(1)
Department of Neuroscience, Oregon National Primate Research Center, Oregon Health and Science University, Beaverton, OR, USA
Abstract
The rhesus macaque represents a pragmatic animal model for elucidating mechanisms underlying normal and pathological human behaviors. Many of the same techniques that are used in clinical studies can be readily applied to the nonhuman primate studies. These including the use of Actiwatch recorders for monitoring of 24-h activity–rest cycles and the use of a remote blood sample collection system for assessment of changes in circadian hormone profiles. In addition, comprehensive rhesus macaque gene microarrays (Affymetrix) are now commercially available, and these can be used for profiling gene expression changes under various physiological and pathological conditions. Our recent application of these methodologies to rhesus macaque studies emphasizes that many physiological and behavioral events, and the expression of associated genes, have a distinct 24-h expression pattern. Consequently, it is important to take these circadian rhythms into account when designing experiments and interpreting the results.
Key words:
Circadian rhythmsphotoperiodgene microarrayscortisolDHEASsleep1 Introduction
1.1 The Rhesus Macaque as a Pragmatic Translational Animal Model
Humans and rhesus macaques (Macaca mulatta) are both long-lived primates, and they show many similarities in their anatomy, physiology, and genetics (1). Consequently, these nonhuman primates are regarded as pragmatic animal models for studying mechanisms that underlie normal and pathological human physiology and behavior. Their use as translational animal models has many advantages. For example, rhesus macaques can be maintained under carefully controlled environmental conditions (e.g., photoperiod, temperature, diet, and medication). In addition, animals of a specific age, size, sex, and genetic characteristic can be selected, thereby eliminating extraneous variables and self-selection bias that are typically associated with human clinical trials. Moreover, because the timing of the necropsies can be carefully controlled in rhesus macaques, high-quality postmortem tissues and RNA samples can be collected for biochemical analysis and gene expression profiling.
The present chapter describes two methodologies that we use routinely in rhesus macaques studies to help with the interpretation of physiological and behavioral data. These include continuous monitoring of 24-h activity–rest cycles and remote collection of serial blood samples for the determination of 24-h circulating hormone profiles. Both of these methodologies have analogous applications in clinical research and highlight the important role that circadian rhythms play in human physiology and behavior. To gain further insights into the underlying mechanisms we also routinely perform comprehensive gene expression profiling, using GeneChip microarrays (Affymetrix, Inc., Santa Clara, CA, USA), and this will be discussed in the context of effective experimental designs that take circadian variations into consideration. For details of the latter procedure and data analysis the reader is referred to two recently published articles (2, 3).
1.2 Circadian Rhythms
Most animals, including humans, live in an environment that is characterized by daily and seasonal changes in lighting and temperature. Consequently, many aspects of their physiology and behavior show circadian and circannual adaptations. On the one hand, circadian rhythms are intrinsic to a wide range of body functions, including the sleep–wake cycle, metabolism, immune response, and reproduction (4), and a common human manifestation of desynchronized circadian rhythms is jet-lag. On the other hand, many mammals also show seasonal rhythms in metabolism, reproduction, and immune function (5, 6). Interestingly, in humans seasonal variations have been reported for blood pressure, immune response, birth rate, and sleep duration, as well as for behavioral traits associated with seasonal affective disorders, bulimia nervosa, anorexia, and suicide (7). Many of these physiological and behavioral alterations can be linked to underlying changes in the secretion of hormones, such as cortisol, dehydroepiandrosterone sulfate, testosterone, leptin, and melatonin, all of which have distinct 24-h release patterns in the rhesus macaque (8–11).
The present chapter focuses on two methodologies that can be readily applied to both human and nonhuman primate studies, in order to gain insights into an individual’s circadian physiology. For example, performance testing, collection of diagnostic specimens, and the administration of medications, all have an optimal time of day. Hence, information about an individual’s 24-h activity–rest cycles and associated 24-h hormone profiles provides an important physiological context for interpretation of test results, and also for the design of effective drug and hormone-replacement paradigms.
