Chapter 7
Therapeutics
Diagnosis
Similar to class Mammalia, class Reptilia includes a diverse group of species, each with a unique pharmacologic response to every chemotherapeutic agent. As a result, therapeutic safety and efficacy differ among species. Unlike mammals, conscious reptiles are ectothermic, so physiologic and biochemical processes are strongly influenced by body temperature.1 Reasonable assumptions about each individual species’ metabolism and immune response can therefore only be made under certain conditions. In most cases, the body temperature of a reptile patient must match that of the subjects in a given pharmacokinetic study for a treatment plan to be designed, although pharmacokinetics and pharmacodynamics do not necessarily differ at different temperatures.2–5 Species-specific pharmacokinetics, pharmacodynamics, therapeutic efficacy, and environmental requirements must be known before administration of any drug can be considered. This is not only to predict absorption, distribution, metabolism, excretion, and the therapeutic window but also to meet the environmental needs of a reptile so that an appropriate immune response and healing can occur.4,6–8 It remains true that, even though many pharmacokinetic studies have been published in reptiles, not all drugs have been studied in all of the species presented to reptile veterinarians. Published doses are available for many drugs, and these may have been selected empirically, may have been calculated with the use of allometric or other scaling technique, or may be from published pharmacokinetic research data collected from healthy animals under laboratory conditions. It is incumbent on the clinician to evaluate the probability that a published dose is likely to be effective in a particular species under the individual circumstances of a clinical case. Dosage, dosing interval, and administration route must be evaluated in light of the individual circumstances of each case. Consult up-to-date, peer-reviewed literature to identify species and their environmental needs, optimal body temperature, and the pharmacokinetics and pharmacodynamics of the drug in that species.9 Consider how physical and physiologic changes caused by disease might affect absorption, distribution, metabolism, excretion, side effects, and required therapeutic levels of a drug.
Managed thermoregulation, hydration, and nutrition are paramount for safe, effective drug therapy. Thermal options and gradients must be provided for conscious, active reptiles to behaviorally thermoregulate, and body temperature should be monitored during therapy. In cases of weakness and decreased activity, the patient’s thermoregulation should be actively managed as a part of the therapeutic plan. Dehydrated, anorexic reptiles may not absorb, distribute, metabolize, or eliminate chemotherapeutic agents in the same manner as the healthy animals tested under controlled conditions in pharmacologic research, so nursing care also must include management of fluids, electrolytes, and nutrition.4,5,10
Few therapeutic agents are approved for use in reptiles by the United States Food and Drug Administration (FDA), so almost all drug use is “extralabel.” In the United States, the Animal Medicinal Drug Use Clarification Act of 1994 (AMDUCA) and the Minor Use and Minor Species Animal Health Act of 2004 (MUMS) serve as guides for extralabel drug use. Prescription drugs may be used to treat disease in reptiles under the supervision of a veterinarian if (1) they are approved by the FDA for any purpose in any species, (2) a valid veterinarian–client–patient relationship exists, and (3) the FDA has not specifically prohibited extralabel use of the drug. Certain medical record and prescription label requirements must be followed to meet the obligations of veterinary licensure.11
Administration and Dosing
Dosage and route of administration are determined by the chemical, pharmacokinetic, and pharmacodynamic properties of each agent in each species. Much work has been done to understand the mechanism of action, therapeutic window, absorption, distribution, metabolism, and excretion variables of many drugs in many species of reptiles, although many questions remain unanswered and informed, empiric dosing is still used in many cases.4,9 Veterinarians administer therapeutic agents to reptiles via oral, enteral via gavage, subcutaneous (SC), intramuscular (IM), intravenous (IV), intracoelomic, intratracheal, intrapulmonary, intraosseous, intraperitoneal, intrathecal, and intracardiac routes, as well as via nebulization. These administration routes have been described.4
Parenteral administration has proven to be more reliable than the enteral route for many drugs in reptiles.4 Sufficient evidence exists to support administration of most parenteral drugs in the cranial half of the body when possible so that the first-pass effect for drugs that are eliminated via renal tubular excretion or hepatic metabolism is avoided. Venous blood from the caudal half of the body enters the caudal vena cava through either the renal portal system and peritubular capillaries or the hepatic portal system from the abdominal or mesenteric veins and through the hepatocellular parenchyma.12,13 Administration in the caudal half of the body has proven to be acceptable for a few specific drugs and should be considered for those drugs in circumstances when the cranial half is not available for injections.5,14,15 Dosing adjustments must be made to account for the effects of renal tubular or hepatic metabolism as determined in these reports. Intracoelomic administration of fluids or systemic drugs cannot be recommended, except in rare, specific cases in which other routes are unsatisfactory. Absorption across coelomic membranes is difficult to assess in clinical patients and is not guaranteed, particularly in cases of abnormal blood proteins, coelomitis, or ascites. Accidental needle puncture or laceration of an organ and accidental deposition of the agent into the intestines, reproductive tract, or urinary bladder rather than the coelomic space have been observed and probably occur undetected in many cases; adverse effects have been reported.16 Some therapeutic challenges in reptiles can be overcome by novel approaches to drug administration, including osmotic pump, dermal patch, depot formulation, cardiac access port, and topical administration.
