and Lois M. Black2
(1)
Department of Behavioral Neuroscience, Oregon Health and Science University, Portland, OR, USA
(2)
Center for Spoken Language Understanding, Oregon Health and Science University, Beaverton, OR, USA
Abstract
Autism spectrum disorders (ASDs) are characterized by impairments in communication, social interaction, and the presence of restricted and repetitive behaviors. Impairments in the capacity for social interaction include deficits in the use of nonverbal behaviors, failure to develop peer relationships, lack of motivation to share enjoyment with others, and lack of social reciprocity. This multidimensional diagnosis, both sophisticated and nuanced, contrasts sharply with our limited assessments of mouse “social behavior,” which typically involve measures of approach behavior. Our objectives here are to examine the deficits in social interaction in ASD, highlighting the role of affective and nonverbal communication, and then to provide the theoretical and empirical basis for expanding translation-relevant measures of impaired social interaction in mouse models. We ask whether diminished approach toward another mouse, the current behavioral paradigm for mouse models of autism, is sufficient to capture the nuanced social impairments featured in the autism diagnosis. We conclude that these tests should be complemented by assessments of mouse social reward, vocal communication, and mouse abilities to respond to the emotional cues of others. General protocols for several of these behavioral tests are presented.
Key words:
Mousegeneticsenvironmentpervasive developmental disordersautismADOSADI-Rempathyreciprocityprosodyanimal communicationsocialitysocial motivationsocial reward1 Introduction to the Autism Diagnosis: Social Interaction Impairments
Impairment in social interaction, as described in DSM-IV-TR (1), is manifested by at least two of the following:
marked impairment in the use of multiple nonverbal behaviors such as eye-to-eye gaze, facial expression, body postures, and gestures to regulate social interaction;
failure to develop peer relationships;
a lack of spontaneous seeking to share enjoyment, interests, or achievements with other people (e.g., by a lack of showing, bringing, or pointing out objects of interest); and
a lack of social or emotional reciprocity.
The ADOS, as a “gold standard” research tool in ASD, provides, through a series of semi-structured activities, opportunities to observe how a child behaves and interacts with a clinician–examiner in order to rate the child on core ASD symptomology (2–4). The clinician judges the child’s use of both verbal and nonverbal forms of communication that support social interaction. Ratings include whether communications have reciprocal intent or are dominated by the child’s own interests or focus; whether the child’s social overtures and social responses are appropriate; whether the child shows a broad range of communicative affective expressions; and whether the child links verbal and nonverbal communications in a subtle and flexible way that supports reciprocal, emotional social interactions.
The ADOS, in combination with other diagnostic instruments, mitigates some of the inherent variability of clinical diagnosis of a human social disorder. Inevitably, as carried out by many clinician–examiners, ratings can be subject to frame of reference effects and the clinician’s own level of insight and experience. These ratings require substantial experience, clinical training, and expertise. Moreover, ratings are susceptible to changes in cultural and clinical perspectives regarding normal and impaired levels of social expressivity and responsivity. Culturally, there are disparities in emotional expression across societies, ethnicity, social-economic status, rural versus urban upbringing, gender, age, and variations in parental personalities in response to societal expectations. Against these environmental influences, the child inherits a rich genetic variability and its own individual experiences that underlie a variety of social capacities and motivations that have nothing to do with ASD per se. A child can be shy, reclusive, introverted, or extroverted. A child can also have sensory and other impairments and psychological issues that influence social skills. For example, poor peripheral vision, internal pain, sensory hypersensitivity, and language impairment can all impair social functioning. Other contributing factors include abuse, poor coping skills, and poor social models at home or school.
In this regard, there is growing recognition that autism research with human subjects would benefit from automated tools to measure and analyze on-going verbal and nonverbal communication in ASD. Such tools would ideally employ thoroughly blind methods that allow for a precise focus on the behavioral feature of interest without interference from other features. Technologies are being developed that may ultimately provide better tools to identify atypical behaviors. For example, eye-tracking technology can help elucidate idiosyncratic nonsocial forms of attentional focus (5, 6), and machine learning/pattern recognition analyses can be employed to help automate assessment of facial affect and gestural movements (7, 8). Speech technology combined with statistical analysis methods can aid in exploration of vocalizations and automated assessment of prosody in autism and other disorders (9). Given the enormous progress that has been made in computer performance and algorithm sophistication, it is likely that these technologies only mark the beginning of a revolution in automated, highly sensitive behavioral assessment. As many of these methods focus on nonverbal behavior, they raise the possibility of applying similar or even identical methods to the study of behavioral features of ASD in nonhuman species.
Social interactions require that a participant detect a host of verbal and nonverbal cues associated with the other participant’s emotions and intentions, that one participant has an internal emotion in response to these cues, and that the other participant then expresses his own emotional responses in a fashion that can be detected by the first individual. We might consider this interaction as a reciprocity chain that links emotion with expression and ensuing emotional response with expressive response. We can consider each link by its temporal and contextual features. For example, there could be a temporal progression from the first change in the emotion of an individual (1), to the outward expression of the emotion (2), to detection of that individual’s expression by a companion (3), to changes in the companion’s emotional state (4), which begins the cycle again. Among humans, this progression of expressed and perceived emotions is mediated primarily via nonverbal visual cues, such as eye gaze, facial expressions, bodily postures, gestures (10), as well as vocal prosody cues. Affective communication can be amplified when visual and auditory cues are spontaneously expressed in a coordinated fashion.
Communication and expression of emotions in the “reciprocity chain” is supported when an individual is attuned to the inter-subjective and situational context and can respond and express himself consistently across communication modalities. Consider, for example, a child who is talking to another in a flat tone, irrespective of the emotional import of the environmental context or unresponsive to the semantic content of the child’s own or the other’s communications. In this case, the flat-toned expression could index either a deficit in awareness of the other’s emotions or mental state, and/or a deficit in the child’s own self-expressivity. In either case, it may signal impairment in social–emotional reciprocity. The same concerns could be raised if a child were to talk in a singsong melody regardless of the content of what was said or the situational context. Thus, a lack of a dynamic, multimodal response to an emotionally salient event may identify an abnormal response or impairment in social–emotional reciprocity. To translate impairments of social interaction from the clinic to the lab bench and then back again, we might directly investigate the “stimulus–response” relationships between outward expressions of emotion.