2 Materials and Methods
2.1 Monitoring Activity–Rest Cycles and Remote Blood Sampling
A convenient, noninvasive method of continuously monitoring motor activity in humans involves the use of Actiwatch recorders (Philips-Respironics, Bend, OR, USA; part number U198-0301-00). These unobtrusive watch-size recording devices are typically worn on the wrist, and continuously collect activity–rest data, providing information about 24-h motor activity rhythms as well as insights into the quality of sleep. These devices comprise an accelerometer and a 32-Hz microprocessor, which selects peak activity intensity during each second to sum up activity counts per sample. Using a 1-min epoch setting Actiwatches can continuously record data for 45 days, which can then be readily downloaded to a personal computer using a dedicated data reader (Philips-Respironics; part number 198-0150-00). Actiware 5.0 software can then be used to determine the total level of activity in an individual, and importantly, the mean level of activity during specific times of the day (e.g., day time or night time). Equally important, the software can analyze bouts of activities that occur during the night and provide insights into sleep quality based on parameters such as sleep latency, sleep fragmentation index, and number of wake bouts. Note, Actical recorders are also commercially available (Philips-Respironics; part number 198-0210-03). They are very similar to Actiwatch recorders except they average the activity intensity (instead of taking the peak activity intensity) during each second to sum up activity counts per sample. They are used with Actical 2.1 software and are primarily used in studies that focus on energy expenditure studies rather than sleep.
Rhesus macaques, like humans, are diurnal and also show consolidated sleep patterns. Consequently, we have made effective use of the same Actiwatch devices to study 24-h activity–rest cycles in rhesus macaques (12–15). Attaching the Actiwatch to the animal’s wrist is not a viable option and so instead we usually place it inside an aluminum protective case (Philips-Respironics; part number 198-0232-00 M); this case is compatible with the nylon and aluminum primate collars available from Primate Products, Inc. (Immokalee, FL, USA) and can be unobtrusively worn around the animal’s neck. For downloading the stored activity data, the animal is briefly sedated using ketamine (10 mg/kg body weight, i.m.) and the collar/recorder removed. Alternatively, if the animal is trained to enter a small transfer cage, which restricts head movement, then the Actiwatch can be removed without resorting to the use of sedation.
An alternative strategy to using the protective case and collar, which we have also found to be very effective, is to place the Actiwatch directly inside a custom-made small pocket at the rear of a nylon mesh primate vest (Lomir Biomedical, Inc., Malone, NY, USA). These protective vests are worn by our animals when they have been fitted with an indwelling subclavian or jugular vein catheter and connected to a swivel-based remote infusion/sampling system (Lomir Biomedical, Inc.). This minimally invasive long-term blood sampling system (Fig. 9.1), when combined with the Actiwatch recorder, enables 24-h hormone rhythms to be monitored with reference to the light–dark cycle as well as with reference to the animal’s endogenous activity–rest cycle (Fig. 9.2). As already indicated, many hormones show a pronounced 24-h release pattern. Consequently, many serial blood samples are needed to clearly disclose changes in the hormone secretion pattern, and often these samples need to be collected when an individual is asleep. The situation is even more complicated when attempting to disclose plasma profiles in hormones, such as luteinizing hormone (LH), which is released episodically or in a pulsatile manner. For example, the first major endocrine event associated with the onset of puberty in primates is an evening increase in the amplitude of LH pulses. Further subtle differences occur across the menstrual cycle of adults (16), in which the follicular phase is associated with high-frequency low-amplitude pulses whereas the luteal phase is associated with low-frequency high-amplitude pulses (Fig. 9.3). By remotely collecting small (<0.3 ml) blood samples every 10 min for 24 h, it is possible to establish detailed pulsatile LH release profiles for each individual, without perturbing its sleep–wake cycle or behavior.
