Chapter 21 Clinical Reproductive Endocrinology B. Chemical Classes of Reproductive Hormones D. Local Conversion of Steroids in Target Tissues E. Synthesis and Clearance of Hormones III. Physiology of Reproductive Hormones in the Female B. Control of the Corpus Luteum IV. Clinical Aspects of Reproductive Endocrinology B. Analysis and Storage Effects Clinical reproductive endocrinology includes the study of diseases and secretory status of the endocrine glands involved in reproduction and their secretory products, the reproductive hormones. To obtain a satisfactory understanding of the complex endocrinological events that occur during normal and abnormal reproductive function, it is necessary to quantify specific hormones. Major progress in hormone analytical techniques occurred as the result of the development of immunoassay and related systems. The first of the assays, radioimmunoassay, was developed in the late 1950s (Berson and Yalow, 1959) with the competitive protein binding assay following a few years later (Murphy, 1964). Nonradiometric assays (e.g., enzyme immunoassay) were developed in the 1970s (Engvall and Perlmann, 1971; van Weemen and Schuurs, 1971). These assay systems have the characteristics of being sensitive, specific, relatively inexpensive, and requiring small amounts of assay material. They have been of special value for studying endocrinological reproductive function in domestic animals in that they have made possible the study of dynamic endocrine changes through the assay of serial blood samples from the same animal. The immunoassay systems have also been useful as diagnostic aids for identifying and elucidating clinical reproductive problems. In clinical practice, these methods are important from both a diagnostic and therapy-monitoring point of view. Because this book deals with clinical biochemistry in domestic animals, this chapter emphasizes the determination of hormones and the use of the data as diagnostic aids. General reproductive endocrinology in domestic species is broadly covered. Readers specifically interested in this subject are referred to specialized books dealing with this matter. The best understood humoral control system in the body is the endocrine system. This system uses specific messengers, termed hormones, to regulate important body functions. By the classical definition, hormones are chemical substances synthesized and secreted by ductless endocrine glands in minute quantities directly into the blood vascular system and are transported to a remote target organ where they regulate the rates of specific biochemical processes. The classic endocrine glands include the pituitary, thyroid, parathyroid, adrenal, pancreas, ovary, testis, placenta, and pineal gland. In the case of reproduction, the pituitary and pineal glands, the gonads, and the placenta play a primary role in controlling the system. Other endocrine glands such as the adrenal and thyroid glands also have some influence on reproductive function. It has also been shown that other organs like the uterus and the hypothalamus, although they may not fulfill the strict requirement of being defined as endocrine glands, can synthesize and secrete hormones that have a profound influence on reproductive function. a. Releasing Hormones Several types of hormones are involved in the regulation of reproduction. Releasing hormones are peptide hormones, which are produced within the hypothalamus and transferred via the hypothalamo-hypophyseal portal veins to the adenohypophysis, where they regulate the synthesis or release of adenohypophyseal hormones. Gonadotropin-releasing hormone I (GnRH), a decapeptide with the sequence pGlu-His-Trp-Ser-Tyr-Gly-Leu-Arg-Pro-Gly-NH2, regulates the release of two important reproductive hormones, luteinizing hormone (LH) and follicle-stimulating hormone (FSH). A conserved second GnRH (GnRH-II) form exists in some mammals but appears not to have importance for gonadotropin release (Millar, 2005). Thyrotropin-releasing hormone, a tripeptide (pGlu-His-Pro-NH2), which regulates the synthesis and release of thyroid-stimulating hormone (TSH), also causes the release of prolactin in several species. GnRH is released in a pulsatile fashion, where the frequency of peaks varies according to reproductive status (see Clarke and Pompolo, 2005). Recently discovered neuropeptides have shed some light on the central regulation of GnRH release. Kisspeptin (or mestatin) and its receptor (G protein-coupled receptor 54) are expressed in the hypothalamus in close connection with GnRH-neurons. Kisspeptin is regulated by sex steroids and can directly activate GnRH neurons and induce GnRH secretion; thus it may be an important regulator of the reproductive axis and puberty (Seminara, 2005). First discovered in birds (Tsutsui et al., 2000), gonadotropin-inhibiting hormone (GnIH) has also been demonstrated in mammals, where its hypothalamic localization, association with steroid receptors, and inhibiting effect on gonadotropin secretion suggest an important role in the reproductive axis (Kriegsfeld et al., 2006). b. Hypophyseal Hormones Luteinizing hormone (LH) and follicle-stimulating hormone (FSH) are glycoproteins containing 13% to 25% carbohydrate. The molecular weight of LH in domestic animals (bovine, ovine, porcine, equine) is about 30,000. Ovine and equine FSH have molecular weights of about 32,000. Prolactin is a protein with a molecular weight of approximately 23,000 (bovine, ovine, porcine). Luteinizing hormone and FSH, as well as TSH, consist of two nonidentical subunits designated α and β. Within a species, the α-subunit is identical for these glycoprotein hormones, whereas the β-subunit is unique for each hormone and determines the biological activity. Individual subunits by themselves