Osmotic Pump
Alzet osmotic pumps (Durect Corp., Cupertino, Calif.) (Figure 7-1) are implanted, miniature infusion pumps designed for continuous infusion of therapeutic agents to unrestrained animals. They are available with fixed delivery rates between 0.11 and 10 μL per hour and delivery durations between 1 day and 6 weeks. Marketed for laboratory animals, they range in size from 15 to 51 mm in length and 6 to 14 mm in diameter and have been used to deliver dozens of different therapeutic agents.17 The “pumps” operate via an osmotic pressure difference between the tissue environment and a salt-containing osmotic sleeve. The high osmolality of the salt sleeve causes water to diffuse into the pump, across the outer semipermeable membrane and into the salt sleeve, which puts pressure on a flexible (impermeable) internal reservoir that contains the therapeutic agent. This pressure causes the agent to be expelled via a flow moderator at a controlled, predetermined, continuous rate that is independent of the chemical properties of the agent being dispensed. Different dosing rates can be achieved by varying the concentration of the agent used to fill the pump reservoir. Virtually any commonly used parenteral drug can be delivered by these pumps because they are loaded by the user just before the time of implantation.
FIGURE 7-1 Alzet osmotic pumps (Durect Corp., Cupertino, Calif; model 1002 shown) can deliver medications continuously without the need for periodic injections. Although early clinical trials have not been successful, these do show promise for future drug delivery in reptiles that are difficult or dangerous to handle.
The use of osmotic pumps has been reported in Cornsnakes (Elaphe guttata), Mojave Rattlesnakes (Crotalus scutulatus), and Green Iguanas with amikacin, florfenicol, and gonadotropin-releasing hormone.18–20 It is important to consider the tissue environment of the implant (availability of free water) and the pharmacokinetic and pharmacodynamic properties of the drug to be administered because some drugs, including amikacin, may be safer and more effective when administered in pulses rather than continuously.21 In addition, substantial local necrosis and death occurred in five of six Mojave Rattlesnakes that were given florfenicol via subcutaneously implanted osmotic pumps.20 In spite of disappointing results in recently published studies, further investigation using different implantation sites, other drugs, and other species should be considered. Implantable, bioresorbable devices also deserve consideration, particularly for use in treatment of dangerous and venomous animals in which multiple surgical procedures are impractical.22
Transdermal Patch
Fentanyl, scopolamine, nicotine, buprenorphine, naloxone, nitroglycerine, ketoprofen, and many other drugs can be delivered by transdermal patch to humans and domestic mammals. Recently, one study tested fentanyl that was suspended in an ethanol and cellulose gel and enclosed by a plastic barrier with a permeable adhesive membrane impregnated with a loading dose of fentanyl on Prehensile-tailed Skinks (Corucia zebrata).23 The study showed that all of the skinks in the study obtained measurable plasma concentrations of fentanyl within 24 hours of patch application, and fentanyl concentrations reached the human analgesic range by 36 hours. The most important finding of this study is that the scaled skin of Prehensile-tailed Skinks does not present an insurmountable barrier to systemic absorption of fentanyl delivered via transdermal patch. It is possible that other drugs could be delivered via transdermal patch, and further investigations are warranted in Prehensile-tailed Skinks and other species.