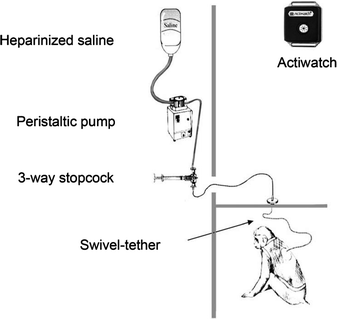
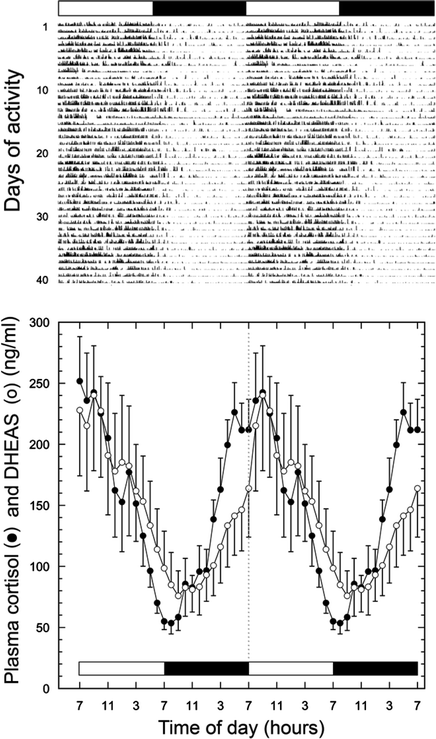
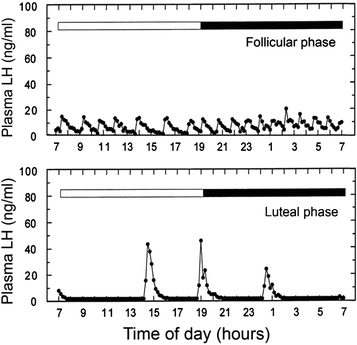
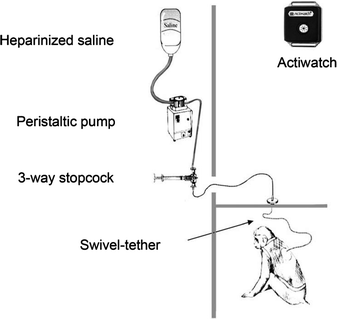
Fig. 9.1.
Schematic illustration of the set up that enables serial blood samples to be collected remotely from conscious, undisturbed monkeys. The animals are surgically fitted with an indwelling subclavian vein catheter, which is channeled subcutaneously to the middle of the back. There, the catheter is connected to sampling tubing which itself is protected by a flexible stainless steel tether and, in turn, is connected to a swivel assembly at the top of the cage. The tubing then passes through a small port in the wall and into a laboratory, where it is connected via a three-way stopcock to a blood sampling syringe and also to a peristaltic pump; the latter is used to maintain catheter patency by continuously infusing a heparinized saline solution. Using this set up, serial blood samples can be collected throughout the day and night, even while the animals are asleep. The vests that the animals wear are to protect the catheter; they are routinely inspected every week and washed every 2 weeks. In addition, the vests can house a watch-sized Actiwatch recorder (inset, upper right) for continuous monitoring of activity–rest cycles.
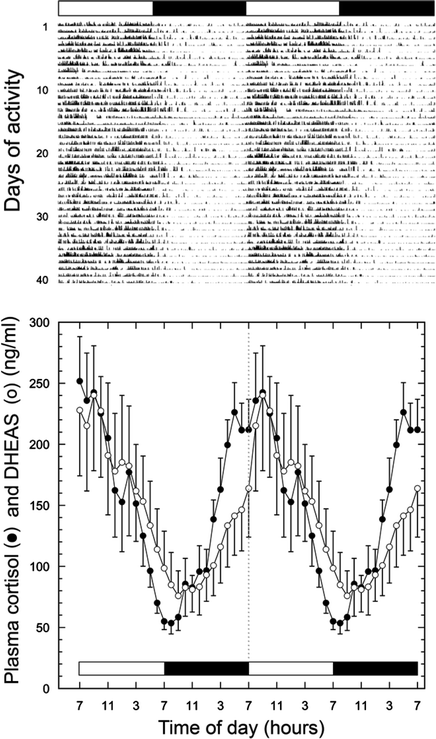
Fig. 9.2.