1.1 Expressions of Emotion: Vocal Prosody
Let’s now consider in more detail the deficits in vocal communication associated with ASD. Social interactions critically involve individuals to both vocally express their emotions and to understand and respond to the emotional expressions of others. The emotions experienced by an individual can be expressed through the semantic content of what is said. If I tell you that I feel angry, despondent, frustrated, defeated, overwhelmed, cagy, indignant, exhilarated, content, proud, or surprised, you have an idea of how I feel. Emotions are also critically conveyed by how words are said; that is, through vocal prosody. Prosody can be described at multiple levels. At the acoustic level, it refers to the usage of features, such as variations in the pitch of a phrase or sentence, the duration or loudness of a particular syllable, or vocal quality (e.g., tense, hoarse, breathy). At the mechanical–physiological level, prosody refers to how these acoustic features result from the complex interplay of all components of the speech production apparatus, ranging from the lips to the lungs. At the brain level, prosody refers to how these components, in turn, are controlled in a coordinated fashion by multiple brain systems. Finally, at the functional level, we can delineate the following classes of functions. Affective prosody refers to the expression of internal emotional experiences of joy, sadness, achievement, or anger. For example, a flat pitch contour can convey sadness, while significant dynamic changes in pitch and energy levels can indicate greater emotional salience. A tremble in a voice can indicate sadness or fear.
Grammatical prosody helps us to distinguish between meanings of words or sentences. Stress on a particular syllable can help us to differentiate a verb (pre-sent’) from a noun (pre’-sent) and the different meanings of a word, while upward trend in pitch can help us identify a question from a string of statements. Pragmatic prosody is used to help us put emphasis on an important idea, sentence, or word in a sentence and thus reveals our focus and intentions. Enhanced duration and loudness (energy) can indicate the salient point of a statement. For example, “It wasn’t the blue chair you spilled ink on, it was the NEW chair.” Prosody allows us to express subtle or not so subtle attitudes. For instance, one can be sincere or sarcastic depending on how one says, “I really want to go to dinner with you.” In another, and quite different function of pragmatic prosody, people speak to infants in “motherese” or “infant-directed talk” (11) which they do not use with older children. People speak differently to a policeman than they do to an intimate partner. Pragmatic prosody thus also signals the context, roles, and relationships between speakers. Although it is customary in human research to include role-related prosody in the category of pragmatic prosody, we will include it in the category of affective prosody for studies of rodent vocalizations. While role-related prosody is relevant for mice, its classification as “pragmatic prosody” would be inappropriate because of its strong verbal connotations and because, even in humans, role-related prosody typically has an affective component (e.g., motherese cannot be de-coupled from the feelings toward the baby).
1.2 Prosody in ASD
Atypical prosodic expression has been considered a hallmark of autism since the disorder was first described (12). Prosody in ASD has been described as monotonous or flat (13), stilted, robotic, overly melodious, or singsong (14). Atypical features include aberrant stress, unusual pitch patterns, abnormalities of rate and volume, and problematic quality of voice (e.g., hypernasality) (15, 16). Children with ASD are more likely to express odd phrasing patterns and to exhibit abnormalities in their modulation of pitch, and in their rate and volume of speech compared to typically developing children (17). Unusual prosody in very young children, ages 12–24 months, has been found to be one of the “red flags” that distinguish ASD from other developmental disabilities (18).
Unusual prosody may interfere with the communicative effectiveness of speech, for example, by making it difficult to determine whether or not a statement is intended as a question, or what emotion is being conveyed. In fact, certain repetitive patterns of specific pitch, duration, or energy changes irrespective of verbal content may trigger an unintentional negative emotional response in the listener, which may then severely disrupt the reciprocity chain. In ASD, atypical prosody very much impacts communicative competence and reciprocal social interactions.
Using objective methods, expressive prosody issues have been documented in about 50% of a group of adolescents and young adults with ASD (16). Receptive prosody issues have been less frequently studied, but are thought to be closely associated with expressive deficits, both having been looked at in autism in studies that use controlled perceptual methods (17, 18). Automated methods for the study of expressive prosody are few and will likely expand on the labor-intensive approaches pioneered in seminal studies, such as Shriberg’s PVSP (16, 19). Manual approaches unavoidably reduce the amount of data available for research (e.g., it is not unusual to analyze only the first 50–100 utterances of an individual’s language sample, even when there may be many more relevant utterances in a spontaneous conversational dialogue across different topics or activities, for example, using the ADOS). Second, the use of the human ear in the PVSP and in Peppé’s methods introduces additional variables, particularly for detection of more subtle cases of atypical prosody (9). Clinical judgment ratings on the ADOS for atypical prosody, for example, have such poor inter-rater reliability, that this item, despite its importance, has never been included in any version of the ADOS “algorithm,” that subset of items most critical for a diagnosis.
Advances in speech technology, mathematical analyses, and algorithm development, however, are making it increasingly possible to perform automated analyses of expressive prosody. For example, preliminary findings using automated analysis of expressive prosody show that even when in ASD certain prosodic contrasts (e.g., focal stress) are expressed clearly; they are expressed with an atypical usage of prosodic features that are not detected by human observers (9). Speech technology can also create stimuli for the study of receptive prosody with carefully controlled or manipulated speech stimuli that cannot be produced by humans, such as affectively distinctive speech stimuli that have identical speaking rates, or stimuli in which certain prosodic features (e.g., pitch) are altered while keeping others (e.g., duration) invariant (20, 21). These speech manipulation methods can in principle be applied to nonhuman vocalization as well, for example, to determine which acoustic features are behaviorally relevant when studying different strains of mice in different situations.