Depot Formulations
Numerous chemotherapeutic agents are available in long-acting formulations. Examples of long-acting formulations that have been used in reptiles include oxytetracycline, doxycycline, ivermectin, and leuprolide acetate.24–27 Reports of pharmacokinetic studies of these formulations in reptiles provide support for the use of long-acting oxytetracycline for treatment of mycoplasmosis in American Alligators (Alligator mississippiensis) but not the other drugs.24–27
Vascular Access Ports
Vascular access ports are used to repeatedly collect blood samples or administer IV medications over time. They are particularly useful in pharmacokinetic studies and for cancer chemotherapy. Several authors have placed the rubber stopper from an evacuated blood collection vial into a round plastrostomy drilled ventral to the cardiac ventricle in tortoises. This has been used to collect multiple blood samples over time and can lead to myocardial fibrosis.28,29 Vascular access ports were placed into the common carotid artery to study blood gasses in seven Green Iguanas and remained functional in most cases for several weeks.30 A vascular access port (CompanionPort, Norfolk Medical Products Inc., Skokie, Ill) was placed into the ventral abdominal vein in a Green Iguana for lymphoma chemotherapy.31 This port was replaced after 28 days because of dermal necrosis over the port, and the second port was used for 6 months without a complication.31
Topical Administration
Numerous topical medications are used in reptiles, primarily to treat wounds or skin disease. Ivermectin, ophthalmic drugs, disinfectants, creams, ointments, and lavage solutions are all applied via the topical route. These applications are generally intended to deliver local therapy, and systemic uptake is usually an undesired side effect. Recently, several topical preparations for systemic therapy have been evaluated. Topical administration is ideal for antiparasitic agents because most traditional antiparasitic drugs are delivered via orogastric intubation, which can be difficult in some lizards and many chelonians. Two antiparasitic products have been evaluated for systemic uptake of topical preparations. A commercially available topical treatment for intestinal parasites containing imidacloprid and moxidectin (Advantage multi/Advocate, Bayer, Shawnee Mission, Kans) is available for domestic companion animals. The product was applied to the skin of Frilled Dragons (Chlamydosaurus kingii) and Bearded Dragons (Pogona vitticeps). After treatment, the feces no longer contained Kalicephalus spp. or oxyurid ova.32 The safety of this imidacloprid/moxidectin topical formulation was not evaluated, and pharmacokinetic research is needed. A commercially available topical preparation containing emodepside and praziquantel (Profender, Bayer HealthCare, Shawnee Mission, Kans) was tested for absorption, elimination time, and efficacy in several lizards, snakes, and chelonians.33,34 Both drugs were absorbed via the skin, except emodepside in a Red-eared Slider (Trachemys scripta elegans) that was returned to the water about 1 hour after application.33,34 Treatment with emodepside/praziquantel at doses much greater than those used in mammals was followed by a marked decrease in the number of nematode ova in feces within 48 hours. Similarly, no adverse effects were observed and fecal nematode egg counts decreased after treatment in a relatively large (n = 417), uncontrolled clinical trial of emodepside/praziquantel in species from four different reptilian families.35 The safety of this topical combination should be specifically studied because it holds great promise.
As opposed to these promising therapeutic innovations, several techniques that were proposed and have not gained broad clinical acceptance include percloacal deworming of tortoises, intrapulmonic therapy in chelonians, and intracoelomic administration of anesthetic agents.36–38
Antibiotics
Several antibiotics are currently used in reptile antibacterial therapy. Many pharmacokinetic studies have been published, so background information is available for assisting in selection of dose and dosing interval for several drugs in a number of species, although more research is necessary in the most common species presented to reptile veterinarians. A number of individual circumstances guide antibiotic selection in each case. Empiric antibiotic selection may be necessary in critical cases and should be based on the prevalence of specific pathogenic organisms for the disease syndrome, antimicrobial spectrum of activity, distribution of the antimicrobial to the diseased tissues, potential side effects, metabolic and excretory pathways, volume of the formulation necessary to deliver the necessary dose, dosing interval, and ease of administration. In many cases antibiotics can be withheld until culture and sensitivity results are available and antimicrobials are selected accordingly. Selection of antibiotics with a narrow spectrum of activity may help reduce the risk of therapy shifting the balance between enteropathic and commensal bacteria in the favor of the pathogens with the potential risk of infection. Multiple antimicrobials may be used to combat resistant strains of bacteria, and current guidelines for achieving synergy should be followed.39
Antibiotics often used in reptile medicine include amikacin, azithromycin, ceftazidime, ciprofloxacin, clarithromycin, danofloxacin, enrofloxacin, marbofloxacin, metronidazole, oxytetracycline, piperacillin, ticarcillin, trimethoprim/sulfamethoxazole, and tylosin. Most of these have been in use for decades and have been previously described.4 Information presented here is limited to agents with recent advances.