Upper panel: Actogram from an individual rhesus macaque, showing diurnal activity and indication of consolidated nocturnal sleep, as in humans. Note that the data are double-plotted to facilitate viewing of the circadian rhythm, and the periods of light and dark are represented by white and black horizontal bars, respectively. Lower panel: Mean plasma cortisol and DHEAS profiles from young adult rhesus macaques; the serial blood samples were collected every hour, using the remote blood sampling set up depicted in Fig. 9.1. The hormonal data are double-plotted to facilitate visualization of the 24-h rhythms, which show peaks in the morning when the animals begin their daily activity. [Figure adapted from (12), with permission, Copyright 2006, The Endocrine Society.]
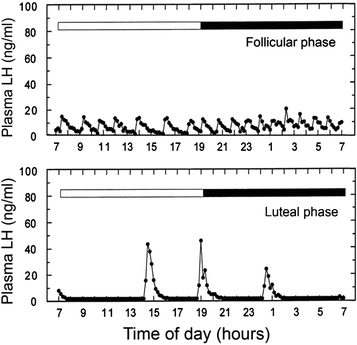
Fig. 9.3.
The remote blood sampling set up (Fig. 9.1) enables detailed changes in the episodic release profiles of hormone such as LH to be disclosed. The upper panel shows a well-defined 24-h pulsatile plasma LH profile from a female rhesus macaque, sampled during the follicular phase of the menstrual cycle. The lower panel shows a 24-h pulsatile plasma LH profile from the same animal, sampled during the luteal phase of the menstrual cycle. These data emphasize that multiple serial blood samplings are essential for the disclosure of subtle changes in the release of some hormones.
2.2 Notes on Activity Recording
The actograms generated by the Actiware 5.0 software are typically double-plotted (Fig. 9.2). This means that each line of data represents two consecutive days worth of activity; the second day’s data are duplicated at the start of the next line, etc. This double-plotted form of activity depiction helps with the visualization of the activity rhythm, especially when it shows daily drift (e.g., when it is uncoupled from external environmental cues and is free-running). When humans or monkeys are maintained under fixed photoperiods, such as 12 L:12D (i.e., 12 h of light and 12 h of darkness per day), analysis of the actograms reveals the intensity of total daily activity, as well as activity during the day and activity during the night. Such data are valuable in establishing whether an individual is showing normal activity behavior. For example, using a night-vision camera we have established that rhesus macaques typically wake up a few minutes before the lights come on in the morning at 7:00 h; that is, they anticipate when dawn will occur. They then show a major peak of activity in the late morning, just after their morning feed at ∼8:00 h. Finally, after the lights turn off in the evening (19:00 h), the animals continue to move around for a few minutes but generally close their eyes soon afterward and cease to show major episodes of motor activity. An animal that is sick typically does not show such a biphasic diurnal activity profile; it may show an attenuated level of activity during the daytime or excessive activity at night. Consequently, an additional use for the Actiwatch is to gain insights into the general health of an animal. A way to further refine the nocturnal activity is to perform a sleep analysis. However, it should be emphasized that this component of the Actiware 5.0 software has been validated for human studies only, and so strictly speaking, when applying it to the analysis of nonhuman primate activity one cannot refer to it as “sleep analysis” but simply as “nocturnal activity analysis.” Nevertheless, the algorithm has been designed to provide information about “sleep fragmentation” and “sleep latency,” which are used to establish whether a human subject is experiencing perturbed sleep, or in the case of nonhuman primates, whether the animal is showing unusual activity during its normal nocturnal rest period. Note that the epoch setting of the Actiwatch needs to be set to 1-min bins, or short, in order for this function to work. A practical application of this analysis is that if an individual is not sleeping well because of some pharmacological intervention or illness, then performance in a cognitive task may be impaired – indirectly because of perturbed sleep–wake or activity–rest cycles (14). Note also that the absolute level of activity detected by the Actiwatches may be influenced by whether an animal is caged single or pair-caged. As part of a psychological enrichment strategy, rhesus macaques at ONPRC are typically group housed or pair-caged, which promotes beneficial grooming behavior. On the other hand, when animals are caged individually, as when part of a remote blood sampling study, pair caging is not recommended because the protective catheter tubing from the two animals can become entangled. So instead, we cage the animals individually but install a semi-open partition between adjacent cages; this enables neighboring animals to perform mutual grooming without invading each other’s cage floor space. Animals kept under different housing/caging conditions may show difference in their absolute activity levels, and this needs to be taken into account when comparing data from animals that have been housed differently.