possess little or no biological activity and are probably not released into the circulatory system under normal physiological conditions. The cells of the adenohypophysis can be divided into basophiles (affinity for basic stains) and acidophiles (affinity for acid stains). Luteinizing hormone and FSH are produced within basophilic cells; it has been demonstrated that LH and FSH can be present within the same cell (the gonadotrope). Prolactin, on the other hand, has been localized in acidophilic cells. Gonadotropes have G protein-coupled GnRH-receptors on their surface, and the secretion of LH and FSH during the ovarian cycle is controlled by changes in the frequency of GnRH pulses. Except during the preovulatory LH surge in the estrus cycle, there is a clear difference in the patterns of FSH and LH secretion, as measured in the peripheral circulation, and only LH is released in a clear pulsatile manner reflecting GnRH secretion (see Pawson and McNeilly, 2005). c. Neurohypophyseal Hormones The posterior pituitary is responsible for storage and release of oxytocin, an important reproductive hormone, and anti-diuretic hormone (vasopressin). These two hormones are synthesized primarily in the regions of the paraventricular nucleus and supraoptic nucleus of the hypothalamus. The hormones are transported to the posterior pituitary by axoplasmic fluid. Release of these hormones occurs as a result of stimulation of the nerve cell bodies in the nuclei. Oxytocin is an octapeptide with a molecular weight of 1000. d. Gonadal Activins and Inhibins Activins and inhibins are structurally related proteins belonging to the transforming growth-factor-β (TGF-β) superfamily and have impact on the endocrine reproductive axis. Inhibins and activins are made up of two peptide chains. Inhibins form α–β dimers and activins β–β dimers. There are two varieties of the β-unit (called A and B) producing two forms of inhibin, inhibin A (α–βA), and inhibin B (α–βB), and three forms of activin, activin A (βA-βA), activin B (βB-βB), and activin C (βA-βB). Activins and inhibins secreted from the gonads act antagonistically on the gonadotropes to regulate FSH release. In the female, activins have several autocrine/paracrine functions in reproductive tissues and ovary activin A has been indicated as an endocrine stimulator of FSH release. In the male, inhibin is produced by Sertoli cells and function as a negative feedback regulator of pituitary FSH, probably in synergy with testosterone. In most species (e.g., bull, boar, and stallion), the dominant testicular form is inhibin B, whereas in the ram, inhibin A appears to be the dominant inhibin of the testicles of adult males (McNeilly et al., 2002). A gonadotropin, renamed equine chorionic gonadotropin (eCG) because of its close relationship with human chorionic gonadotropin (hCG) (Farmer and Papkoff, 1979), was formerly called pregnant mares’ serum gonadotropin (PMSG) and is produced by mares during early pregnancy (day 40 to 140) by fetal trophoblastic cells of the chorionic girdle, which attach to, invade, and phagocytose the maternal epithelium and become embedded within the uterus as specialized endometrial cups. This process begins on day 36 of pregnancy. No specific placental gonadotropins have been demonstrated in other domestic species. Steroid hormones are derived from a common precursor molecule, cholesterol, via the metabolic pathway schematically outlined in Figure 21-1. More than 1500 biologically active steroids have been isolated from biological material or have been produced synthetically. The molecular weight of steroid hormones is low, usually below 500 (Table 21-1). Examples of steroids that play an important role in reproductive processes are estrogens, androgens, and progestagens, with the main source being the gonads. The structure of the most important sex steroids is presented in Figure 21-2. The most common steroid hormones are usually designated by a trivial name (e.g., estradiol, testosterone, or progesterone). The International Union of Pure and Applied Chemistry (IUPAC; www.iupac.org) has recommended systemic names for steroid hormones. These systemic names describe the chemical and stereoisomeric characteristics of the particular steroid hormone (Table 21-1). FIGURE 21-1 Pathway for the synthesis of biologically active steroids from acetate. The steroids secreted from the gonads and the adrenals are formed from acetate and cholesterol. Prostaglandins constitute a group of 20-carbon unsaturated fatty acids with molecular weights usually between 300 and 400 (Table 21-1). Prostaglandins are not hormones in the strictest sense, and the expressions “paracrine factor” or “local hormones” have been used to describe these substances. This is because prostaglandins are not secreted from any particular gland and the biological half-life of prostaglandins is usually extremely short, allowing, in most cases, only a local action. Several different prostaglandins are found in a number of types of mammalian tissues. One prostaglandin released from the uterus, prostaglandin F2α (PGF2α), plays an important role in regulating reproductive cycles in domestic species through the control of luteal activity in nonpregnant animals and the initiation of delivery in pregnant animals. The structures of prostaglandin F2α and its main metabolite, 15-keto-13,14-dihydroprostaglandin F2α, are presented in Figure 21-2. FIGURE 21-2 The number of sequence for the carbon atoms of the steroid skeleton and lettering sequence for the four rings are shown for testosterone. The structures of three other important sex steroid hormones, estrone, estradiol-17β, and progesterone, as well as the structure of prostaglandin F2α and its blood plasma metabolite 15-keto-13,14-dihydroprostaglandin