Chapter 27 Clinical Biochemistry in Toxicology IV. TOXINS AFFECTING SKELETAL AND CARDIAC MUSCLE V. TOXINS AFFECTING THE LUNG AND RESPIRATORY TRACT VI. TOXINS AFFECTING THE GASTROINTESTINAL TRACT VII. TOXINS AFFECTING ERYTHROCYTES AND THE HEMATOPOIETIC SYSTEM VIII. TOXINS AFFECTING HEMOGLOBIN AND OXIDATIVE METABOLISM IX. TOXINS AFFECTING THE ENDOCRINE SYSTEM X. TOXINS AFFECTING THE NERVOUS SYSTEM XI. TOXINS AFFECTING THE INTEGUMENT The availability of accurate historical information including a list of the animal species affected, clinical signs, toxins suspected, potential route of exposure, vehicle, relative amount, and timing of exposure is often a limiting factor in the diagnosis of toxic disease. This information permits the diagnostician to make a rational selection of samples and tests to be performed by considering the known target organs of the toxins suspected. Unfortunately, this information is often unavailable during the initial stages of an intoxication. In the absence of such detailed history, the identification of target organs using clinical biochemistry may help clinicians to create a list of potential toxins retrospectively. There are species differences in the most useful biochemical markers of organ-specific cellular injury and in the susceptibility to various toxins (Kramer and Hoffmann, 1997). For this reason, the following discussion is organized on an organ system and species basis. Neither bacterial toxins nor hereditary disease predisposing to intoxication will be discussed. Many toxins induce hepatic injury (Table 27-1). The susceptibility of the liver to toxic insult is in part a consequence of its location between the digestive tract and the rest of the body and the central role it plays in biotransformation and disposition of xenobiotics (Miyai, 1991; Snyder, 1979). Extrahepatic metabolism of toxins by mixed function oxidases may affect the target organ and potential hepatotoxicity of a given xenobiotic (Gram et al., 1986). A variety of factors including the induction of these enzyme systems by drugs (Snyder, 1979) and suppression of enzyme activity by infectious agents and cytokines (Monshouwer et al., 1995) may modify the response to a given toxin. Lipophilic compounds tend to be more hepatotoxic than hydrophilic ones because the latter are eliminated by the kidney (Kelly, 1993). Many toxins are hepatotoxic and nephrotoxic, however, and most toxins have multiple target organs. The cytosolic enzyme alanine aminotransferase (ALT) is found in both hepatocytes and skeletal muscle of animals. The dog and cat have high levels of ALT in hepatocytes, making it a useful marker for hepatocellular injury in these species (Stockham and Scott, 2002). The plasma half-life of this enzyme in the dog is estimated to be approximately 60 h. Increased serum levels parallel the magnitude of hepatocellular injury in acute disease. Several days following injury, ALT levels may be spuriously low. Therefore, ALT is not helpful in evaluating chronic liver disease. ALT levels also may be elevated by corticosteroid treatment and some anticonvulsant medications. ALT is not useful in evaluating hepatic disease in the horse and cow because of relatively low levels of ALT in hepatocytes relative to muscle (Lassen, 2004). Alkaline phosphatase (ALP) activity is found in the cell membranes of many tissues including liver, bone, intestine, kidney, and placenta. Serum ALP is primarily of hepatic origin in the dog, cat, and horse, though bone isoenzyme can also be detected. Also dogs can have measurable levels of corticosteroid induced ALP in serum when the animal is subjected to increased levels of endogenous corticosteroids or with administration of glucocorticoids (Stockham and Scott, 2002). Hepatic isoenzymes have a longer half-life (days) than intestinal, renal, or placental (minutes) isoenzymes. Cholestasis induces hepatic ALP. An increase in serum ALP can precede