2.3 Notes on Remote Blood Sampling
2.3.1 Potential Biohazard
When collecting body fluids, such as blood, from nonhuman primates the investigators need to treat them as if they are potentially pathogenic and take appropriate protective measures. Typically this involves a face mask, eye protection, gloves, and gown. Moreover, the blood tubes should be capped during centrifugation, to avoid creating potentially infectious aerosols.
2.3.2 Overview of Remote Blood Sampling
In our sampling set up (Fig. 9.1), the dead space in the line (i.e., the volume between the sampling stopcock and the tip of the vascular catheter) is typically <2 ml. Consequently, before collecting a blood sample, we first draw out this dead volume of heparinized saline from the line and discard it. We then withdraw a saline–blood mixture, until only dark blood is visible in the line. Finally, we collect the blood sample and immediately deposit it into either a heparin-coated or EDTA-coated ice-cold borosilicate glass sample tube. Note, some hormone assays are adversely affected by specific anticoagulants; therefore, the choice of anticoagulant will depend on the hormone assay systems that will subsequently be employed. After the sample has been collected, an infusion of heparinized saline is used to flush the line and to restore vascular fluid volume.
Note, some investigators include an additional step in the blood sampling procedure, in which red blood cells from the sampled blood are re-suspended in heparinized saline and re-infused into the animal. We prefer to avoid this step as it increases the possibility of introducing a blood clot or infection. Provided the volume of sampled blood from an adult rhesus macaque does not exceed 50 ml in a 24-h period and the animal is given time to build up its hematocrit before the next sampling session, significant anemia is avoided.
2.3.3 Protocol for Remote Blood Sampling
Note, although heparinized saline (4 IU/ml of 0.9% saline solution) is continuously infused (∼1 ml/h) into the animal to keep the vascular catheter patent, this concentration of heparin is too low to prevent coagulation of a drawn blood sample. Therefore, blood should not be allowed to stagnate in the sampling line for more than a minute or so. With practice and preparation (e.g., pre-filling flushing syringes with heparinized saline) the entire procedure can usually be completed within 2 min. The following protocol refers to the set up depicted in Fig. 9.1 and describes the key operations of the remote blood sampling procedure:
1.
