Chapter 3
Clinical Aspects of Evolution in Reptile Medicine
Evolution
A definition is first necessary (Box 3-1). A monophyletic group is defined as a group that contains a common ancestor and all descendants. In comparative medicine, understanding what constitutes a monophyletic group is needed to understand relationships and to choose appropriate models. A group that is not monophyletic is called paraphyletic. Paraphyletic groups may not share a common ancestor or may exclude some descendants of the common ancestor. It is illogical to predict that paraphyletic groups would share characteristics that are not in nonmembers. Primates constitute a monophyletic group; when the primates except for humans are referred to, the term nonhuman primates is generally used. This qualification in the term helps the reader understand that a paraphyletic group is being referred to.
Evolution of Tetrapods
When the evolution of the tetrapods (terrestrial vertebrates) is examined, both the fossil record and the even stronger evidence from nucleic acid sequence phylogeny analyses are in agreement on relationships.1 The earliest divergence among the tetrapods is between the amphibians and the amniotes (Figure 3-1). The amniotes consist of the reptiles (including birds) and the mammals. The amnion was a major evolutionary advance, enabling the amniotes to have a completely terrestrial life cycle without the need to return to water for reproduction. This is also the first place in tetrapod evolution where common knowledge gives us fundamental errors. Herpetology is the study of nonavian reptiles and amphibians; it does not include mammals and birds. However, nonavian reptiles are far more fundamentally similar to birds and mammals than they are to amphibians, so it is not reasonable to expect nonavian reptile biology to resemble amphibian biology more closely than avian biology or mammalian biology. This is most medically apparent when looking at skin function, respiration, or reproduction.
Amniotes
The amniotes are then further divided into mammals and sauropsids (see Figure 3-1, shown in green). Within the sauropsids, the first group to diverge is the squamate (lizards and snakes)/sphenodontid (tuatara) clade. After this, the testudines (turtles) diverged, and the last two major sauropsid groups to diverge were the crocodilians and the dinosaurs, collectively known as the archosaurs. The recognition of dinosaurs as reptiles is widespread in our culture. However, what is not generally recognized is that dinosaurs are not extinct and that birds are the only surviving group of dinosaurs. This is supported both by the fossil record and by sequence data.2 Part of this failure may be due to an erroneous picture of nonavian dinosaurs: they shared a number of traits with birds, including feathers,3 and there is evidence they were warm blooded.4 Nevertheless, although birds themselves constitute a monophyletic group, if birds are not considered to be part of the reptile group, then reptiles are not a monophyletic group. What we really mean by reptiles is sauropsids, and birds are a group of reptiles. The term nonavian reptile should be used if excluding birds from the reptiles, an awkward term indicating a logically awkward paraphyletic group.
Crocodilians and Birds
Crocodilians and birds share a number of medically relevant similarities. In a mammal, a persistent right aortic arch is a developmental problem that obstructs the esophagus, and the left aortic arch is the main outflow for oxygenated blood from the heart. In archosaurs, the right aortic arch is the major outflow.5 Unlike birds, crocodilians have retained their left aortic arch, but the function it serves is primarily to transport hypercapneic blood to the stomach, where it is used to create an extraordinarily low gastric pH.6 Indeed, crocodilians are one of the few groups of animals studied in which oral fluoroquinolones do not result in good blood levels, and it is possible that breakdown in the extremely acidic stomach may play a role in this.7,8
Both birds and crocodilians have four-chambered hearts. A four-chambered heart is not needed for separation of right and left blood flow, which is accomplished in nonarchosaur reptiles that lack a ventricular septum.9 The major advantage of a ventricular septum is facilitation of pressure differentials between the left and right sides of the heart, as may be needed in an animal with a high metabolic rate. Ventricular septa evolved separately in archosaurs and mammals; both groups have warm-blooded members.
Crocodilians and birds also both have in common a respiratory system with unidirectional rather than tidal air flow, a much more efficient design than the mammalian lung.10 An efficient respiratory system is also needed for a higher metabolic rate.