1.3 Nonverbal Communication in ASD: Facial Expression, Eye Gaze, and Gesture
Nonverbal communication is thought to be a critical component of social interaction and communication of affect. Evidence exists that facial and gestural communication are impaired in ASD, both expressively and receptively, as is the use of eye gaze.
1.3.1 Facial Expression
Facial expressivity has been described as more constricted in range, more flat or neutral, and more idiosyncratic and ambiguous in children with ASD (22). Behavioral research on facial expression is itself extremely limited, however, due to the enormous amount of labor involved in the manual coding of facial expressions (e.g., using variants of the Facial Affect Coding Scheme (FACS) (23)) and the still early state of automated methods. Machine learning models of automatic affect recognition, although fast progressing, are not yet capable of analyzing facial affect in natural situations. Current methods, able to capture spontaneous affect, still require a nearly invariant frontal view of the individual and hence cannot easily be used in dynamic social situations (7).
Difficulties in the receptive processing of facial affect have been documented in ASD in numerous behavioral studies (24–29). Lindner and Rosén (30), using both static and dynamic (video) facial affect stimuli, vocal affect, and verbal content, as assessed with the Perception of Emotion Test or POET (31), showed that children with Asperger’s (As) have more difficulty than TD children identifying emotions. Mazefsky and Oswald (32) using the DANVA (33), which measures facial affect and tone of voice unimodally at two levels of intensity, showed that children with As perform similarly to the standardization sample, whereas those with high functioning autism (HFA) perform significantly worse on both facial and vocal affect, and significantly worse than As at low levels of intensity. Thus differences emerge depending on the subtlety of the affects expressed, the behavioral measures used, the methodology employed, and who the subjects in the research groups are, which, too frequently, are not adequately characterized.
Studies with functional MRI indicate that children with autism have less activity in the fusiform gyrus, more activity in the precuneus, and reduced activity in the amygdala relative to TD children (34). Among adults, fMRI scans indicate that individuals with ASD take longer to habituate to repeated exposures to faces, accounting for what sometimes looks like conflicting findings of both under-arousal and hyperarousal of the amygdala in ASD; also shown was a significant correlation between reduced neural habituation and the severity of social deficits (35). Given that habituation is associated with learning and novelty detection, reduced neural habituation implies difficulty in extracting social information and adapting to the unfamiliar.
1.3.2 Eye Gaze
Averting eye gaze or atypical eye contact with others is a well-recognized aspect of ASD (17). Children with ASD have difficulties using eye gaze to initiate and maintain interactions, to reference objects beyond need-related requests as, for example, in pure social exchanges of sharing pleasure, feelings, uncertainties about the environment, and, in fact, people with ASD prefer to look at objects rather than other people or their faces (5). Notable is the deficit in the linkage of eye gaze with other forms of communication, especially gestures and vocalizations, in order to share attentional focus with another. Difficulties in initiating joint attention or responding to the bids of others in joint attention are among the most prominent deficits that lead to ASD diagnosis before the age of 3 (36–38).
1.3.3 Gesture
Gestures are communicative actions that make use of fingers, hands, arms, facial, and head movements, as well as body movements and postures. Limited use of gestures in children has also been considered a red flag for early identification of ASD in 12–24-month olds (18, 39–41) and a diagnostic marker for older children (4). Lack of communicative, descriptive, and emotional gestures appears, for example, on all ADOS algorithms for all modules. Gestures have a critical pre-linguistic communicative and interpersonal function in the very young. The earliest deictic gestures emerge between 7 and 12 months, with their meaning known from context (i.e., reaching to be picked up; pushing an object away for refusal; pointing to an object wanted; showing or giving an object to another for sharing) and make up nearly 80% of infants and toddlers’ gestures (42). Representational and symbolic gestures, which have meaning in themselves and signify features of an object or action, start to appear around 12–24 months (e.g., fingers to mouth to represent “eating;” waving a hand to say “bye”). These gestures usually emerge within inter-subjective contexts and social routines, such as play with caregivers and singing songs accompanied by gestures with peers (i.e., “itsy bitsy spider”), and are thought to depend on modeling by and observation of others.
Tools currently in use to assess quality and types of gestures typically involve impressionistic observations or videotaping activities structured to elicit gestures and then using manual coding schemes to rate them (e.g., 18, 42). Although not yet applied as an analysis tool for autism, there is progress in pattern recognition methods for gestural analysis in other fields. For example, technology tools have been developed to precisely capture expert surgeons’ manual movements in order to implement robotic surgery (43). The possibility of applying similar methods to the analysis of gestural communication in autism may be on the horizon.
Weaknesses in understanding and using gestures are thought to relate to fundamental impairments in ASD in imitation (44, 45), possibly due, in part, to underlying motor deficits (46). In turn, imitation difficulties in ASD have been linked to neural dysfunction in the mirror neuron system (47, 48). Mirror neurons, which were first discovered in area F5 of the macaque, have been documented in humans, especially in the analogous area to F5, the pars opercularis of the inferior frontal cortex (Brodmann’s area 47), as well as in parietal cortex (49, 50). Mirror neurons are said to fire when one performs a goal-directed action as well as when one observes a goal-directed action performed by someone else. Thus the mirror neuron system has been characterized as the neural mechanism whereby others’ actions, and the motor plans for them, as well as others’ intentions and goals are quickly or even automatically learned, even by just observing. In interconnection with other cortical areas, such as superior temporal sulcus and visual areas that respond to biological motion, the mirror neuron system likely plays a role in the human ability to imitate others. Via interconnection with the insula and limbic system, the mirror neuron system may support our understanding of others’ minds and the ability to empathize (48, 51, 52). Using facial affect imitation and observation tasks in an fMRI study, Dapretto et al. (53) found significant differences in mirror neuron activity between children with ASD and TD (especially in the right inferior frontal lobe with links through the insula to the limbic system).