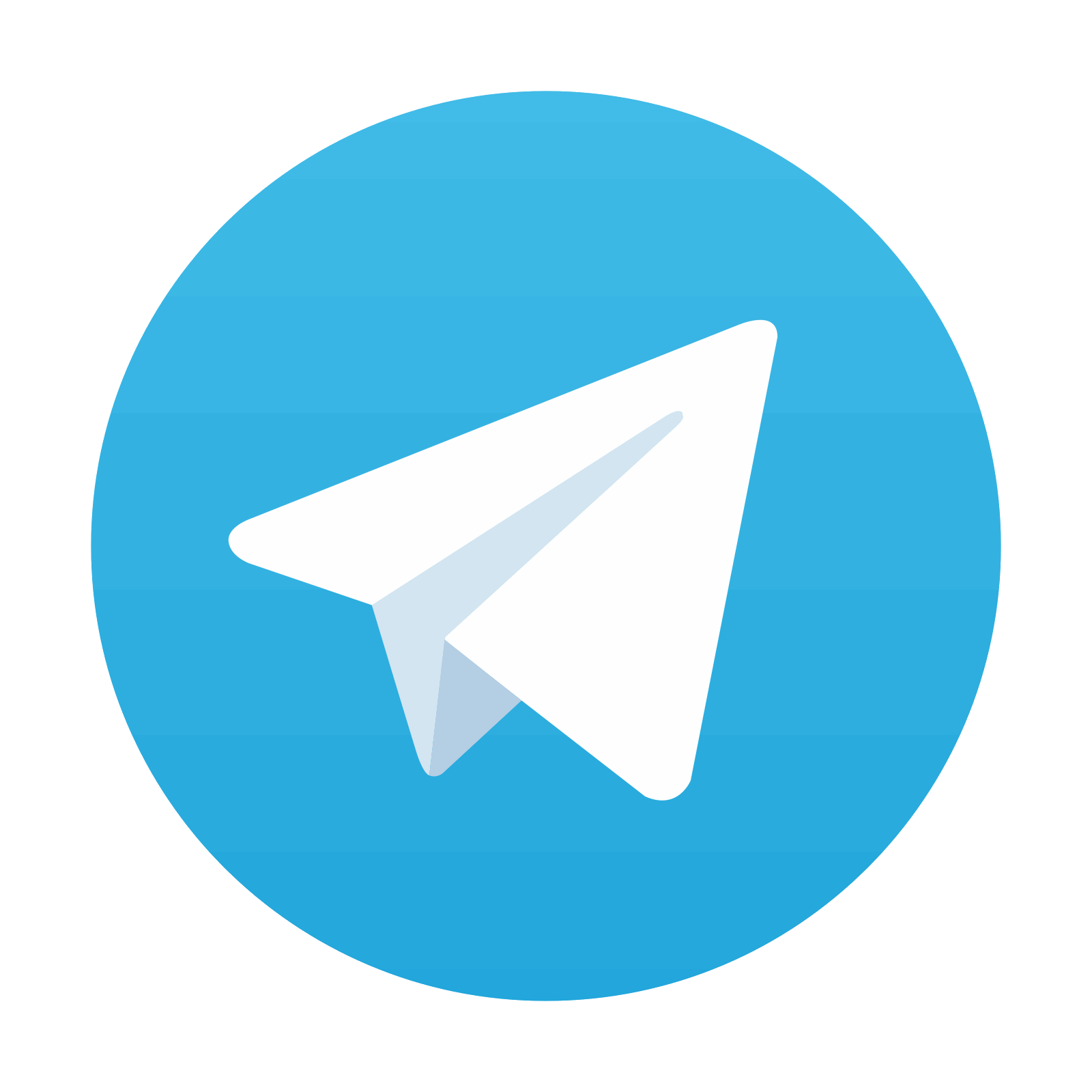
Removal of dead space saline: Turn the stopcock lever toward the pump port (to close the supply line) and draw all of the clear saline from the line (animal’s side) into a 3-ml syringe; the volume removed should be about 2 ml. Turn the stopcock lever to a position midway between the pump and the syringe ports (to close both ports). Remove the syringe, discard its contents into a receptacle containing bleach, and re-attach it to the stopcock.