Ancestors of modern crocodilians from shortly after the divergence from dinosaurs were morphologically consistent with long-legged active terrestrial predators.11 It is plausible that ancestral archosaurs were warm-blooded animals and that modern crocodilians lost homeothermy, which would be an evolutionarily disadvantageous trait for aquatic ambush predators.
Lizards
Another prevalent error is the concept of lizards as a group distinct from snakes. In squamate evolution, the earliest divergence is the geckos, followed by the divergence of the skinks, night lizards, and plated lizards (Figure 3-2). The next groups to branch off were the teiids, lacertids, and amphisbaenids, and the remaining group, containing snakes, iguanids, agamids, chameleons, monitors, helodermatids, and anguids, is known collectively as the Toxicofera (see blue on Figure 3-2), named for the commonality of the presence of venom glands. There is a very nice image of the histologic features of Bearded Dragon venom glands in Fry et al.12 Snakes diverge in the middle of the squamates, and if snakes are removed, then lizards are not a monophyletic group. Snakes are a group of lizards, and a Cornsnake is a better model for a Bearded Dragon than a Leopard Gecko.
Caring for Captive Animals
It is critical, when caring for captive animals, to consider environments in which they have evolved. Eons of selective pressure have resulted in reptiles that have adapted to specific diets, habitats, and threats, and disease may result when captive conditions differ. Periodontal disease is common in lizards with acrodont dentition (agamids and chameleons) in captivity but not in the wild.13 Infiltrative lipomas appear to be common in obese captive Cornsnakes.14 Significant rostral trauma is common in water dragons and basilisks kept in glass enclosures without sufficient cover.
Microbes
Microbes are essential for all vertebrate life, for functions including digestion, nutrition, and defense. In veterinary school, we have all been taught Koch’s postulates as criteria for establishing a microbe as a pathogen. Although Koch’s postulates have their use, they frequently result in a false dichotomous understanding of microbes as either pathogenic or nonpathogenic. There is no such thing as a microbe that is always either a pathogen or a nonpathogen. There have been many asymptomatic human Ebola virus infections, and people have died of septicemia due to Lactobacillus acidophilus.15,16 A microbe does not “want” to cause disease or not cause disease. All life on earth has been selected for billions of years to reproduce successfully, and this is all that matters from an evolutionary standpoint. If pathogenic traits provide an evolutionary advantage in a given situation, they will be selected for. If they provide a disadvantage, they will be selected against.
A number of important selective pressures affect microbes in a vertebrate host, including nutrient availability, temperature, competition with other microbes, the need to transfer to a new host, and the host immune system. A vertebrate host is a nutrient-rich environment. However, some nutrients may be sequestered; significant resources are spent by the host synthesizing transferrin, lactoferrin, and ferritin to make it unavailable. Many bacterial virulence pathways have evolved to access this sequestered iron.17,18
Homeothermic vertebrates also provide a highly temperature-controlled environment, whereas poikilothermic hosts require the ability to survive at different temperatures. Because of this, infectious disease manifestation may be highly temperature-dependent in poikilotherms.19–21 This adds an extra dimension to disease ecology, especially with latent or persistent infections. Further investigation of the role of temperature in disease manifestation in poikilotherms is strongly indicated, especially with populations of many reptile species critically declining and likely to be affected by anthropogenic climate change.22
Parasites often adapt to a balance with their hosts. Many parasites tend to have slower generation times compared with viruses or bacteria, which makes rapid reproduction and moving on to a new host less of a viable strategy. The costs of parasites to their definitive hosts are relatively minimal in many cases because it is advantageous to parasites to preserve their habitat. Bullfrog tadpoles carrying the pinworm Gyrinicola batrachiensis have better feed conversion and metamorphose earlier than uninfested controls, rendering the relationship mutualistic rather than parasitic.23 However, for parasites with indirect life cycles, causing disease in an intermediate host may be advantageous. If a rodent carrying pentastomid larvae is debilitated, it is more likely to be eaten by a snake, completing the life cycle. This may also result in greater disease in accidental hosts.24 Some parasites do survive well in the environment; this reduces the selective pressure not to harm the host. Parasites that survive well in the environment are much more likely to cause significant disease; perhaps the best known examples of this in reptiles are Rhabdias sp., which cause pulmonary disease in squamates that may be fatal.25
Most fungi also survive well in the environment, resulting in little selective pressure to keep their host alive. They compete significantly with bacteria for the same niches; this has resulted in the production of antibacterial compounds by fungi and antifungal agents by bacteria. The fungi are some of the closest relatives of animals; fungi, choanoflagellates, and metazoa (multicelled animals) form a clade known as the Opisthokonta.26 An animal is much more closely related to a mushroom than it is to an oak tree. Antimicrobial drugs generally exploit differences in chemistry and metabolism between pathogen and host. Because fungi and vertebrate hosts diverged more recently, there are fewer differences to exploit, and antifungal drugs tend to have narrower therapeutic indices and use a smaller subset of mechanisms.