1.4 Empathy in ASD
ASD has been linked to impairments in the ability to perceive others’ emotions, intentions, and mental states, as well as in the ability to perceive that the experience of another individual is different from one’s own. These concepts are embodied in the notion of empathy. In the early twentieth century, empathy was coined from the German word, einfühlung, or “feeling into” (54). This term has since been expanded and refined by numerous authors (55, 56), but contemporary definitions of empathy continue to maintain a primary role for affective reactivity to others (57). Eisenberg (58) defined empathy as “an affective response that stems from the apprehension or comprehension of another’s emotional state or condition, and which is similar to what the other person is feeling or would be expected to feel” (p. 72).
The mechanisms that underlie deficits in empathic ability in autism are far from well understood and are being researched at both neural and behavioral levels. Some studies focus on impaired and atypical social information processing at the behavioral level and the cascade of events that it can induce over time. These deficits may be tied to (both precursor and consequent) functional and structural brain abnormalities. There is research that relates many of the known social deficits in autism to dysfunction in the “social brain” (which includes, especially, the amygdala, fusiform gyrus, limbic system, and areas of prefrontal cortex) (59, 60). Other studies, as mentioned, are investigating dysfunction in the mirror neuron system and its interconnected circuitry, especially as related to deficits in imitation ability to understand others’ intentions, and empathy (48). There has also been important evidence of uneven distribution of white matter and neural under-connectivity in recent research. For instance, studies have shown functional under-connectivity, measured using correlations between time series of pairs of regions of interest in fMRI (61), as well as structural under-connectivity (62). Uneven distribution and volumes of white matter in ASD may contribute to this reduced interconnectivity (63). White matter volumes may be significantly (25% or more) larger in certain brain areas (e.g., the frontal lobe) and smaller in other areas (e.g., the corpus callosum). The uneven distribution of white matter, together with atypically larger, but not functionally more productive areas, points to inefficient interconnectivity. In addition to these findings, there are additional features at the microscopic level that may also have implications, such as increased numbers of minicolumns (vertical columns through the cortex layer) with greater cell dispersion in autism (e.g., Casanova 64).
Implications of under-connectivity include impairments in the integration of complex social information. Understanding of others’ emotions and the processing of other relevant nonverbal social cues may itself be thwarted by the lack of exposure and focus in autism on the relevant information. For example, Klin et al. (5) employed eye-tracking technology to better understand how individuals with ASD view the world. In a series of experiments in which participants watched video clips from Edward Albee’s Who’s Afraid of Virginia Woolf, individuals with autism were found to focus significantly less time on the eye region of the actors’ faces and significantly more time on the mouth, body, and object regions in the film compared to normal controls. Eye-tracking studies in toddlers with ASD have shown, similarly, a preference to look at the mouth rather than eyes, objects over people, and to orient to physical rather than social contingencies (6). Because the nonsocial focus of visual pursuit takes place at a very young age, children then miss out on much socially relevant information and social learning that would allow for greater understanding of interpersonal contexts and improved social adaptation. However, it is unknown to what degree such biases and lack of input is causal, leading to further neural and neurodevelopmental changes, or themselves caused by fundamental neural impairments in brain circuitry.
Under-connectivity may also result in poor cross-modal integration in ASD, which has been documented via behavioral, anatomic, electrophysiological, and neuroimaging methods. At the behavioral level, Wetherby et al. (18), for example, used the systematic observation of red flags (SORF), rated by two people with good inter-rater reliability (k=0.94) to confirm the presence of six red flags that distinguish children with ASD during the second year of life from those with DD (developmental delay) and TD. One of those flags was the lack of typical “coordination of gesture with eye gaze, facial expression, or vocalization” (with at least three modalities coordinated) and was found in 100% of the children with ASD.
As with the above explanation underlining the lack of vital social input early on, there is strong plausibility for poor cross-modal integration being a major obstacle to empathic ability, since empathy requires a near-automatic integration of many subtle behavioral cues in multiple modalities simultaneously. Although behavioral observations have testified often enough to the lack of linkage in multiple verbal and nonverbal modalities, most of these studies have used subjective judgment or manual coding methods. There are, however, studies underway to examine this in an objective behavioral paradigm and to automate detection of affective communication in individual modalities (i.e., facial, gestural, postural, verbal, and vocal prosody) and analyze, via mathematical algorithms, their temporal coordination and synchrony.
As we transition here to a discussion about how we assess mouse models that may be relevant to autism, we can see the tremendous translational benefit of analysis tools that assess gestures and vocalizations and behavioral responses to these social signals. Analogous diagnostics can be developed, in theory and in practice, for studying laboratory mice. Do mice share these nuanced social abilities? In the subsequent sections, we will explore the social repertoire of the feral and laboratory mouse, including a broad assessment of the expressive and receptive aspects of mouse communication.
1.5 The Social Aptitude of the Laboratory Mouse
When a genetic linkage to autism is found, mouse studies can help to discern whether there is a causal relationship between the mutation and changes in brain function or behavior relevant to autism. Mice are useful species for genetics research because they have a mammalian brain and a relatively short generation time (10 weeks from conception to reproductive maturity). The entire mouse genome has been sequenced, mouse genes can be deleted, gene expression can be changed (65), and human genes can be inserted into the mouse genome (66, 67). With these tools, relationships between genetic mutations and social behavior can be examined. After mutations in fmr1 were linked to fragile-X disorder (68), a knockout mouse strain (fmr1KO) was generated to model the disorder (69). The fmr1KO was then studied for relevant cognitive, social, and seizure-related deficits (70–73). As a result, this mouse model is now being used to explore pharmacological and genetic treatments for the symptoms of fragile X (74–76). Other findings of genetic linkages to autism have resulted in knockout mice with disruptions in WNT2 (77), MECP2 (78), engrailed 2 (79), CAPS2 (80), and neuroligin-4 (81).
Are mice capable of social interactions that would make the study of mouse behavior relevant to autism? Feral mice have been found at population densities of up to 4,000 mice per acre (82, 83). In the wild, both males and females establish territories and social hierarchies (84–86) and compete for food, territory, and mates (87). Dominant males can distinguish mice that belong in their territory and the degree of relatedness of mice in adjacent territories (86). In natural environments, social arrangements are dynamic, fluctuating with season and food availability (88). Aggressive behavior is more common at high population densities, while gregarious social groups form when the resources for each mouse are more plentiful (89, 90). Social arrangements are also dynamic, changing with the birth of new litters, with movements of mice into and out of geographic areas, with the death of dominant males, and changing food and mate availability.