< div class='tao-gold-member'>
Only gold members can continue reading. Log In or Register a > to continue
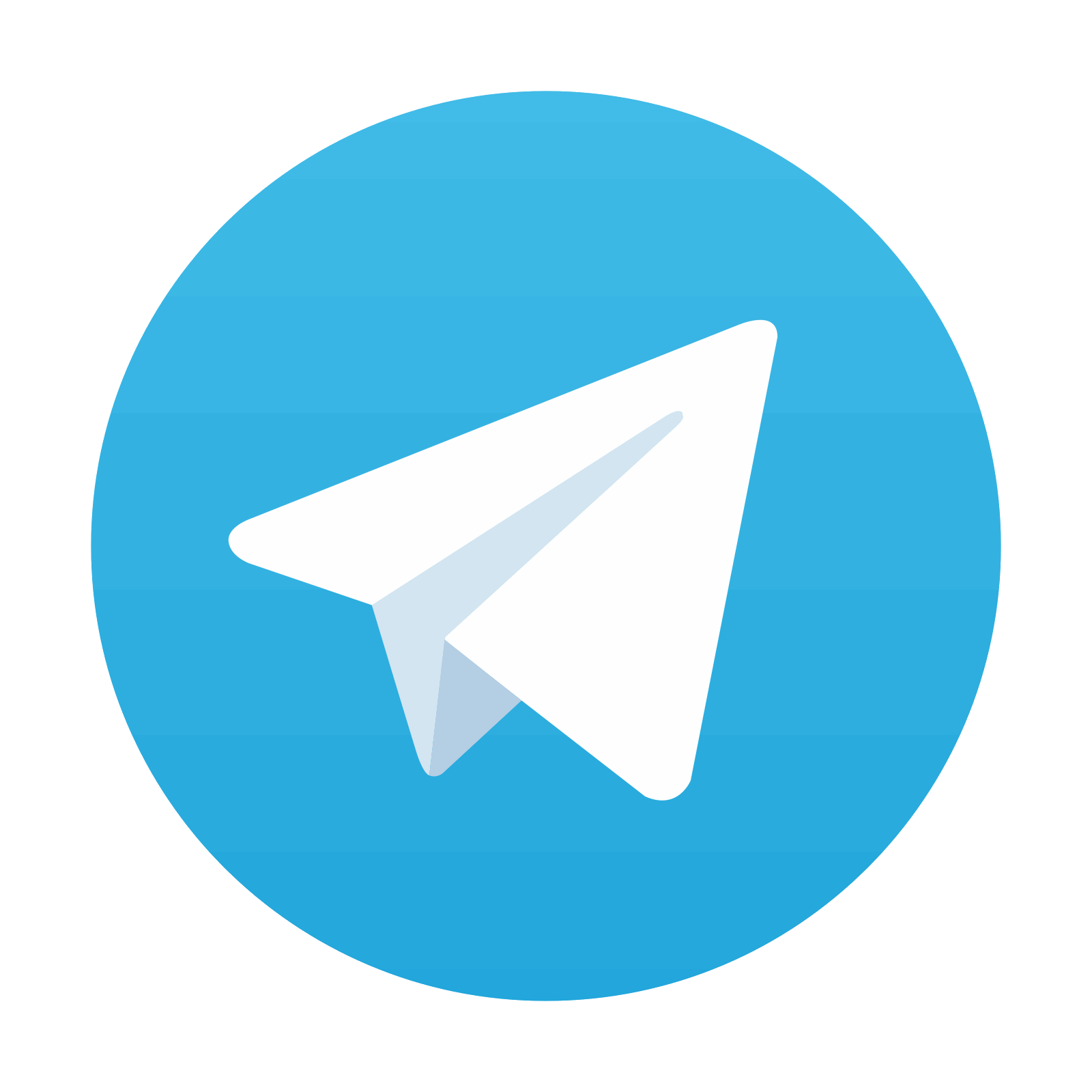
Stay updated, free articles. Join our Telegram channel

Full access? Get Clinical Tree
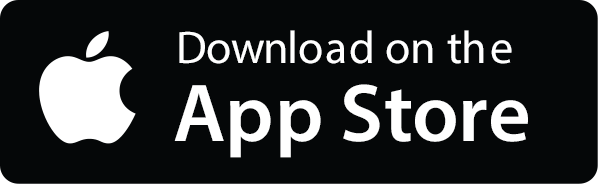
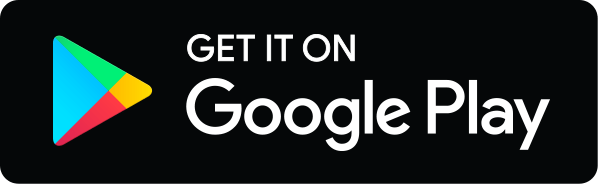