hyperbilirubinemia. Corticosteroids, phenobarbital, dieldrin, and other compounds may induce hepatic ALP. Increased osteoblastic activity in hyperparathyroidism, bone healing, or osteosarcoma may elevate ALP. Horses and ruminants have wide reference intervals for ALP; therefore, this enzyme has decreased sensitivity for the detection of cholestatic disease in these animals (Lassen, 2004; Stockham and Scott, 2002). Gamma glutamyltransferase (GGT) is found in many cells, but specifically the renal tubular epithelium, canalicular surfaces of hepatocytes, pancreas, and bile duct epithelium. The mammary gland is another source of GGT in cattle, sheep, and dogs, which can result in high serum levels in neonates of these species after nursing (Lassen, 2004). In renal disease, GGT is excreted in the urine (see nephrotoxicity). Serum GGT is generally of hepatic origin and is elevated by cholestasis. GGT has narrower reference intervals than ALP in horses and ruminants, which makes it more useful for detecting cholestatic disease in these species (Lassen, 2004; Stockham and Scott, 2002). Increased serum GGT activity proved to be a sensitive and long-lived indicator of liver insult in cattle exposed to moldy hay (Casteel et al., 1995). Like alkaline phosphatase, GGT appears in serum as a result of increased synthesis, rather than as a result of leakage from cells (Pearson, 1990). In dogs, the increase of GGT tends to parallel that of ALP. A high activity of sorbitol dehydrogenase (SDH) is found in hepatocellular cytoplasm of dogs, cats, horses, and ruminants (Lassen, 2004). The plasma half-life of this enzyme is very short, and serum activities may return to normal within 5 days of hepatocellular insult. Though this enzyme is more specific for hepatocellular damage than other enzymes in the horse and ruminant, the relatively low in vitro stability makes it less commonly used in the dog and cat compared to ALT. Lactate dehydrogenase (LDH) is a tetrameric enzyme with five isoenzymes that catalyze the reversible conversion of L-lactate to pyruvate in all tissues. All LDH isoenzymes are found in varying concentrations in all tissues. LDH1 is the principal isoenzyme in cardiac muscle and kidney of most species. It is also found in the liver of cattle and sheep. Unlike the other isoenzymes, it is heat stable at 65°C for 30 min. LDH5 is the principal isoenzyme in skeletal muscle and erythrocytes. Serum LDH activity is tissue nonspecific; however, necroses of muscle, liver, and hemolysis are the major causes sources of elevations. Isoenzyme analysis would improve the specificity of LDH analysis for hepatocellular damage, but this is not commonly performed in most veterinary laboratories (Lassen, 2004; Stockham and Scott, 2002). Bilirubin is derived from destruction of damaged or senescent erythrocytes by macrophages of the spleen, liver, and bone marrow. It is noteworthy that bilirubin at physiological levels is an antioxidant (Stocker et al., 1987). Bilirubin is transported in plasma bound to proteins (albumin, globulin). Hepatic uptake and glucuronide conjugation render it water soluble. Conjugated bilirubin is secreted into bile canaliculi and transported to the intestine where the majority is transformed into urobilinogen by intestinal flora and excreted. Direct diazo assay for bilirubin detects conjugated bilirubin. Total bilirubin is measured after addition of alcohol, which allows additional color development. Unconjugated bilirubin is determined by the difference in direct and total bilirubin. Cholestasis results in conjugated hyperbilirubinemia. Bilirubinuria may occur as a result of “regurgitation” of conjugated bilirubin. Increased ALP or GGT can precede hyperbilirubinemia in most species. Hemolysis may result in unconjugated hyperbilirubinemia and elevations of LDH5. Sulfobromophthalein (BSP) injected intravenously is removed rapidly from the blood, conjugated by hepatocytes, and excreted in