Azithromycin is an azalide, a subclass of macrolide antibiotics that exert their bacteriostatic effect by inhibiting protein synthesis by binding to the 50S ribosome.40,41 It concentrates in phagocytes and fibroblasts, which may increase its distribution to inflamed tissues. It is available as an oral suspension, oral tablets, and an IV injection and, as a dihydrate, is soluble in water. On administration it is widely distributed in human tissues but not cerebrospinal fluid. Its antimicrobial activity is pH-related and appears to be reduced with decreasing pH. It is eliminated unchanged via biliary and some urinary excretion in humans. Renal impairment has a minor effect on elimination. Drug–drug interactions are minor and not sufficient to warrant altered dosing. Its antimicrobial spectrum includes many gram-positive and gram-negative agents, including aerobes such as Staphylococcus spp., Streptococcus spp., Haemophilus spp., Bordetella spp., Neisseria spp., and Moraxella spp.; anaerobes including Peptostreptococcus spp. and Prevotella bivia, and other notable bacteria including Legionella pneumophila, Chlamydophila spp., Mycoplasma spp. Azithromycin demonstrates cross-resistance with erythromycin-resistant gram-positive strains; most strains of Enterococcus faecalis are resistant, and methicillin-resistant staphylococci are also resistant. Hepatotoxicity has been reported in humans, and azithromycin could cause reversible nonregenerative anemia in reptiles. It can contribute to dysbiosis and overgrowth of enteropathogens. Oral preparations should not be given concurrently with aluminum-containing or magnesium-containing antacids or phosphate binders.40 In a single-dose pharmacokinetic study in Ball Pythons (Python regius), the authors found that the drug is distributed and excreted similar to humans, and the dose, 10 mg/kg per os (PO), is given at different frequencies based on the location of the infection: skin, every 3 days; respiratory tract, every 5 days; and liver/kidneys, every 7 days.42
Ceftazidime is a semisynthetic, broad-spectrum, bacteriocidal, beta-lactam antibiotic for parenteral administration.43 It exerts its effect by disrupting the synthesis of the peptidoglycan layer in bacterial cell walls. It is highly stable to most clinically important beta-lactamases, either plasmid or chromosomal, so it is active against many organisms that are resistant to penicillins and other cephalosporins. Solutions for injection range from light yellow to amber depending on the diluent and volume used, and the pH ranges from 5 to 8. It is administered either IV or IM, is minimally metabolized, and is primarily excreted unchanged in urine. The dose should be reduced in patients with renal insufficiency, and in humans, elevated levels of ceftazidime can cause neurologic signs. Prolonged therapy with ceftazidime may lead to a fall in prothrombin activity, and vitamin K therapy may be needed.43 It can contribute to dysbiosis and overgrowth of enteropathogens such as Clostridium difficile. It is active against numerous bacteria. Gram-negative aerobes include Acinetobacter spp., Citrobacter spp., Enterobacter spp., Escherichia coli, Haemophilus spp., Klebsiella spp., Neisseria spp., Morganella spp., Proteus spp., Providencia spp., Pseudomonas spp., Salmonella spp., Shigella spp., Serratia spp., and Yersinia enterocolitica. Gram-positive aerobes include Staphylococcus spp., and Streptococcus spp. Anaerobes include Bacteroides spp. (although many strains of Bacteriodes fragilis are resistant), Clostridium spp. (but not C. difficile), Peptococcus spp., and Peptostreptococcus spp. It is synergistic with aminoglycosides against Pseudomonas aeruginosa and the enterobacteriaceae. It is synergistic with carbenicillin against P. aeruginosa. It is not active against methicillin-resistant strains. A single-dose pharmacokinetic study was performed in five snake species kept at 30°C (86°F) in which the half-life of 20 mg/kg IM was 24 hours and a dosing interval of 72 hours was proposed at that temperature.44 A single-dose pharmacokinetic study in Loggerhead Sea turtles (Caretta caretta) maintained at 22° to 26°C (75°F) found a serum half-life of 19 to 20 hours, and the authors proposed a dose of 22 mg/kg every 72 hours.45 In a single-dose pharmacokinetic study of ceftazidime 22 mg/kg IM at the same time as fluconazole 21 mg/kg SC in cold-stunned Kemp’s Ridley Sea turtles (Lepidochelys kempii) maintained at 21° to 24°C,46 two of the turtles died during the study, and E. faecalis was cultured from the blood and lung of one of them. The maximum concentration (Cmax) was similar to the study in Loggerhead Sea turtles (61.3 μg/mL versus 69.9 μg/mL, respectively), but the half-life in Kemp’s Ridley Sea turtles was more than double that of Loggerheads (43.9 versus 19.1 hours, respectively).45,46 Ceftazidime is widely prescribed to numerous species in reptile clinical medicine because of its broad-spectrum, low administration volume (when diluted to 100 mg/mL) and prolonged dosing interval of 48 to 72 hours. Further research with boids, lizards, tortoises, and freshwater turtles is needed to determine appropriate dosing in these species.