F2α are also depicted. Because steroid hormones are fat soluble, they are able to enter all cells of the body as the lipid cellular boundaries present no barrier. Steroid hormone concentrations in plasma are very low as compared to many compounds including their important precursor, cholesterol. For example, plasma estrogens in nonpregnant domestic animals range from as low as 10 pmol/l to as high as 150 pmol/l. In this situation, most cells within the body have very low concentrations of estrogen. The specificity of tissue response occurs because cells of tissues that have need of estrogen stimulation should have receptors that enable those particular cells to concentrate the hormone within the cell and, more important, to elicit particular cellular responses. This explains the important generalization that specific tissue response requires specific receptors to be present within the cell, in this case for a particular steroid hormone. Binding of a hormone to a receptor in a target cell can be considered to be the primary event and the hormone receptor interaction will cause a measurable biological response that differs for different hormones. Receptors have a limited binding capacity (receptors are saturable thus limiting number of hormone molecules that can enter a target cell), they bind specific hormones (e.g., estrogen receptors are specific for estrogenic compounds), and upon binding a biological response will be created. Steroid hormones enter cells by passive diffusion and bind to receptors inside the cell. It is assumed that only nonprotein bound or free hormone can enter target cells. Traditionally, the steroid bound irreversible to the carrier protein have been considered to be virtually biologically inactive and only the minute quantity of free (nonprotein bound) steroid can enter the cell. However, studies suggest that sex steroids bound to the sex hormone-binding globulin can be internalized and as the carrier is degraded by lysosomes, the steroids are released to induce steroid-responsive genes (Hammes et al., 2005). When the steroid interacts with its receptor, a steroid-receptor complex is formed. The hormone-receptor complex is then activated and alters gene expression. The target cell responds by increased RNA synthesis with the transcription of specific mRNAs, which enters cytoplasm and stimulates protein synthesis. The specific effect of steroid hormones on target cells is an altered cell function related to a change in the pattern of protein synthesis. In addition to this classical genomic steroid action, rapid effects (within minutes) of steroid have also been described (see Bramely 2003; Kelly and Wagner, 1999). Protein hormones like GnRH, LH, and FSH do not enter the target cell to exert their effects but interact with their receptors, which are located on the plasma membranes of the cell, s.c. G protein-coupled receptors. The binding of the hormone to the cell surface receptor activates second messenger(s), for example, cyclic AMP (3′, 5′-AMP). The second messenger(s) is the intracellular mediator of many actions of LH and FSH in the ovary and the testis. The second messenger(s) is thought to activate another intracellular enzyme, protein kinase, which will influence the transport of cholesterol into the mitochondrion and the conversion of cholesterol to pregnenolone, which is the rate-limiting step in the biosynthetic pathway for the steroids that play a significant role in reproductive processes. The effects of steroid hormones on cells can be accentuated or modulated by the conversion of the entering hormone to another form. For example, many of the tissues that are particularly responsive to androgens have the enzyme 5α-reductase that converts testosterone to 5α-dihydrotestosterone (5α-DHT); 5α-DHT has a much higher affinity for the androgen receptor within the target cell, which makes 5α-DHT more biologically active than testosterone as to its androgenic effects. The androgenic potency of 5α-DHT is twice that of testosterone. Another important interconversion of steroid hormones is the one resulting from the increase in circulating cortisol concentrations that occur in the fetal lamb before parturition. The elevated fetal cortisol concentrations stimulate 17α-hydroxylase, C17-C20 lyase activity, and probably aromatase activity in the plaxcenta. This makes it possible for progesterone to be converted to estrogen synthesis in the placenta. Estrogens then affect the synthesis of prostaglandin F2α, which precipitates delivery. The interconversion of progesterone to estrogen is well documented in the sheep and probably also occurs in a similar way in the goat and the cow. The determination of concentrations of hormones in biological fluids including plasma, urine, saliva, milk, and feces has been useful in determining the reproductive status of animals. Although a number of factors can influence hormone concentrations, the overriding factors are synthesis and clearance. We are usually concerned about the rate of synthesis of a hormone from a particular endocrine gland because factors that govern clearance are usually stable, and the concentration of the hormone usually reflects the rate of synthesis or secretion. The synthesis of steroid hormones of the reproductive system is under the control of gonadotropins, which are released in pulsatile fashion. This has a profound influence on the secretion of testosterone in the male in that changes in pulsatile rate can occur a number of times a day with increases in pulse rate resulting in greatly increased concentrations of testosterone. For example, in males of many domestic species, testosterone values can range from 3.5 to 20 nmol/l within a period of a few hours with the extremes still representing normal production of