Bacteria constitute a large portion of the host ecosystem. There are far more bacterial cells in a normal vertebrate than there are vertebrate cells. Traditional approaches to examining bacterial diversity have depended on culture; this is a poor way of assaying diversity. Culture-independent methods, such as 16S polymerase chain reaction (PCR) and cloning, or high-throughput sequencing methods, have revealed that standard culture-based methods will detect between 1% and 10% of bacterial species present in most ecologic niches. As an understanding of further diversity has arisen, it becomes clearer that a vertebrate is a complex ecosystem.27 This system may be very dynamic. The gut flora of Burmese Pythons changes significantly in response to feeding.28 Postprandially, bacteria in the phylum Firmicutes (“classic” gram-positive bacteria, containing organisms such as Clostridium, Lactobacillus, and Peptostreptococcus) increase dramatically, while those in the phylum Bacteroidetes (primarily containing anaerobic gram-negative organisms such as Bacteroides and Prevotella) make up a greater percentage of the fewer species present after fasting. The gut flora did not significantly share species with prey mice, with the exception of the temporary postprandial establishment of Lactobacillus sp.
Ecologic disturbance may have significant negative impacts on many aspects of health. Damage to healthy gut flora by antibiotic use provides opportunity for invasive species; recent treatment with antibiotics markedly increases host susceptibility to Salmonella.29 A 5-day course of ciprofloxacin will change human gut flora diversity and composition for several weeks, and the original composition may never reestablish.30 In many ways, the use of broad-spectrum antibiotics for a bacterial infection in a vertebrate is