One could imagine that a mouse with an impaired ability to recognize expressions of dominance, submissiveness, or the changing needs of offspring might have difficulties surviving in a natural population. For instance, a mother is behaviorally responsive to the wriggling calls (91) and distress calls (92) of her pups. One could envision that a nonresponsive mother would be less likely to produce viable litters. Inability to recognize some of the cues that dictate social hierarchies might also result in forced expulsion from the territory of a dominant male or female. A mouse that avoids social interaction might remain ignorant of social cues for safe foods to eat, safe times and places to forage, and mate availability. Taken as a whole, the natural environment likely imposes strong selective pressures for abilities to detect fear or pain in another mouse and recognize the conventions of social hierarchies (for an enlightened overview, read Crowcroft 93).
1.6 Mouse Social Approach Tests
Against this backdrop of mouse social capacities in natural environments, we can consider the most useful and widely employed approach for examining the social capabilities of a laboratory mouse; the social approach test. The social approach test has been variously attributed as measure of mouse “sociability,” “sociality,” “reciprocal sociality,” or “social interaction.” This social interaction test has been used extensively to examine mice with targeted alleles relevant to autism (see Section 2). Social approach behavior of a “test” mouse is measured typically after a period of social isolation. During isolation, the test mouse becomes habituated to a cage where it resides. A stimulus mouse is then introduced to the test mouse’s cage. During the test, the stimulus mouse explores the cage and the test mouse follows or “approaches” the stimulus mouse. “Sociality” in this test is defined as a composite measure of social approach; the fraction of time that the nose of the test mouse is within a short distance of the head, flank, or anogenital region of the stimulus mouse. Social approach is most vigorous after extended periods of social isolation and can vary with the time of day when social isolation occurs (94). This test has various permutations. Among juveniles, it can indicate “social interaction” (95). Among adult males or nursing females confronted with a visitor, this resident–intruder test measures aggressive behaviors (96).
It should be noted here that some very insightful information are gained about the social approach behaviors of a laboratory mouse by simply observing the home cage. For instance, dvl1 knockout mice do not huddle together or barber the whiskers of their cagemates like their wild type controls (97). This particular study highlights the importance of simply observing the home cage, in the colony room during periods when the white lights are on and also under dim red light (see details on mouse husbandry at the end of this chapter).
There are also automated versions of social approach tests, to rapidly determine whether a test mouse is more likely to approach an unfamiliar mouse, versus a familiar mouse, or versus an inanimate object (98). Approach behavior is indicated when the test mouse breaks an infrared beam and enters a room that contains a cage wherein the stimulus mouse resides. A more detailed description of this test is provided at the end of the chapter.
Just as social behaviors and motivations of children are different from those of adults, mouse behaviors likewise change with development. For instance, social approach behaviors of early adolescent mice can be very sensitive to genetic background and insensitive to the gender of the test mouse or the stimulus mouse. As mice approach reproductive maturity, social approach becomes more stereotypical: males approach females more than females approach males. Genetic influences of social approach behaviors may be marked by gender differences that emerge with reproductive maturity (99). There are several excellent references on the ontogeny of mouse behavior that should be considered (100–104). A common mistake is to consider a juvenile mouse as a smaller version of a juvenile rat, which is a very different rodent (105–108). For instance, social play among young mice is very infrequent and not at all robust (106), whereas young rats express “rough and tumble” play (108, 109). Further, mouse motor behaviors do not indicate strong “reciprocal” social interactions in this test. The stimulus mouse typically walks around the perimeter of the cage while the test mouse follows the stimulus mouse. These behaviors do not indicate play or reciprocity. It is possible that there are reciprocal vocal exchanges, but this aspect of their social exchange is currently unknown.
It can also be difficult to interpret differences in social approach response. Diminished approach toward another mouse could indicate shyness, social anxiety, reduced preference to engage in a social encounter, or an increased preference for social isolation. Shyness and social anxiety are not core features of autism. Preference for social isolation may have more to do with autism, at least as originally described and emphasized by Kanner (110) who characterized “autistics” as “aloof,” “indifferent,” and “isolated.” Currently, however, the spectrum of autism disorders is much broader and involves a more heterogeneous group of children for whom aloofness or lack of social interest may not be descriptive. Reduced social motivation is not mentioned as a core symptom of the DSM-IV, but it does characterize the broader autism phenotype as described by Dawson et al. (111).
Moreover, in humans “impaired social interaction” has a much more inter-subjective connotation than does diminished social approach, sociability, or “sociality.” A person with autism can be sociable and still have a severe impairment in social interaction. Social interaction has to do with the behavior of one individual impacting another individual, of individuals in interaction. At the very least, impaired social interaction assumes that social behavior is “inappropriate” in the means, timing, or manner in which it is done with respect to both norms (e.g., age, sex, cultural, contextual, social) and with respect to the individual to or for whom the behavior is directed. A question then arises, does the social approach test paradigm capture core symptoms of “impaired social interaction” in autism? Is it reasonable to think that it could, given some modifications to the paradigm?
1.7 Interpreting Social Approach Through the Lens of Reward Theory
Can we be sure that a diminished social approach behavior indicates a lack of social motivation? Approach behaviors of any sort toward a stimulus might suggest that the stimulus induces a psychological expectation of reward or pleasure, but this inference is far from guaranteed. For instance, an amoeba, which lacks a nervous system altogether, swims up a chemical gradient. Having a brain allows an animal to navigate through its environment to gain access to rewards and avoid painful stimuli, through the cues that are associated with these states. Such cues can be contextual (berries to eat or thorns to avoid) or temporal (a sting following a buzzing sound). This capacity to make associations allows an animal to adapt to dynamic conditions. For example, an animal can learn that a novel red berry is not sweet. By contrast, an amoeba cannot modify its behavioral strategy to obtain food. Its movement is dictated by membrane receptors, directed by fixed stimulus–response action patterns.