testosterone by the testes. The usual judgment as to normalcy is based on an animal having at least the minimal or basal concentration. In the female, estrogen and progesterone synthesis by the ovary is also under the control of a pulsatile mode of gonadotropin secretion. The pulse rate usually remains relatively stable over certain periods of time so that fluctuations in concentration of these hormones are not as acute as for androgens. In the female, synthesis rates for ovarian steroid hormones are obviously related to ovarian function. Progesterone concentrations, relatively stable during the luteal phase of the estrus cycle, decline rapidly over a 24- to 36-h period during luteolysis. Estrogen values continually increase during the follicular phase of the cycle, declining with the onset of the gonadotropin preovulatory surge as the granulosa is converted from estrogen to progesterone production. Even though secretion rates can change for both progesterone and estrogen, analysis of these hormones usually brings useful information as to luteal or follicular activity, respectively. One other factor must be considered if one wishes to use hormone values (in blood, for example) as an indication for secretory activity of an endocrine organ (i.e., the conversion of steroid hormones by peripheral tissues). For example, in primates, estrone concentrations are derived mainly from the conversion of ovarian estradiol-17β and adrenal androstenedione by tissues such as the liver. Steroids are eliminated via conjugation with glucuronic acid or sulfates to form inactive mono- or diglucosiduronates or sulfates. These conjugates are all water soluble with excretion occurring via urine or bile (feces). The conjugation occurs mainly in the liver, and the conjugates formed lack steroidal activity. Steroids are also rendered inactive by their metabolism to compounds that have greatly reduced biological activity. In this way, steroids are rapidly cleared from the bloodstream. Clearance is defined as the volume of blood that would be totally cleared of a particular steroid per unit time. Clearance can thus be expressed as liters/minute, and the clearance for most steroid hormones is around 1 liter/minute. In most situations, the clearance rate of steroids is relatively constant, so that blood concentrations are a fairly good measure of fluctuations in production rates. Placental gonadotropic hormones like hCG and eCG are produced in high concentrations and have much longer half-lives than the pituitary gonadotropins and prolactin. The latter have half-lives that are around 10 to 30 min, whereas the corresponding figures for the placental hormones are from 1.5 days for hCG to 6 days for eCG. One exception is equine LH, which shows structural similarities to eCG and also has a much longer half-life (days) than LH from other species. The increased half-lives are due to the fact that the molecules are composed of a larger portion of carbohydrate moiety as compared to FSH and LH of most species. In the blood circulation, prostaglandins are rapidly metabolized to their respective 15-keto-13,14-dihydro compounds (Fig. 21-2). Primary prostaglandins like PGF2α have a half-life in the peripheral circulation that is less than 20 sec, whereas the 15-keto-13,14-dihydro-PGF2α have a somewhat longer half-life of about 8 min; 90% or more of PGF2α is metabolized by one passage through the lungs. The 15-keto metabolites are biologically inactive, and before being excreted into urine they are degraded into short dicarboxylic acids (Neff et al., 1981). The radioimmunoassay (RIA) technique was originally introduced for the measurement of plasma insulin (Berson and Yalow, 1959) and the enzyme immunoassay (EIA) techniques for the quantitative determination of immunoglobulin G (Engvall and Perlmann, 1971). The techniques are competitive and utilize the same basic principle, which is based on the ability of nonlabeled hormone to compete with a fixed amount of labeled (radioactive isotope or enzyme) hormone for the binding sites on a fixed amount of protein. The nonlabeled hormone reduces the number of free binding sites on the protein, thus decreasing the availability of the binding sites to the labeled hormone. At equilibrium, the free hormone is separated from the protein-bound hormone, and the reaction is quantified by the determination of the amount of labeled hormone that is antibody bound or free (Fig. 21-3). The degree of inhibition of binding of the labeled hormone to the binding protein is a function of the concentration of nonlabeled hormone present in the solution. As a basis for the quantification, a standard curve is developed with fixed amounts of labeled hormone and binding protein incubated together in the presence of a known and graded concentration of unlabelled hormone (Fig. 21-3). FIGURE 21-3 The principle of an immunoassay technique is based on the ability of the binding protein to bind the labeled hormone. Excess-labeled hormone as added to ensure saturation of the hormone-binding sites on the binding protein. The addition of increasing amounts of nonlabeled hormone (2,4, and 8 pmol) results in a proportional decrease in the quantity of labeled hormone bound to the binding protein. Separation of labeled hormone bound to the binding protein from free hormone must be achieved before quantification can be done. In the lower part of the figure, this reaction is depicted in three different ways. In the panel to the left and in the middle, the percentage-labeled hormone bound to the binding protein is on the ordinate, and the amount of hormone on a linear scale (left) and log scale (middle) is on the abscissa. In the panel to the right, the logit of the response variable is on the ordinate, and the amount of hormone is