bile. The rate of hepatic blood flow, functional hepatic mass, and patency of the biliary system affect the hepatic clearance of this compound. Altered blood flow secondary to cardiotoxicity discussed later may increase BSP retention. The use of this test is limited as BSP is no longer commercially available, and similar information can be obtained by assessment of bile acids and total bilirubin (Stockham and Scott, 2002). Bile acids are secreted from the liver into the bile and are subsequently reabsorbed in the intestine. The portal blood flow delivers the bile acids to the liver where, in healthy animals, they are efficiently cleared by the hepatocytes. Increased serum bile acids in fasting animals are a result of decreased biliary excretion or decreased clearance by hepatocytes. Increased serum bile acids are highly sensitive for hepatobiliary dysfunction; however, there are many diseases that may cause hepatobiliary dysfunction (Stockham and Scott, 2002). Bile acids may be useful for detecting hepatic dysfunction in instances where enzyme levels or clinical signs are equivocal (Lassen, 2004). Acute toxic hepatic necrosis increases serum bile acids (cholic and chenodeoxycholic) in the dog, horse, sheep, and cow (Bain, 2003). Some studies have recommended tests for urine bile acids as a possible alternative to serum bile acids to detect hepatic dysfunction in the dog and cat (Balkman et al., 2003; Trainor et al., 2003). Ammonia is generated by microbial activity and digestion of protein within the intestinal tract. It is absorbed from the intestine and transported to the liver by the portal venous system where it is converted to urea by the healthy liver. Elevations of plasma ammonia during fasting or following ammonia challenge suggest reduction in clearance from the blood, frequently resulting from a decrease in functional hepatic mass. Urea toxicosis in cattle and consumption of ammoniated forages by cattle can result in high plasma ammonia levels because of increased production and consumption of ammonia, respectively (Stockham and Scott, 2002). Severe hepatic insufficiency may result in hypoproteinemia (Kaneko, 1997a) with reduction of plasma oncotic pressure that promotes tissue edema and effusions that mimic the effects of cardiotoxins (Table 27-3). The clinical signs of acute submassive or massive hepatic necrosis may include anorexia, vomiting, icterus, hepatic encephalopathy, disseminated intravascular coagulopathy, edema, and effusions. Surprisingly, there may be few or no clinical signs in some cases. The activity of ALT and SDH with short half-lives may be elevated but often fall rapidly. Inducible enzymes such as ALP and GGT may increase gradually. All enzymes may return to normal in the presence of chronic severe liver disease. Hyperbilirubinemia may follow if lesions progress to chronicity and fibrosis. Chronic hepatotoxicity has sequelae for most organ systems, but especially the nervous (hepatic encephalopathy), integumentary (secondary photosensitization in herbivores), and cardiovascular systems. Cardiotoxins and pneumotoxins may produce enzyme elevations suggestive of hepatic or renal disease as a result of ischemia/hypoxia. Ingestion of toxic plants (Table 27-1) tends to be more common in herbivores than carnivores; however, nonherbivorous species are often susceptible if they are willing to ingest them. In addition to hepatotoxins, the blue-green algae (actually classified as cyanobacteria), which contaminate water (Carmichael, 1994), possess neurotoxins that may induce sudden death that precedes alterations of clinical biochemistry and morphological changes. However, one report documents marked elevations of ALT and AST in a dog 12 h post ingestion of a blue-green algae (DeVries et al., 1993). Therapeutic drugs can also occasionally have hepatotoxic effects in animals. Nonsteroidal anti-inflammatory drugs, barbiturates, antineoplastic agents, and