analogous to starting a forest fire to get rid of coyotes. The ideal treatment for a bacterial pathogen would be as narrow-spectrum as possible, minimally disturbing the rest of the host ecosystem. Isoniazid, which targets only Mycobacterium tuberculosis and a few very closely related species and does not significantly affect many other Mycobacterium sp., is an excellent example. Unfortunately, current market forces have resulted in pharmaceutical companies developing antibiotics with as broad a spectrum as possible, and narrow spectrum antibiotics are often not put through further development and clinical trials.
Antibiotic use without consideration of microbial ecology and evolution rapidly leads to failure. Back in the 1980s, gentamicin was promoted for eradication of Salmonella from turtles.31 Over the next few years, the Salmonella isolates from farmed turtles acquired a high rate of gentamicin resistance32 and therefore posed a greater risk to human health than they had previously. Several studies have suggested that wild turtles may have a lower carriage rate for Salmonella.33,34 The only realistic way to reduce the risk of Salmonella in farmed turtles over the long term is to alter the ecologic niche that it inhabits. Keeping farmed animals in high population densities increases contact rates, pathogen loads, and stress, lowering barriers to transmission. Increased ease of transmission reduces the selective pressure to keep the host alive and healthy. Reducing the risk of Salmonella in hatchling turtles probably means farming at lower densities and eliminating high-density turtle farming.35
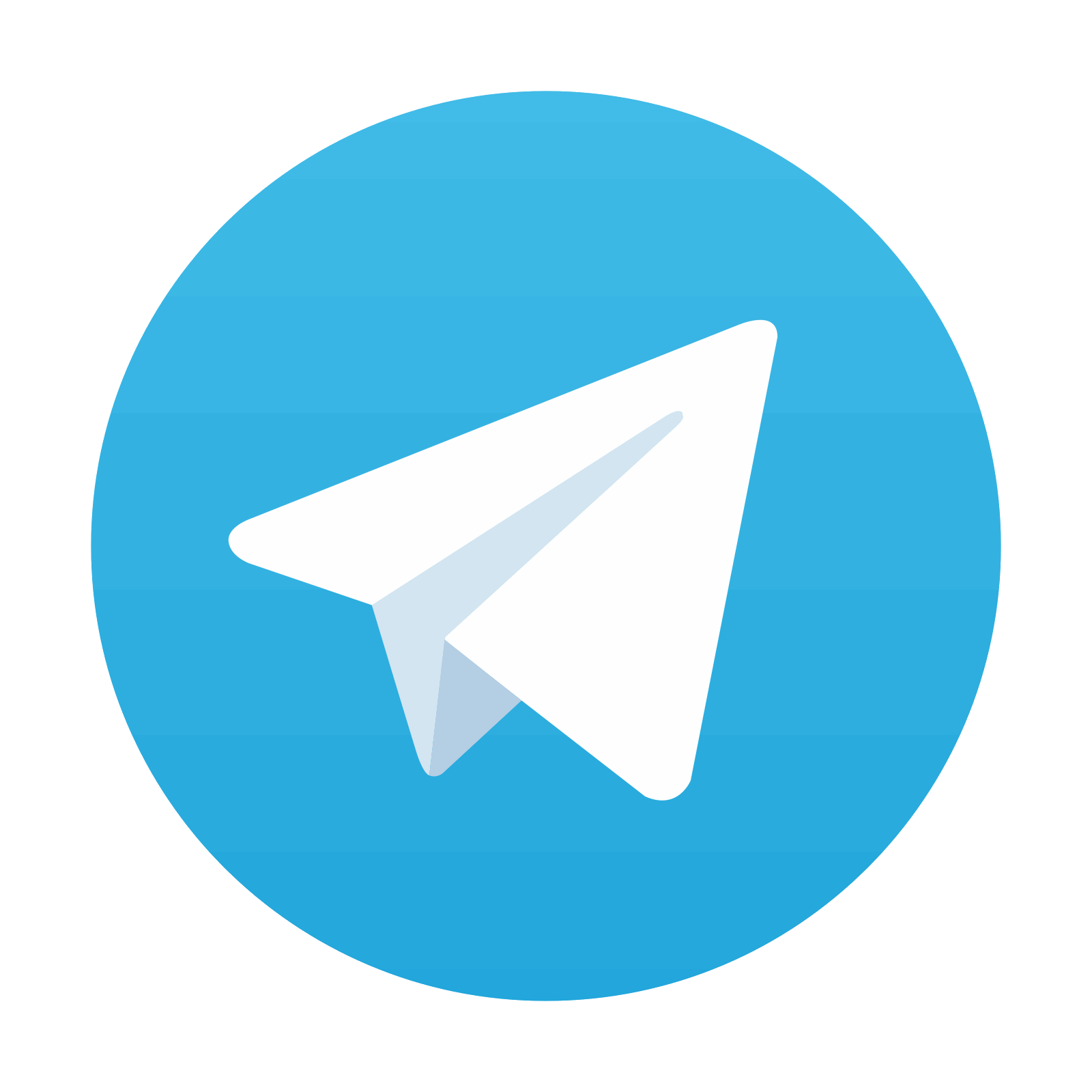
Stay updated, free articles. Join our Telegram channel

Full access? Get Clinical Tree
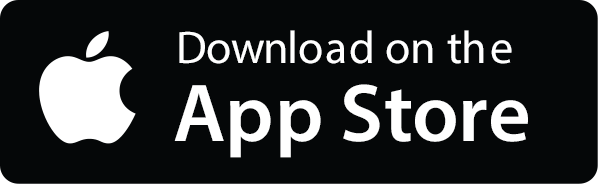
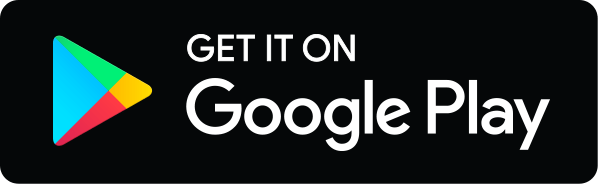