plotted on a log scale. The method depicted in the middle and to the right can be used for determination of parallelism. The logit/log transformation (right) is frequently used when computers analyze immunoassay data. Certain disadvantages exist to the use of radioisotopes as labels in immunoassays. Among those are limited shelf life and stability of radiolabel compounds, need for relatively expensive counting equipment (especially for tritium-labeled compounds), need for well-trained personnel and specialized laboratory, and problems in disposal of radioactive waste. Consequently, great progress has been made to develop assays that use nonisotopic labels such as enzymes, fluorogens, and chemiluminescent precursors. Enzyme immunoassays can be as sensitive, accurate, and precise as radioimmunoassays; the specificity depends on the quality of the antibody as is true for radioimmunoassay systems (Munro and Stabenfeldt, 1984). Of particular interest in domestic animals has been their use in the determination of progesterone in blood (Lopate and Threlfall, 1991; Meyers et al., 1988; Munro and Stabenfeldt, 1984) and in milk (Allen and Foote, 1988; Arnstadt and Cleere, 1981; Etherington et al., 1991; Sauer et al., 1981). Enzyme immunoassay in the laboratory setting is very efficient, particularly as concerns the time required for the assay reaction (2 h or less), ease of separation of bound from free hormone when using microtiter plate system (30 sec to wash 96 wells on a microtiter plate), and speed of end point analysis (optical densities can be determined in 1 min for a 96 well microtiter plate). Among the various approaches to enzyme immunoassay, the double antibody sandwich method for determination of larger hormones is frequently used. In this assay system, the plastic wells are coated with antibody. The sample to be processed is then added, and its hormone binds to the antibody-coated plastic well. A second enzyme-labeled antibody directed against another epitope of the hormone is then added. The amount of enzyme-labeled antibody bound is directly proportional to the amount of hormone in the sample. There are two advantages with this methodology: (1) the hormone does not need to be isolated for labeling, and (2) the same general technique can be used to label different antibodies. The method can only be used to assay hormones with at least two binding sites, and it is thus unsuitable for measurement of low-molecular-weight hormones like steroid hormones. The analytical equipment used for enzyme immunoassay can be used for a variety of determinations including those involved in disease surveillance and drug analysis. This allows for the sharing of one specialized spectrophotometer among several disciplines at a great reduction in cost. Another benefit is that the analytical equipment needs little maintenance. The elimination of the problems engendered by use of radioisotopes is a major advantage for enzyme immunoassay. This and the fact that color change is fundamental to enzyme immunoassay means that the assay can be used visually to determine the presence or absence of a corpus luteum (CL) in domestic and other species. Enzyme immunoassay-based analytical systems for progesterone in blood or milk can be used on the farm beside the animal to directly assess the functional ovarian status of an animal (Herrler et al., 1990; Matsas et al., 1992; Nebel et al., 1989; Romagnolo and Nebel, 1991). Automatized immunoassay instrumentation has been developed mainly for the human medical market, but some systems have specific veterinary applications (e.g., chemiluminescent-labeled immunoassays by DPC), and as some hormones (e.g., steroids) cross-react between species, these automatized immunoassay instruments can be useful in a veterinary diagnostic lab with high throughput. Immunoassay techniques utilize antibodies as binding proteins. Hormones such as LH and FSH, which are glycoproteins with molecular weights of around 30,000, are antigenic because of their size and chemical composition. In general, a lower level of purity is required of the polypeptide hormones for antibody production as compared to the hormone used in the labeling procedure. If an assay for bovine LH utilizes an antibody to bovine LH, radio- or enzyme-labeled bovine LH as tracer, and bovine LH as the standard, the assay system is completely species specific and is said to be of the homologous type. Such a system represents the ideal immunoassay system for measuring a polypeptide hormone. Because of the limited availability or lack of suitable purity of polypeptide hormone preparations, heterologous assay systems have been developed. In these cases, an antiserum to a polypeptide hormone of one species has been used to determine the same polypeptide hormone in another species. The standard hormone used for quantification of the assay should, however, originate from the same species for which the measurements are performed. Polypeptide hormone antisera are available that show a high degree of cross-reactivity. One such antiserum of special interest in the field of reproductive hormones in domestic species is a polyclonal LH antiserum raised against ovine LH (Niswender et al., 1969). This antiserum reacts specifically with LH from other species and has been used to determine LH in approximately 45 species including the cow, sheep, pig, cat, and dog (Madej and Linde-Forsberg, 1991; Millar and Aehnelt, 1977). A monoclonal antibody generated against bovine LH has been reported to have high cross-reactivity between species (Bravo et al., 1992; Forsberg et al., 1993b; Matteri et al., 1987). Steroid hormones and prostaglandins have considerably lower molecular weights and thus are not immunogenic per se. However, these structures can be rendered