antiparasitic compounds have all been found to have hepatotoxic effects (Kristal et al., 2004; Macphail et al., 1998; Roder, 2003). Carprofen, a nonsteroidal anti-inflammatory drug, has been reported to cause acute hepatocellular necrosis and cholestasis in some dogs. This adverse reaction is associated with marked increases in serum ALT, AST, ALP, and total bilirubin (Macphail et al., 1998). The antineoplastic drug 1-(2-chloroethyl)-3-cyclohexyl-1-nitrosourea (CCNU) is reported to occasionally cause hepatotoxicity in dogs with increases in ALT, AST, ALP, GGT, and bilirubin noted in the affected dogs (Kristal et al., 2004). Hepatotoxic plants of the order Compositae include Asaemia axillaris, Athanasia trifurcata, Helichrysum blandowskianum, Lasiospermum bipinatum, and Xanthium spp. The toxin in these plants has been identified as carboxyatractyloside. Profound hypoglycemia is reported as a finding in carboxyatractyloside poisoned animals (Barr and Reagor, 2001). Cycadales contain methylazoxymethanol, which is converted by hepatic microsomal activity to potent alkylating agents. A report of dogs ingesting cycads indicates bilirubin, ALT, and ALP are most commonly elevated values on the chemistry panel (Albretsen et al., 1998). Hepatotoxic plants of the order Myoporaceae include Myosporum deserti, M. acuminatum, M. insulare, M. tetramdum, and M. laetum. These plants contain furanosesquiterpenoid oils, the best characterized of which is ngaione. Chronic intoxication of swine with fumonisins, mycotoxins produced by Fusarium moniliforme, is associated with elevations of serum total bilirubin, ALP, AST, GGT, and cholesterol (Casteel et al., 1994). In addition, fumonisins inhibit N-acetyl transferase resulting in loss of complexsphingolipids and accumulation of sphinganine and sphingosine in tissues and serum (Riley et al., 1993). Iron toxicity from nutritional supplements may produce hepatic necrosis in foals (Acland et al., 1984) and pigs (Kelly, 1993). Excessive dietary copper may be associated with elevation of GGT in goats (Solaiman et al., 2001) and llamas (Weaver et al., 1999). Hepatotoxic pyrrolizidine alkaloids are found in the plant species Amsinckia intermedia, Crotolaria spp., Cynoglossum officinale, Echium plantagineum, Heliotropium europeaum, Senecio jacobea, S. vulgaris, and S. longilobus. Acute intoxications with these plants result in large increases in AST, ALP, GGT, and SDH (Stegelmeier, 2003). Chronic exposure to small amounts of the plant is more difficult to detect, though serum GGT levels have been suggested as a screening test for subclinical hepatic damage in horses exposed to Crotolaria spp. (Curran et al., 1996). Hepatotoxic solanaceae include Cestrum parqui, C. laevigatum, and C. aurantiacum. The toxin in these plants has been identified as atractyloside. Sawfly larvae (Lophyrotoma interruptus and Arge pullata) infesting eucalyptus and birch trees in Australia and Denmark, respectively, have been reported to induce hepatic necrosis in cattle, sheep, and goats browsing foliage. Terminalia oblongata, the yellowwood tree of Australia, contains hepatotoxic tannins and pucicalagin, which induces acute hepatic necrosis (Kelly, 1993). Acute nephrotoxicity (Table 27-2) may initially induce polyuria that is followed by oliguria or anuria. Nephrotoxins affecting approximately 66% of the nephrons will result in inability to concentrate urine to a specific gravity greater than 1.030 in the dog, 1.035 in the cat, and 1.025 in the horse and cow. Chronic toxicity may result in isosthenuria (constant urine osmolality in the range of glomerular filtrate, 1.008 to 1.012). The majority of urea is synthesized in the liver from ammonia formed by protein catabolism or intestinal absorption. Urea enters the vascular system and is distributed throughout the total body water compartment by passive diffusion. The urea concentration of blood and glomerular filtrate is approximately