But how do we infer whether a mouse finds a stimulus rewarding or aversive? The question of how to infer animal motivation from animal behavior is a hard problem but it has been extensively studied. Through conditioning experiments (described below), we can infer animal capacities for fear and fear learning (112–114) and for the anticipation and consumption of rewards (115, 116). These capacities are supported by a highly differentiated limbic anatomy (117) and physiological systems that include glucocorticoids, dopamine, serotonin, and endogenous opiates (118). Drugs of abuse can co-opt these natural reward systems (119–123). Thus, mice respond to their environment, in part, based upon associations between particular contextual or temporal cues and underlying affective states, such as feelings of reward or fear.
How can we explore the relationship between social approach and these underlying experiences of reward or pain? The conditioned place preference (CPP) test is particularly valuable in this regard. CPP tests were developed in the context of a rich theoretical framework (120, 124–127). If a particular stimulus (such as access to morphine, cocaine, or a highly palatable food) is rewarding, then a test animal will choose to spend its time in an environment that it associates with access to this putative reward. In the CPP experiment, the test mouse is exposed to two environments discernable by distinct but unimportant qualities, such as particular beddings or odors. One environment is repeatedly paired with the presence of the putative reward and the other environment is conditioned by the absence of the reward. During the test, the mouse is allowed to roam between both of the conditioned environments. No reward is provided during the test period, but the mouse is expected to spend time in the environment formerly paired with the putative reward and less time in an environment where it either did not experience the reward (for example, saline or standard lab chow) or where it previously experienced something aversive. CPP tests show that exposures to morphine, cocaine, and highly palatable foods can be rewarding for rodents (120). CPP tests have also demonstrated that different kinds of social encounters, including play (126, 127), sexual interactions (128, 129), juvenile social interactions (130), mother–infant bonding (131), and even aggression (132), are rewarding to mice.
Within the context of the social CPP, we can ask whether social approach can be associated with social reward. The experimental framework of a social CPP is depicted in Fig. 7.1. BALB/cJ mice (BALB) express levels of much lower social approach than C57Bl/6 (B6) mice, consistent with previous studies (133, 134). The B6 strain shows a strong place preference for environments paired with social access. By contrast, the BALB strain shows no conditioned respond to the different environments; they do not find access to other juveniles rewarding. Comparison of these two strains indicates that social approach can be associated with social reward (130). A more detailed description of the social CPP test is described at the end of the chapter.
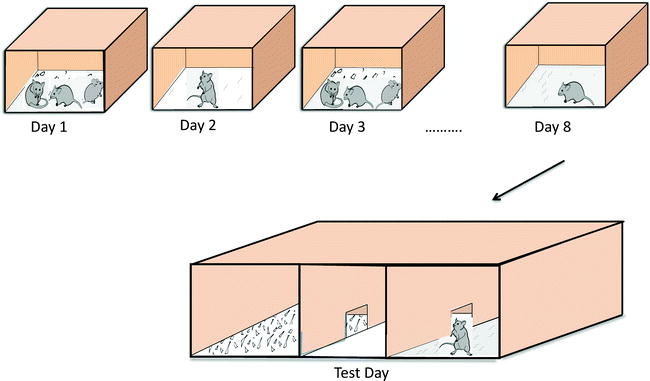
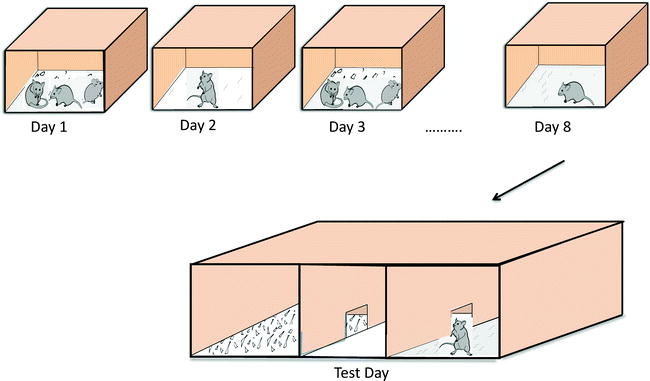
Fig. 7.1.
Juvenile mice learn to associate a particular kind of bedding with the presence or absence of social interactions. Mice are placed in clean bedding material each day that alternates between aspen shavings and recycled paper and either the presence of other juvenile mice or social isolation. After 10 days of conditioning, mice are tested for their preference of the bedding materials in the absence of other mice. Typical juvenile mice, such as the B6 mouse, prefer the beddings that they experienced when they had access to social encounter versus beddings associated with social isolation. BALB mice do not show this preference. This is a measure of social reward.
We can also envision situations in which the association between social approach and social reward does not exist. As mentioned earlier, a mouse strain might have a strong motivation for social novelty but an aversion to group housing. A mouse might avoid social approach for other reasons, such as shyness or social anxiety, yet prefer social housing to isolation. Social reward can be multiplicitous and ephemeral. Nursing dams, when they must choose between environments paired with access to newborn offspring versus access to cocaine, choose environments paired with offspring during the first week after birth, but when they have older pups, they prefer environments paired with access to cocaine (131).
Social reward is likely mediated, at least in part, by endogenous opioids, dopamine, and their receptors within the brain. These endogenous molecules are distributed within regions involved in emotional regulation and mediate responses to pain and stress, reward, and social bonding (135, 123). Activation of opioid receptors promotes maternal behavior in mothers and social play among juvenile animals (136–138) and can be modulated by agonists and antagonists of opioid receptors (139, 140). Separation distress, exhibited by archetypal behavior and calls in most mammals and birds, is reduced by opioid agonists and increased by opioid antagonists (141). Access to social encounter can, in turn, influence opioid physiology. For instance, social play and short-term social isolation alter opioid receptor binding in the nucleus accumbens and other regions in rats (142). Dopamine is also important in social interactions. In the nucleus accumbens, dopamine appears to be a necessary signal for pair-bond formation in male prairie voles (143). Social stress or social isolation promotes long-term changes in mesolimbic dopamine (144), and social isolation can render animals more sensitive to dopamine-releasing drugs of abuse (145, 146). There are also important roles for oxytocin and vasopressin in social approach behaviors (147, 148), which influence dopaminergic reward circuits (149). While endogenous opioids and dopamine can moderate social approach and response to social access, the interaction of these physiological mechanisms with social encounter does not rule out the possibility that mice, under some circumstances, might engage in or avoid social approach for other reasons, such as novelty-seeking, shyness, or social anxiety.