immunogenic if covalently linked to large carrier molecules such as bovine serum albumin, and specific antibodies can be elicited. For such a hormone-protein conjugate to be immunogenic, approximately 10 to 20 hormone molecules should be present per molecule of protein. In the case of bovine serum albumin, about 30% of the sites available for conjugation should be occupied. Most naturally occurring steroid hormones and prostaglandins contain hydroxyl or ketone groups, which are used to prepare derivatives containing active groups such as carboxyl or amino groups. These groups are then activated so that they react with amino or carboxyl groups of the protein molecule. The specificity of the antisera obtained by immunization with a steroid-protein conjugate depends on the site used for conjugating the steroid to the protein. More specific antisera are obtained if the hapten (steroid) is attached to the protein at a site remote from the characteristic functional groups of the hormone (Lindner et al., 1972). The species most frequently used to produce polyclonal antibodies are sheep and rabbits. One of the most popular and efficient schedules for immunization involves multiple injections in the back and neck of the animal of the antigen emulsified in complete Freund’s adjuvant (Vaitakaitus et al., 1971). During immunization, the developing antibody titer is monitored, and a relatively large number of milliliters of serum can be obtained when a suitable titer has been achieved. A few milliliters of a high titer antiserum are usually sufficient for millions of immunoassay determinations. Antisera seem to be stable when stored at 220°C, although the usual preferred temperature is 270°C. The discovery that hybridomas could be used to produce an endless supply of antibodies with certain specificity (Köhler and Milstein, 1975) led to a new development in the technology of antibody production. The procedure involves the fusion of two cell lines: B lymphocytes selected for the production of a specific antibody and myeloma cells that have the capacity for permanent growth. Antibody production occurs by injection of the cell lines into mice; permanency is assured by maintaining a supply of cells in the frozen state. Monoclonal antibodies are useful for the quantitative immunoassay of hormones. They have the advantages of specificity, unlimited supply over time, and the possibility to standardize assay methods between laboratories; disadvantages are that they have lower affinities as compared to polyclonal antibodies and they do not always form precipitates with antigens. Mixing of monoclonal antibodies may increase affinity (Ehrlich et al., 1982). In radioimmunoassay techniques for polypeptide hormones, the antigen (hormone) is most commonly used for preparing the radioactive tracer. Usually, radioactive iodine, 125I, is used for radioiodination of the antigen. The two most frequently used techniques for iodination are the chloramine-T (Hunter and Greenwood, 1962), immobilized chloramine-T (Iodobeads; Markwell, 1982), and the lactoperoxidase procedures (Thorell and Johansson, 1971). Peptide hormones containing tyrosyl or histidyl residues can also be iodinated with these techniques. Many RIA systems for steroid hormones and prostaglandins utilize tritiated forms of these molecules, which are available commercially. Because tritium has a considerably longer half-life than iodine, tritium tracers can be used in many cases over several years, whereas the iodinated tracers often have to be prepared monthly. There are, however, certain advantages in using iodinated tracers for steroid hormones and prostaglandins in that simpler and cheaper counting systems can be used (i.e., gamma counting as opposed to liquid scintillation counting). Another advantage of radioiodine over tritium is its higher specific activity, which increases the sensitivity of the assay. Direct incorporation of iodine in the skeleton of steroid hormones results in a loss of the immunoreactivity. Thus, the approach taken for radioiodination of steroid hormones has been to link a tyrosyl or histidyl molecule to the steroid molecule, making direct radioiodination possible (Niswender, 1973), or to iodinate a compound such as tyramine and conjugate the iodinated compound to the steroid molecule (Lindberg and Edqvist, 1974). Most EIA systems for steroid hormones use horseradish peroxidase, alkaline phosphatase, or β-galactosidase as labels. The enzyme-labeled steroid is produced the same way as has been described for the synthesis of steroid protein conjugates for the production of antibodies. An essential part of any immunoassay system is an efficient procedure for the separation of antibody-bound and free hormone. Several different approaches have been taken to achieve a rapid and efficient separation. Frequently used separation procedures are based on antibodies coupled to an insoluble polymer, precipitation of antibodybound hormone, or adsorption of free hormone. Antibodies coupled to an insoluble polymer can be used for separating antibody-bound and free hormone in RIA procedures (Abraham, 1969). One procedure involves the decanting of polystyrene tubes in which antibodies have been adsorbed to the surface of the tube, which is followed by determination of radioactivity in the antibody-bound (contained in the tube) or free (contained in the eluent) form. In most EIA systems designed for low-molecular-weight hormones, antibody-bound hormone is measured after free hormone has been removed by washing the wells of the microtiter plate that have been previously coated with antibody. Another common procedure for RIA utilizes antibodies covalently coupled to an insoluble polymer granule. In this case, free and antibody-bound hormones are separated through