equal. Urea passively diffuses from the tubular lumen back to the blood. Urine flow rate is inversely related to urea reabsorption. Gastrointestinal secretion is inconsequential in monogastrics; however, in ruminants up to 90% of urea in glomerular filtrate may be reabsorbed and enter the rumen via saliva for utilization in amino acid synthesis. Renal disease also results in elevation of serum creatinine. The majority of serum creatinine originates from the endogenous conversion of phosphocreatine in muscle, which occurs at a relatively constant rate. Creatinine is not reutilized. The creatine pool is modified by conditioning and muscle disease. Creatinine also is distributed throughout the compartment of total body water. It diffuses more slowly than urea, however, and is not reabsorbed within the tubules after leaving as glomerular filtrate. Creatinine concentration is not affected significantly by diet, protein catabolism, or urinary flow. Reduced renal perfusion affects BUN and creatinine similarly (Finco, 1997). Elevations of BUN and creatinine are not proportional in renal disease of ruminants because of reutilization of urea by the rumen. Ingestion of the plant Nolletia gariepina has been reported to cause renal failure in ruminants with a measurable increase in urinary GGT along with azotemia (Meintjes et al., 2005). Hyperkalemia may occur in renal failure with oliguria or anuria and acidosis. Hypercalcemia is common in equines as a result of decreased renal clearance of calcium. Hypocalcemia is more common in dogs, cats, and cattle with chronic renal disease. Cattle also tend to have hypokalemia, hyponatremia, and hypochloridemia with renal disease. Mild to moderate increases of amylase and lipase may also be seen in dogs with renal disease as these enzymes are inactivated in the kidney (Stockham and Scott, 2002). Proteinuria in the absence of occult blood and cellular sediment suggests renal disease. Glomerular lesions typically result in high protein levels in which albumin is the major constituent. Acute tubular damage observed with many nephrotoxins generally results in lower protein levels containing higher levels of smaller globulins and some albumin (Stockham and Scott, 2002). Analysis of enzymes in the urine can potentially determine the primary site of renal damage because of the characteristic localization of enzymes within the nephron. Increases in the brush border enzymes, GGT and ALP, in the urine have been associated with renal proximal tubular damage in dogs, whereas increases in N-acetyl-beta-D-glucosaminidase have been observed in the early stage of renal papillary necrosis. However, evaluation of several enzymes at multiple time points is needed to compensate for normal enzyme variation and to identify potential anatomic site selectivity of the toxin (Clemo, 1998). Hypoproteinemia secondary to chronic urinary loss (Kaneko, 1997a) promotes tissue edema and effusions that may mimic cardiotoxicity and hepatotoxicity, as discussed previously. Bilirubin is considered to be mildly nephrotoxic. Bilirubinuria may occur because of “regurgitation” of conjugated bilirubin resulting from cholestatic hepatotoxins. Myoglobin is also nephrotoxic. Myoglobinuria may occur with toxic necrosis of skeletal and cardiac muscle. Hemoglobin appears to be nephrotoxic in the presence of concurrent dehydration or hypovolemia. Hemoglobinuria may occur with hemolytic toxins. Dehydration exacerbates the nephrotoxicity of many agents, especially antibiotics in all species and nonsteroidal anti-inflammatory drugs (phenylbutazone) in the horse. Nephrotoxic antibiotics include the aminoglycosides (amikacin, gentamicin, kanamycin, neomycin, streptomycin, and tobramycin), amphotericin B, cephalosporins, polymixins, sulfonamides, and tetracyclines (Maxie, 1993). Elevation of GGT in urine is a sensitive indicator of aminoglycoside toxicity (Gossett et al., 1987). Hypercalcemia and