Clarithromycin is a semisynthetic, bacteriostatic, macrolide antibiotic that inhibits protein synthesis by binding to the 50S ribosome.47 It is soluble in acetone, slightly soluble in methanol, ethanol, and acetonitrile, and practically insoluble in water. It is available in the United States as immediate-release tablets, extended-release tablets, and granules for oral suspension. It is rapidly absorbed from the gastrointestinal tract in humans, with approximately 50% bioavailability, and it is metabolized by the liver into 14-hydroxy clarithromycin, which is also microbiologically active. Clarithromycin and the 14-hydroxy metabolite distribute readily into human tissues and fluids; intracellular concentrations were greater than serum concentrations but cerebrospinal fluid was not tested.47 Both clarithromycin and the 14-hydroxy metabolite are excreted primarily in urine. The antimicrobial spectrum includes a variety of gram-positive and gram-negative bacteria as well as Mycobacterium avium complex bacteria. Clarithromycin has been shown to be active against Staphylococcus spp., Streptococcus spp., Haemophilus spp., Moraxella spp., Mycoplasma spp., Chlamydophila spp., various mycobacteria, and Helicobacter pylori (particularly when in combination with amoxicillin and either omeprazole or lansoprazole). It may also be effective against aerobes including Bordetella spp. and Pasteurella spp., and anaerobes including Clostridium perfringens, Peptococcus niger, Propionobacterium acnes, and Prevotella melaninogenica (Bacteroides melaninogenicus). Most methicillin and oxacillin-resistant isolates are resistant to clarithromycin. Side effects may include hepatotoxicity, and hepatic enzymes should be tested before and monitored during therapy. It should not be administered concomitantly with several drugs including colchicine, anticoagulants, statins (HMG-CoA reductase inhibitors), cisapride, terfenadine, pimozide, or astemizole. Clarithromycin may affect metabolism or serum concentrations of midazolam, alprazolam, digoxin, theophylline, itraconazole, carbamazepine, omeprazole, and ranitidine. It should not be used in gravid or pregnant animals. It can contribute to dysbiosis and overgrowth of enteropathogens such as C. difficile. Clarithromycin may be used to treat severe cases of mycoplasmosis in tortoises.48,49 A recent long-term dosing study showed that clarithromycin therapy can be safe and effective (at resolving clinical signs but not eliminating the organism) in Desert Tortoises (Gopherus agassizii) when 15 mg/kg clarithromycin oral suspension (50 mg/mL Biaxin oral suspension, Abbott Labs, Abbott Park, Ill) is administered by gavage every 3.5 days (every 84 hours).50 This dose results in median clarithromycin concentrations in plasma that rose from 3.32 μg/mL at 14 days to 4.79 μg/mL at 6 months, which is above the proposed target range of 2.0 μg/mL; no 14-hydroxy clarithromycin was detected.50 The study also showed that per rectum administration did not achieve plasma concentrations in the target range when given every 12 hours for 48 hours followed by every 24 hours for 8 days.50
Danofloxacin mesylate is a synthetic fluoroquinolone for SC injection in cattle.51 It exerts its bacteriocidal activity by inhibiting the bacterial DNA gyrase enzyme to block DNA replication. The FDA-approved label dose is 8 mg/kg once or 6 mg/kg repeated in 48 hours for bovine respiratory disease associated with Mannheimia (Pasteurella) haemolytica and Pasteurella multocida. It is rapidly absorbed, highly bioavailable, distributed widely in tissues, and has concentrations exceeding that in plasma. Danofloxacin has a half-life in cattle of 3 to 6 hours and has negligible accumulation. It can cause a transient local tissue reaction at the injection site. It is active against gram-negative and gram-positive bacteria.51 Like other fluoroquinolones, danofloxacin causes articular chondropathy in adult animals at doses exceeding 18 mg/kg and should be used with caution in juveniles. A study assessing the effects of concomitant treatment with ivermectin and danofloxacin