centrifugation. After removal of the supernatant containing the free hormone, the antibody-bound radioactivity can be determined. Antibody-coated glass beads have been used in both RIA and EIA (Schmidt et al., 1993). Separation is achieved by washing the bead and then the radio or enzyme activity is determined. For steroid hormones, a traditional separation procedure is the adsorption of free hormone to dextran-coated charcoal. After the addition of the charcoal, the separation of free and antibody-bound steroids is achieved through centrifugation. This method is rapid and efficient in separating free and bound steroid hormones. However, the charcoal can also adsorb some of the antibody-bound steroid, which is called “stripping.” To control this, timing of the reaction is important. The reliability of immunoassay analyses depends on its specificity, sensitivity, accuracy, and precision. a. Specificity The specificity of an immunoassay, or freedom from interference by substances other than the one to be measured, depends on several different factors, the most important being the specificity of the antiserum used. Demonstration of specificity for the immunoassay of hormones that cannot easily be synthesized is relatively difficult and relies on indirect criteria because these molecules have to be isolated and purified from biological material with variable purity. A relatively common finding is the cross-reaction of TSH with antibodies to LH, and vice versa. Antibody specificity is usually demonstrated by testing the binding of hormones, other than the one intended to be measured, to the antibody. If, for example, bovine TSH significantly inhibits the binding of bovine LH to an antibody to bovine LH, this indicates that the antiserum used is nonspecific or that the TSH preparation contains LH. If the inhibition curves are parallel, the latter explanation is likely because the parallelism indicates the same binding kinetics. It should be noted that parallelism, in itself, is not adequate proof of specificity. As indicated previously, both LH and TSH are composed of two subunits, an α-subunit that is identical for the two hormones and a β-subunit that is unique for each hormone. It is possible that an antiserum could contain binding sites, which will react only with the α-subunit. In such a case, the dose-response curve of LH and TSH utilizing such an antisera will be parallel, the assay system will not be hormone specific, and thus the system will be invalid for the measurement of LH. Double antibody sandwich methods utilizing monoclonal or polyclonal antisera can partly reduce the problem. In the case of immunoassay techniques for steroid hormones and prostaglandins, the same proof of specificity has to be undertaken. Here the situation is simpler, because small-molecular-weight hormones can easily be purified and, in most cases, produced synthetically more easily. Some idea as to the specificity of an antiserum to a steroid hormone can be gained from the position of the steroid molecule that is used as the anchoring point to the protein (Fig. 21-2). If an antiserum to estradiol-17β is produced through the use of an antigen conjugated via the hydroxyl group at carbon 17 of the steroid, the resulting antiserum will react almost equally well with estrone and thus would have relatively poor specificity. This is because the only structural difference between the estradiol-17β and estrone molecules is the configuration at position 17 (Fig. 21-2). In general, steroid antibodies are more specific for the portion of the steroid molecule that protrudes from the carrier protein and less specific for the portion of the steroid used for linkage to the protein. Thus, in the case of estradiol-17β, highly specific antibodies have been developed after immunization with conjugates when carbon 6 of the B ring has been used as the site of attachment to the protein (Exley et al., 1971). Protein hormone determination by immunoassay is often performed in blood serum or plasma. The influence of serum or plasma on the binding of the tracer to the antibody must be investigated. The assay of different amounts of serum or plasma should result in curves parallel to those obtained with protein hormone standard. In some steroid hormone immunoassays, an organic solvent from a blood plasma sample extracts the hormone. Organic solvents can also be used as a means to selectively remove steroids from biological fluids. For example, many immunoassay procedures designed to measure progesterone utilize antisera developed against a progesterone-11 protein conjugate, which results in a minor cross-reaction with corticosteroids (Thorneycroft and Stone, 1972). By using a nonpolar solvent such as petroleum ether for the extraction of progesterone from serum or plasma samples, about 80% to 90% of progesterone is extracted leaving the more polar corticosteroids in the plasma (Johansson, 1969). The use of such a selective extraction system increases the overall assay specificity. Direct analytical systems for steroid hormones omitting the extraction step are also employed. Because steroid hormones in a blood sample to be analyzed are bound to carrier proteins, direct assay systems have to secure that all steroid molecules, both free and protein-bound steroids in the sample, are given equal opportunities to interact with the antibody used in the assay. The synthetic steroid danazol (17α,2,4-pregnandien-20-yno(2,3-d)isoxazol-17 ol) can be used to displace progesterone from the binding proteins (Carrière and Lee, 1994). Certain immunoassay systems may require purification of the plasma extract to achieve an acceptable specificity. The main problem in the immunoassay of primary prostaglandins is that they can continue to be formed in large