1.8 Modeling Impairments in Social Interaction in Mice
How can impairments in social interaction be examined in a way that captures mouse social capacities and has optimal translational value for autism research? Let us first consider the mouse social approach test within the context of the DSM IV-TR diagnosis. In what follows, we review the defining features of “qualitative impairment in social interaction,” as listed in the DSM IV, and ask within this set of criteria whether existing or new behavioral tests can be employed to interrogate behaviors relevant to autism. Let us look at a few of these manifestations and how they could be captured in our mouse models. Importantly, in considering these various tests, it is important to maintain a mouse colony and a behavioral testing environment that minimizes variation in mouse social behavior.
1.8.1 Impairment in the Use of Multiple Nonverbal Behaviors to Regulate Social Interaction
Could a mouse express nonverbal behaviors that fail to regulate social interaction? Just as there are proscribed behavioral patterns for bee waggle dances, fruit fly courtship behaviors, or the hunting sequence for members of a wolf pack, mouse social interactions that are bound by norms for the species. What would be the modalities of these interactions? Feral mice shelter in burrows and forage at night, so their natural environment typically occurs under low levels of light. In this environment, social interactions are mediated by vocalizations, visual and tactile cues, and odors. Mouse use of vision, relative to their other senses, is diminished. We will briefly review how mice communicate and sense each other via their auditory, olfactory, and visual senses, because these modalities form the basic components of social interaction.
Vocalizations: During social interactions, rats and mice often emit ultrasonic vocalizations (USVs) at frequencies above the limit of human hearing (15,000 cycles/s (hertz) = 15 kHz). Rats emit high frequency (50 kHz) upward modulated calls when they anticipate or experience reward and lower frequency (22 kHz) USVs under aversive conditions (150, 151). Consistent with these observations, rats actually self-administer playbacks of recordings of high frequency calls, but not low frequency USVs (150). When rats hear recordings of high versus low frequency calls, there is a differential induction of c-fos gene expression patterns in the brain (152).
Vocalizations among mice can provide information throughout development. When infant mice, from post-natal day (PD) 1 to 8, are displaced from the nest they emit distress calls at very high frequencies (> 90 kHz) that solicit the dam to return the pup to the nest (153). “Wriggling” calls (about 35 kHz) are emitted by pups within the nest and engender the dam to free the pup from an uncomfortable position under the dam or relative to other pups (92, 154). Call rates of pup USVs and maternal responses to these calls are sensitive to drugs that target affective states, such as opiates, dopamine, and serotonin (92, 155, 156).
Juvenile mice emit more complex USV patterns between weaning (PD 21) and adulthood. When a juvenile mouse is reunited with a conspecific after a period of social isolation, it can express an elevated level of social approach and emit a rapid rate of vocalizations. These USVs can be classified as syllables (vocal sounds separated by silence from other USVs) according to how their fundamental frequencies are modulated (such as upward, downward, chevrons, and punctuated calls). These vocalizations can be remarkably complex interactions (99) and vary with mouse strain. For instance, juvenile mice of the B6 strain show a vigorous social approach response and high number of downward modulated and punctuated calls relative to juvenile BALB mice. BALB mice express a higher rate of upward modulated calls during social union interactions (67). Interestingly, the pitch jumps between frequencies of punctuated syllables are more stereotyped among juvenile BALB mice and more varied among age-matched B6 (99), in line with the concept that BALB/c mice have many behavioral features relevant to autism (133, 134). Among adults, these complex call structures can also be sensitive to specific genetic mutations, such as BTBR (157). Like rat vocalizations, adult mouse USVs are responsive to mating access (158, 159), the odors of conspecifics (160, 161), and exposure to drugs, such as amphetamine (162) and alcohol (163).
Odors: Mouse scents are also powerful social cues for mice. Information about age, sex, genetic background (164, 165), degree of kinship (166), and parasitic infection (167, 168) can be conveyed through odor. A mouse “marks” its urinary scent within its environment depending upon its own age, sex, and genetic background and depending upon the age, sex, and genetic background of another stimulus mouse in the vicinity of the test mouse (164). There are multiple behavioral responses to scent marking. Female urine applied to juvenile mice inhibits adult male aggression toward juveniles (169). When males are conditioned to express low levels of aggression, odors in their urine evoke lower levels of aggression from males and approach responses from females (170). Mice also avoid the urine of conspecifics that are distressed by shock (171) and parasitic infection (167). Interestingly, mice lacking a functional oxytocin gene (that modulates social affect among humans and pair bonding among voles) cannot detect parasitic infections of other mice (148), suggesting the possibility that social motivation can influence the extent that a mouse can derive information from social interactions. Mouse social behaviors are even sensitive to odors that indicate whether another mouse was previously housed in a social or isolate environment (172). Odors of female mice also elicit complex patterns of ultrasonic calls from male conspecifics (173, 174).
Movements and gesture: Visual cues can be useful for mouse social interactions. Visual cues are used in imitation, which has been studied in mice and is highly relevant to autism. In an ethological-relevant experiment, mice were allowed to observe other “demonstrator” mice respond to biting flies. The “observer” mice were then examined for their abilities to learn from their visual observations of demonstrator mice with biting flies. The demonstrator mice were then tested in response to biting flies that were surgically altered so that they were no longer capable of biting. After observation of the demonstrators, observer mice displayed both conditioned hypoalgesia and active burying responses in response to the altered flies. Thus, increased pain tolerance of observer mice in this paradigm was dependent on the experience of demonstrators being bitten. The learned active avoidance (burying) response was contingent on visual detection of a demonstrator actively burying itself in bedding to escape the biting flies (176).
