diamond located in the left side of the visual field will project to the nasal retina of the left eye and the temporal retina of the right eye. The input from those retinal areas will both end up in the same visual cortex – that is the right side one. Thus the same region of the brain processes two sets of information seen by the separate eyes, about the same object. This dual perspective results in binocular vision which is essential for depth perception. Depth perception is required for accurate judgement of distance and how fast an object is moving, therefore it is essential for predatory animals. The ability to perform coordinated, conjugate eye movements, including convergence (focussing both eyes on the same object) is also greater in animals with forward-facing eyes and reduced decussation.
Post-chiasmatic visual pathways
From the optic chiasm, axons course caudodorsolaterally in the optic tracts. The majority of axons connect to lateral geniculate nucleus (Fig. A17) of the caudal diencephalon (metathalamus) and then via the optic radiation to the visual cortex for visual perception. Alternatively, axons travel to the midbrain for reflex function (see Visual reflexes). Using the cat as an example, approximately 80% of fibres from each eye go to the lateral geniculate nuclei and 20% to the midbrain.
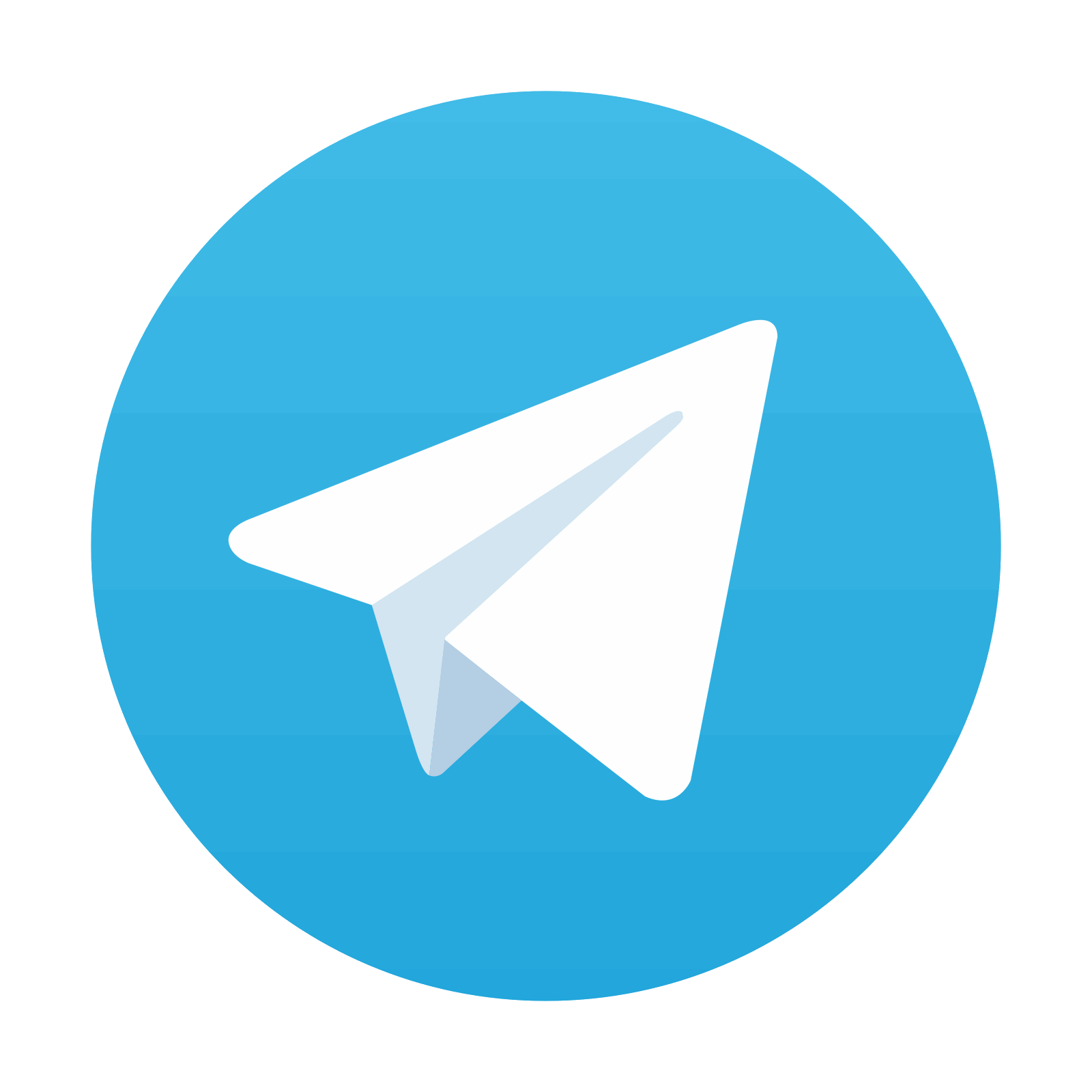
Stay updated, free articles. Join our Telegram channel

Full access? Get Clinical Tree
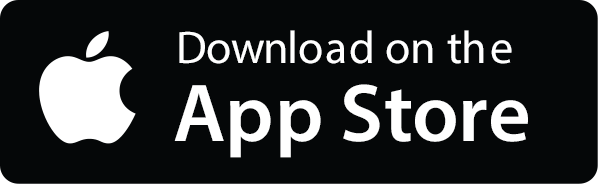
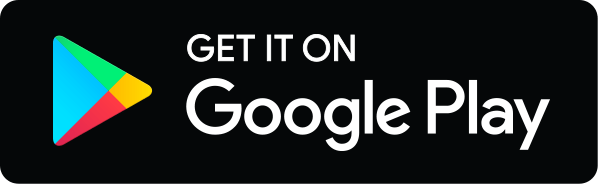