in sheep showed that the danofloxacin elimination half-life and systemic exposure (area under the curve [AUC]) increased.52 A pharmacokinetic study in healthy and experimentally infected chickens with P. multocida showed that a dose of 5 mg/kg via gavage was sufficient to provide tissue levels above the minimum inhibitory concentration (MIC) of the organism; tissue levels were less in infected chickens but still above the MIC.53 A single-dose pharmacokinetic study was performed in Loggerhead Sea turtles after IV, IM, and SC administration of 6 mg/kg.54 Elimination half-life was 18.7 hours after SC administration and 14.7 hours after IM administration. Maximum plasma concentration and time to maximum concentration after SC and IM administration were similar for both routes (approximately 10 μg/mL and 1.5 hours, respectively). The authors speculate that danofloxacin 6 mg/kg IM or SC every 48 hours could be safe and effective against bacteria with an MIC ≤0.5 μg/mL in Loggerhead Sea turtles and called for a multidose pharmacokinetic study to test the hypothesis.54 Danofloxacin has been administered at 6 mg/kg SC every 48 hours for 30 days to treat chronic mycoplasmosis in Gopherus species tortoises.9
Marbofloxacin is a synthetic, broad-spectrum, bacteriocidal fluoroquinolone antibiotic approved by the FDA for skin and soft tissue infections in dogs and cats and for urinary tract infections in dogs.55 It is available as an oral tablet in the United States and Europe and as an injectable product in Europe. Its antimicrobial action is via inhibition of DNA gyrase, like other fluoroquinolones. It is soluble in water, but solubility decreases in alkaline conditions. Marbofloxacin is rapidly and almost completely absorbed from the gastrointestinal tract after oral administration to fasted dogs and cats. Bioavailability in dogs is 94%. Ten percent to 15% is metabolized by the liver in dogs. Approximately 40% of the oral dose is excreted unchanged in the urine of dogs, and 70% of the drug (85%) and its metabolites (15%) are excreted in the urine of cats. The remainder is eliminated in feces via biliary excretion. Marbofloxacin is bacteriocidal against a broad range of gram-negative and gram-positive organisms including Staphylococcus spp., E. coli, Proteus mirabilis, Streptococcus spp., P. multocida, E. faecalis, Klebsiella pneumonia, Pseudomonas spp., Corynebacterium spp., and Bacillus spp. Divalent cations diminish the absorption of fluoroquinolones, including calcium, magnesium, aluminum, and zinc, and drugs including sucralfate, so concomitant administration is contraindicated. Marbofloxacin, like other quinolones, is contraindicated in immature animals because it can cause articular chondropathy, and it should be avoided in animals with known central nervous system disorders. Pharmacokinetic studies have been performed in Ball Pythons, Loggerhead Sea turtles, and Red-eared Sliders, and its metabolites have been studied in Ball Pythons.56–60 In the Ball Python, 10 mg/kg was administered IV or PO to animals maintained at 30°C, and testing continued for only 24 hours, so the half-life could not be calculated; however, the authors proposed a dosage of 10 mg/kg every 48 hours.59 The pharmacokinetic studies in Loggerhead Sea turtles maintained at 26° to 28°C included dosing of 2 mg/kg IM, IV, and PO. While maximum concentrations and half-life differed somewhat among administration routes, the authors proposed administration of 2 mg/kg every 24 hours is sufficient for all three administration routes.56,57 Marbofloxacin, 2 mg/kg, was administered IV and IM to Red-eared Sliders in the forelimbs and hindlimbs. Bioavailability was 77% for IM forelimb and 63% for IM hindlimb administration, but no significant difference was found for AUC, clearance, or half-life between locations. The authors determined that IV administration of 2 mg/kg every 24 hours is appropriate at this dose for organisms with an MIC ≤1μg/mL, and IM administration of 2 mg/kg every 24 hours is appropriate for organisms with an MIC ≤0.25μg/mL.58 Higher doses should be investigated.