amounts by platelets after the blood sample has been obtained (Samuelsson et al., 1975), thus the concentrations of PGF2α reported in blood serum or plasma can appear much higher than the actual values. The primary prostaglandins have a very short half-life in the circulation (Hamberg and Samuelsson, 1971) and are rapidly converted to their corresponding 15-keto-13,14-dihydro derivatives. The latter have considerably longer half-lives and occur in higher concentrations than the parent compounds (Beguin et al., 1972). Analysis of metabolites of prostaglandin F2α avoids the problem of the overestimation observed for the parent compound in that the metabolites are formed only within the body and values thus remain stable once a blood sample has been obtained. Radioimmunoassay systems utilizing antibodies to 9α,11α-dihydroxy-15-ketoprost-5-enoic acid and 5α,7α-dihydroxy-11-ketotetranorprosta-1,16-dioic acid have been developed (Granström and Samuelsson, 1972). Most problems involved in the determination of the primary prostaglandins are avoided if their main metabolites, the 15-keto-13, 14-dihydro compounds, are measured. b. Sensitivity The sensitivity of immunoassays is defined as the smallest quantity of hormone that the assay can reliably distinguish from a zero amount of the hormone. Usually two kinds of sensitivity are evaluated. The sensitivity of the standard curve is defined as the smallest amount of hormone that is significantly different from zero at the 95% confidence limit. However, the most meaningful sensitivity to establish is the smallest amount of hormone that can be measured per unit of biological fluid (e.g., per milliliter of blood plasma). c. Accuracy The accuracy of an assay is defined as the extent to which the measurement of a hormone agrees with the exact amount of the hormone. Accuracy is often determined by comparing immunoassay data with values determined by other procedures such as gravimetry, gas liquid chromatography, and mass spectrometry. For steroid hormones and prostaglandins, accuracy is also often determined by recovery experiments in which different amounts of hormones are added to a biological fluid (e.g., blood plasma), which contains low concentrations of the hormone; the amount of hormone measured in the assay is then compared with the amount of hormone added. d. Precision Two types of precision are usually evaluated. The within-assay precision is determined from duplicate measurements of the same sample within the same assay. The between-assay precision is determined from replicate analyses of the same sample in different assays. Usually the between-assay variance is greater than the within-assay variance. Assay variance should be checked continuously with each assay of a certain hormone by use of plasma pools containing set amounts of the hormone. Usually three different plasma sets containing low, medium, and high hormone concentrations are used. Within- and between-assay variations in immunoassay procedures are usually greater than for ordinary routine procedures used in clinical chemistry. This presentation is relatively brief as to its coverage of endocrinological events during the reproductive cycle. Readers interested in a more complete presentation of hormonal events involved in reproduction are referred to texts dealing specifically with the subject. Also, some hormones with some influence on reproductive processes, such as those of the thyroid, adrenal, and pineal glands, are not covered in this presentation. The major endocrine events that precede ovulation have been well documented in the cow (Chenault et al., 1975), ewe (Nett et al., 1974), sow (Shearer et al., 1972), mare (Evans and Irvine, 1975; Palmer and Jousset, 1975; Stabenfeldt et al., 1975), dog (Concannon et al., 1975), and cat (Shille et al., 1979b). In large domestic animals (cattle, horse, pig, sheep, and goat), follicle growth occurs during the luteal phase in spite of the inhibitory nature of progesterone, the main secretory product of the CL. Although follicles are usually not ovulated during the luteal phase in most species, the mare occasionally ovulates during the luteal phase. With regression of the CL, follicles grow rapidly before ovulation because of gonadotropin stimulation. The follicles secrete increasing amounts of estrogen during development, which is important for the onset of sexual receptivity as well as for the initiation of the surge release of gonadotropins that is essential for the ovulatory process (Fig. 21-4). Estrogens initiate the surge of LH and FSH through the release of GnRH (Moenter et al., 1990). In most species, the preovulatory surge of gonadotropins begins approximately 24 h before ovulation and is usually of short duration, (e.g., 8 to 10 h in the cow). The mare is an exception in that large amounts of LH are released during an 8- to 9-day period with ovulation occurring on the third day (Geschwind et al., 1975). Another important function of GnRH is to elicit sexual receptivity. Thus, the onset of the preovulatory LH surge and sexual receptivity is coordinated via GnRH synthesis and release. FIGURE 21-4
I. INTRODUCTION
A. Definition of Hormones
B. Chemical Classes of Reproductive Hormones
1. Peptide and Protein Hormones
2. Steroid Hormones
3. Prostaglandins
C. Hormone Receptors
D. Local Conversion of Steroids in Target Tissues
E. Synthesis and Clearance of Hormones
II. ASSAY METHODS
A. Immunoassay
1. Production of Antibodies
2. Labeled Hormone
3. Separation of Antibody-Bound and Free Hormone
4. Reliability Criteria
III. PHYSIOLOGY OF REPRODUCTIVE HORMONES IN THE FEMALE
A. Estrus Cycle
Stay updated, free articles. Join our Telegram channel

Full access? Get Clinical Tree
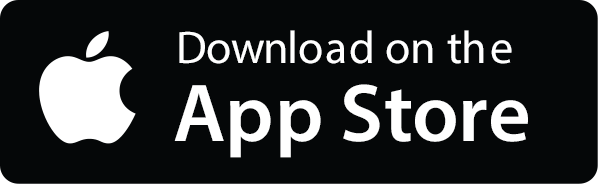
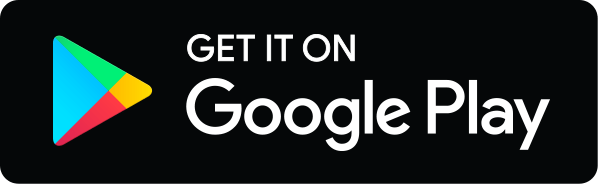