hyperphosphatemia may result in nephrocalcinosis following iatrogenic hypervitaminosis D or ingestion of cholecalciferol rodenticide (Fooshee and Forrester, 1990) by any species. Ingestion of the toxic plants containing vitamin D-like analogues including Cestrum diurnum, Dactylis glomerata, some Solanum spp., and Trisetum flavescens by herbivores also may produce hypercalcemia with calcification of soft tissues including the kidney. Nephrotoxic metals include arsenic, bismuth, cadmium, lead, mercury, and thallium (Maxie, 1993). Plants containing toxic concentrations of soluble oxalates include the species Amaranthus retroflexus (pigweed), Halogeton glomeratus, Oxalis spp., Rheum rhaponticum (rhubarb), and Sarcobatus vermiculatus (greasewood). Intoxication with ethylene glycol from antifreeze is one of the more common accidental or malicious poisonings encountered in dogs and cats. Birefringent hippurate and oxalate crystals may be observed in urine sediments (Kramer et al., 1984). An increased anion gap and decreased blood bicarbonate can be observed in animals with ethylene glycol intoxication (Dalefield, 2003). Blood calcium is lowered in animals intoxicated with oxalate containing plants that also have low calcium content. Blood calcium is also decreased in animals with ethylene glycol toxicosis (Stockham and Scott, 2002). Nephrotoxic pyrrolizidine alkaloids include the plant species listed under hepatotoxicity. Trees of the genus Quercus (oaks) and Terminalia oblongata (yellow-wood tree) contain tannins that induce acute tubular necrosis when leaves, buds, or acorns are ingested. Amaranthus retroflexus (pigweed), via an unidentified toxic principle, also induces similar renal disease in cattle (Casteel et al., 1994) and pigs (Osweiler et al., 1969) in the absence of oxalate nephrosis. At postmortem examination, there were consistent elevations of urea and creatinine concentrations in ocular fluid and serum. The clinical signs of weakness, dysmetria, and incoordination suggest not only the possibility of neurological disease but also skeletal muscular or cardiovascular disease. Acute toxicity of skeletal or cardiac muscle (Table 27-3) can be detected by elevations in serum creatine kinase (CK) (Cardinet, 1997). This dimeric enzyme catalyzes the reversible reaction, phosphocreatine + ADP < = > creatine + ATP, and has three isoenzyme types: CK1, CK2, and CK3. CK1 is found in brain, peripheral nerves, cerebrospinal fluid, and viscera, but it is not found in serum during neurological disease. CK2 is found in cardiac muscle and minute amounts in skeletal muscle. CK3 is found in cardiac and skeletal muscle. CK plasma half-life is short and is considered to be specific for muscle when hemolysis, elevated bilirubin, muscle fluid contamination during venipuncture, and dilution of CK inhibitors during sample processing can be excluded. When injury is not progressive, CK elevations maximize within 6 to 12 h and return to normal within 24 to 48 h. Continuing necrosis can result in persistent elevation. Cardiac troponin T and I have been demonstrated to be sensitive and specific biomarkers of cardiac injury in dogs and laboratory animals (O’Brien, 2006; O’Brien et al., 2006). Cardiac troponins have been demonstrated to correlated with myocardial injury in the dog, cat, and horse and may provide a more specific antemortem method of detecting myocardial compromise secondary to toxicosis in these species (Herndon, 2002; Oyama and Sisson, 2004; Schwarzald et al., 2003). As discussed previously, LDH5 is the principal isoenzyme in skeletal muscle and erythrocytes. LDH activity is tissue nonspecific, but necroses of muscle, liver, and hemolysis are the major sources for elevations of serum activity. Necrosis of skeletal muscle may result in release of myoglobin and potassium resulting in myoglobinemia and hyperkalemia. Myoglobinuria, detectable by urinalysis, may induce secondary nephrotoxicity.