Metronidazole is an oral, synthetic, nitroimidazole antiprotozoal and antibacterial agent. It is bacteriocidal against obligate anaerobes (MIC ranging from 0.25 to 8 μg/mL) and is protozoacidal against numerous protozoa with an in vitro MIC of ≤1μg/mL.61 The antimicrobial action targets DNA synthesis, in that metronidazole acts as an electron acceptor in the phosphoroclastic reaction and causes breaks in the adenine–thymine base pairing. Although resistance is uncommon, it can occur. Metronidazole is available as an IV infusion (metronidazole HCl) and as oral tablets (metronidazole base) in the United States. Metronidazole is a basic compound with a pKa value of 2.62; it is moderately soluble in water (approximately 10 mg/mL at 25°C) at pH 2.5 to 8.0; the pH of the IV infusion is 5.8.61,62 Oral suspensions using pH-appropriate suspending agents can be procured from licensed compounding pharmacies and are generally the most appropriate formulation for accurate dosing in reptile patients. Metronidazole is well-absorbed after oral administration and is widely distributed to most body tissues and fluids.62 The major route of elimination of metronidazole and its metabolites is via the urine (60% to 80% in humans), and fecal excretion accounts for 6% to 15% of the dose.61,62 Metabolism to the 2-hydroxymethyl metabolite occurs, and decreased plasma clearance occurs in patients with decreased liver function but not in patients with renal insufficiency. Side effects are primarily gastrointestinal in humans, although neurotoxic, hepatic, and hematopoietic effects can occur. They include nausea, vomiting, and ataxia, reversible neutropenia, dysuria, yeast overgrowth, encephalopathy, seizures, and peripheral neuropathy. Metronidazole potentiates the effect of coumarin anticoagulants, and concomitant administration with drugs that induce microsomal liver enzymes (e.g., phenytoin or phenobarbital) may accelerate the elimination of metronidazole. Drugs that decrease microsomal liver enzymes (e.g., cimetidine) may prolong its half-life and decrease its plasma clearance.61 It should be used with caution in patients with blood dyscrasia, and it may cause mild leukopenia. It is contraindicated in the first trimester of human pregnancy. Elevated liver enzyme activities in plasma have been reported in reptiles.4 Serious toxic side effects in reptiles can occur at doses between 40 and 100 mg/kg.4,63 Metronidazole is bacteriocidal against (1) gram-negative anaerobic bacilli including Bacteroides spp. (including B. fragilis