Mice can perform additional imitation tests, such as learning manipulation of a puzzle box (177) and how to swing a door open to obtain a food reward (178). In the latter experiment, male and female mice observed demonstrator mice open a swinging door that was hinged at the top and could swing either to the right or to the left to open. Observer mice showed lower latencies to open the door relative to naïve controls. Further, male mice reliably were able to open the door in the same direction (to the right or to the left) as the demonstrator condition. An opaque barrier was sufficient to block this learning response (178). In these two measures of procedural learning, the ability to detect emotional cues is unlikely to play an important role.
“Emotional contagion” among mice can be expressed when individual members of a dyad are subjected to different levels of paw irritation. Mice express degrees of paw licking that is directly correlated with the concentration of irritant injected into the paw. When two mice are injected with different concentrations of the irritant, they experience different levels of pain and lick themselves at different levels. Importantly, when these individuals are placed adjacent to each other, the response of one of the mice influences the response of the other. This bidirectional response to differences in pain response indicates an ability to adjust individual responses according to the emotional cues of a distressed conspecific (175).
< div class='tao-gold-member'>
Only gold members can continue reading. Log In or Register a > to continue
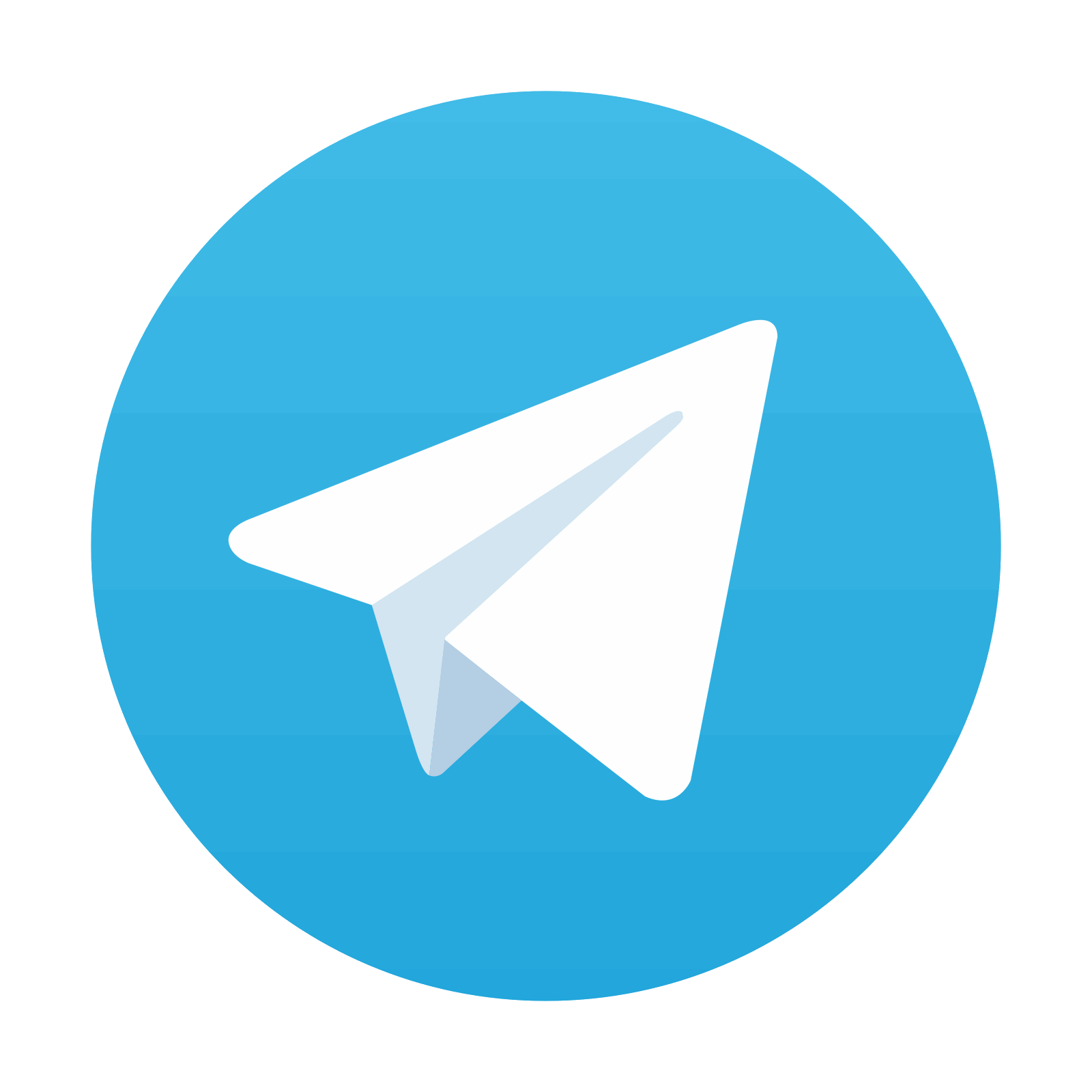
Stay updated, free articles. Join our Telegram channel

Full access? Get Clinical Tree
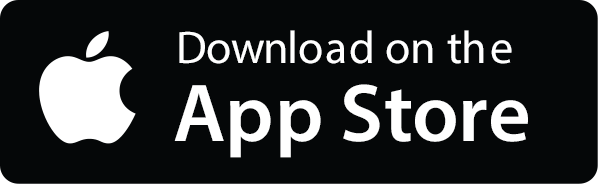
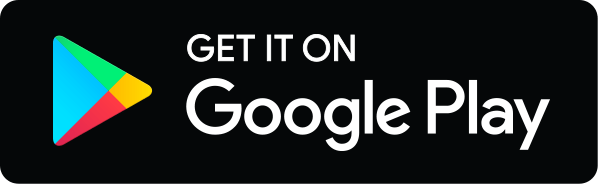