I. INTRODUCTION
II. HEPATOTOXICITY
III. NEPHROTOXICITY
IV. TOXINS AFFECTING SKELETAL AND CARDIAC MUSCLE
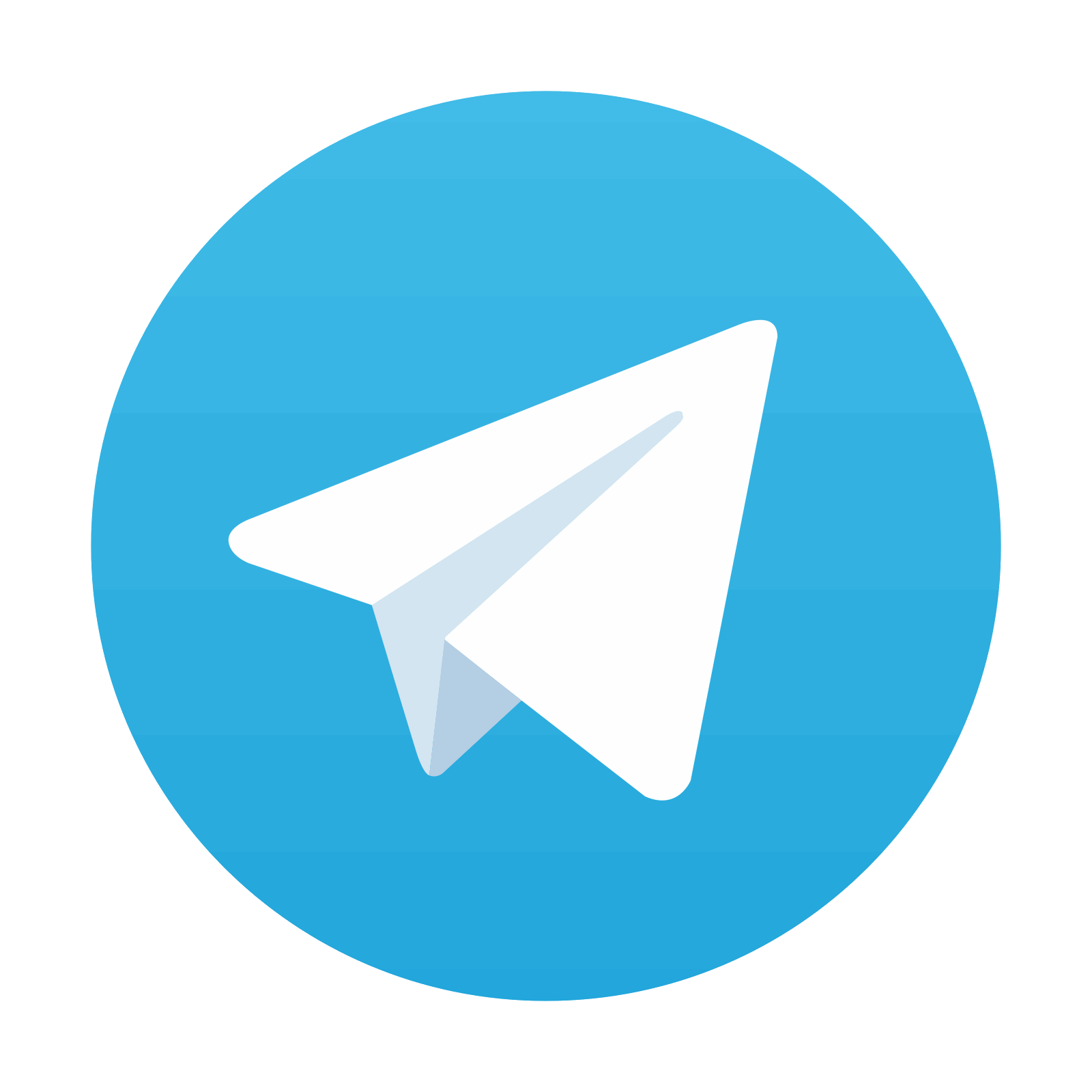
Stay updated, free articles. Join our Telegram channel

Full access? Get Clinical Tree
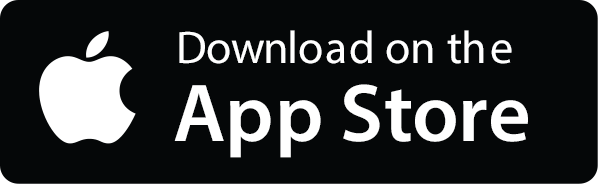
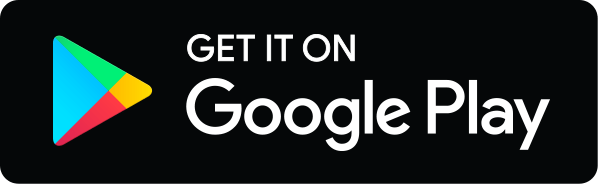