group) and Fusobacterium spp., (2) gram-positive anaerobic bacilli including Clostridium spp. and Eubacterium spp., and (3) gram-positive anaerobic cocci including P. niger and Peptostreptococcus spp.61 It is trichomonacidal and amoebacidal for protozoa with in vitro MIC ≤1μg/mL.61 Pharmacokinetic studies in Red-eared Sliders, colubrid snakes (Elaphe guttata and Elaphe obsoleta), and Green Iguanas have been published.16,64–66 In Red-eared Sliders, a single intracoelomic dose of 20 mg/kg was administered; the maximum plasma concentration was 25 μg/mL at 5 hours after injection, and the elimination half-life was 27 hours.16 The authors suggest an intracoelomic dosage of 20 mg/kg every 48 to 72 hours, depending on the MIC of the organism it is directed against, but caution that adverse effects did occur; thus the intracoelomic route may not be safe. In colubrid snakes, single doses of 20, 50, 100, and 150 mg/kg PO have been studied, and multiple 20-mg/kg doses have also been studied.64,65 The maximum plasma concentration varies from 12.8 μg/mL after 20 mg/kg PO every 48 hours for 6 days to 2039 μg/mL after a single dose of 150 mg/kg PO. The half-life was 15 hours after the first 20-mg/kg dose, 23 hours after the sixth dose, and 26 hours after the 150-mg/kg dose.64,65 No clinically significant adverse behavioral, hematologic, or biochemical effects were observed other than mildly elevated plasma lactate dehydrogenase activity after the last 20-mg/kg dose and mildly decreased glucose after the 50- and 150-mg/kg single doses.64,65 Taken together, the results support a dosage of 20 mg/kg every 48 hours in Elaphe spp. snakes. Higher doses should be reserved for resistant infections so that the risk of adverse effects is avoided. A pharmacokinetic study of a single 20-mg/kg dose followed by 20 mg/kg every 24 hours for 10 days in Green Iguanas found a maximum plasma concentration (Cmax) of 7.6 μg/mL after the single dose with a half-life of 12.7 hours and a Cmax of 22.8 μg/mL after 10 days.66 No adverse hematologic, biochemical, or behavioral effects were observed after the 10-day treatment trial. The authors concluded that 20 mg/kg every 48 hours would be sufficient for “most” infections and that the dosing frequency could be increased to every 24 hours for resistant strains with MICs ≥8 μg/mL.66
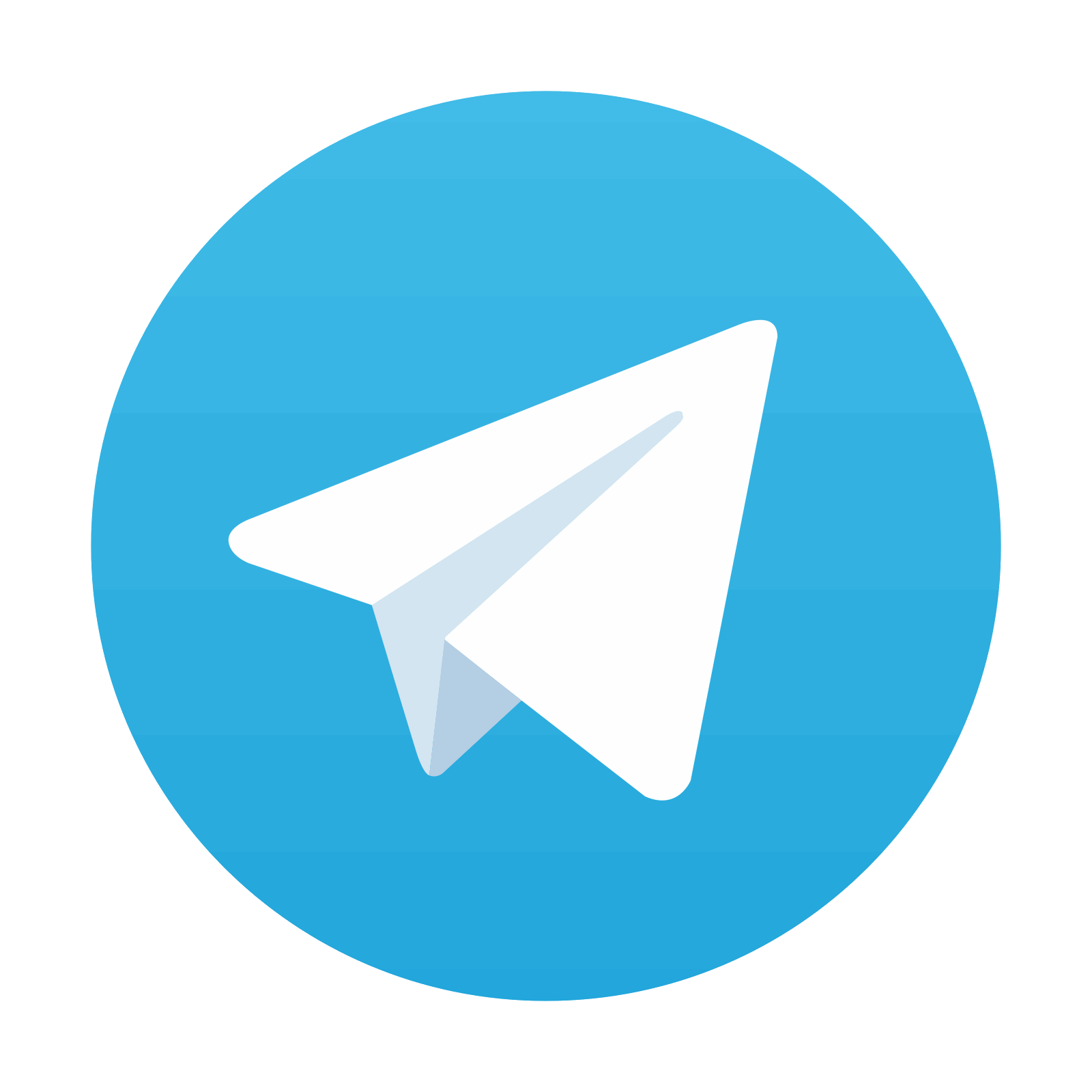
Stay updated, free articles. Join our Telegram channel

Full access? Get Clinical Tree
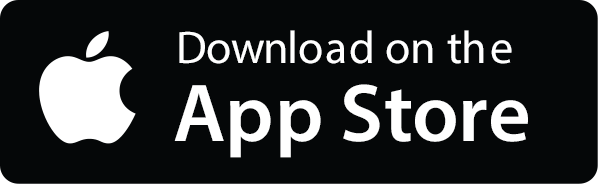
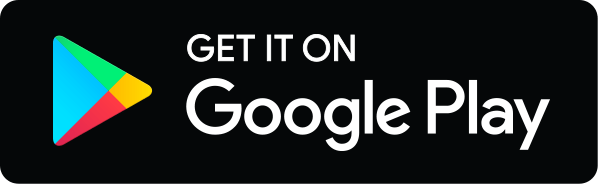