Fig. 5.1.
Schematic illustration of the fear-conditioning chamber and the different experimental phases during delayed auditory fear conditioning. Note that pairing the conditioned stimulus (CS) with the unconditioned stimulus (US) results in gradual increase of freezing during the conditioning phase which can be observed 24 h later during a test phase.
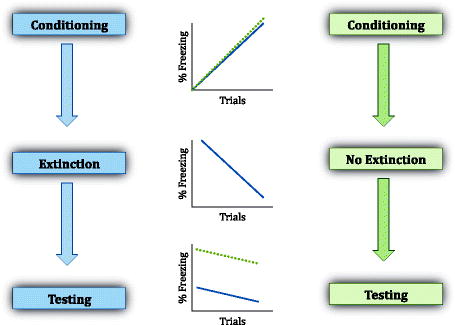
Fig. 5.2.
Schematic illustration of expected behavior of animals undergoing the different phases of fear conditioning, including extinction phase (in which the CS is repeatedly presented in the absence of the US). If extinction training is not to take place, then fear to that CS will remain high during test. The difference in fear responses to the extinguished CS and the unextinguished CS could be referred to as the extinction memory.
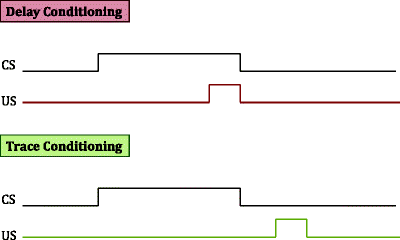
Fig. 5.3.
Schematic illustration of delayed versus trace fear conditioning. In delayed fear conditioning, the US presentation co-terminates with that of the CS. In trace conditioning, the US presentation occurs seconds after the offset of the CS presentation.
Following experimental manipulations (extinction trials, time lapse, surgery, drug administration, etc.), the animals are returned to the startle testing apparatus for the testing phase. This can occur 1 day after training, or even up to a month later, depending on the conditions of the experiment. In this phase, animals are presented with about 60 “startle stimuli,” typically loud bursts of white noise. When a light is used as the CS during training, a pure tone can be used as the startle stimulus (31, 32). Half of the startle stimuli are presented alone and half are presented in concert with the CS. The theory behind fear-potentiated startle is that when a strong association has formed between the CS and US during training, the presence of the CS will produce fear and the animal will exhibit a heightened startle response when the startle stimulus is paired with this CS, compared to when the startle stimulus is presented alone. A larger discrepancy between CS-noise versus noise-alone reflects greater conditioned fear (33, 34). Like cued conditioning, fear-potentiated startle can be used to measure spontaneous recovery and reinstatement. However, renewal tends to be more difficult to establish because the shocks are administered in a different setting than the startle testing apparatus.
2.2 Fear Conditioning in Humans
2.2.1 Early Conditioning Studies
As with cued conditioning in animal studies, human fear conditioning seeks to develop an association between a CS and an aversive US. For example, in an early study of conditioning to social cues investigators used slides of photographs of human facial expressions as CSs and a mild shock to the fingers as the US. Not surprisingly, it was found that stronger conditioning occurred when the reinforced conditioned stimulus was a picture of a fearful face than when it was a happy face (35). (Further discussion of reinforced and non-reinforced conditioned stimuli follows in the next section.) The focus of a considerable amount of the early human conditioning research was directed toward understanding the influence of biological preparedness (35, 36) in the acquisition and extinction of conditioned fear responses. Consequently, many early studies used fearful or angry faces as CSs because they conditioned quickly and without subjecting participants to a large number of, or high intensity, US presentations to achieve a measurable level of fear acquisition (37–39).
2.2.2 The CS, US, and Reinforcement Schedules
Recent studies of human conditioning have trended toward using experimental protocols that more closely follow those established in the animal literature. Commonly, studies use a short (about half a second), mild shock delivered by electrodes to two fingers on the subject’s dominant hand (the non-dominant hand is used for recording physiological measures) (40–42). Other studies deliver the aversive shock to the wrist or ankle of the participant (43). Because of the ethical considerations surrounding shock delivery in human subjects, participants typically predetermine the level of shock to be used as “highly annoying but not painful” (44, 45). Therefore, human fear conditioning in its simplest form involves the association of a CS (normally a colored picture of a geometric shape such as a square or circle) and a mild shock. For example, participants will be seated in front of a computer monitor programmed to display a red circle (the image is typically displayed for about 8–12 s) (46, 47). If the shock is always delivered after presentation of this cue, the subject will quickly learn that it predicts the shock and will demonstrate a conditioned response (e.g., a change in sweat activity) when it appears.
2.2.3 Differential Conditioning
In addition to the CS that is paired with the US, many studies of human conditioning use a second stimulus, such as a blue circle, which is never paired with the US. This type of conditioning is known as differential conditioning and rarely has been used in animal studies. In differential conditioning, the CS that is not paired with the US (e.g., a yellow square) is referred to as the CS− and the CS that is paired with the US (e.g., a blue circle) is referred to as the CS+ (see Fig. 5.4). A typical differential conditioning study might involve several, initial exposures to both the CS+ and the CS− (perhaps 4–5 times each) in random order and with no shocks administered. This “habituation” phase reduces orienting responding and establishes physiological baselines. During the “conditioning” phase, the CS+ and CS− might be presented 5–10 times each with the US being administered immediately following CS+ offset. As with animal conditioning, the duration of time between cues is set to vary around a predetermined average, with an overall range of about 12–25 s for studies using skin conductance change as the measure of fear (48, 49).
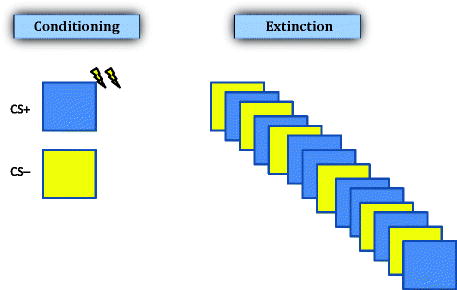
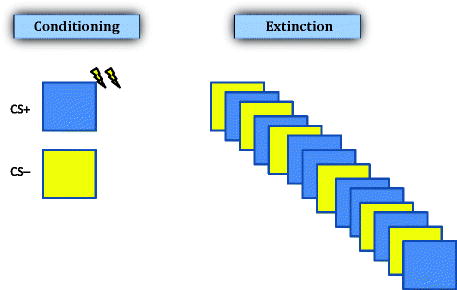
Fig. 5.4.
Schematic illustration of stimuli typically used in human fear conditioning. This type of paradigm is known as differential conditioning, in which two CSs are used: one followed by the US (CS+, dark square in this example), and the other is not followed by the US (CS−, light square). Extinction training in this paradigm involves repeated presentation of both the CS+ and CS− in the absence of the US presentation.
Other types of unconditioned stimuli have also been employed in human conditioning studies. For example, some studies have used aversive noises rather than mild shocks (50, 51). The study designs are similar to those described, commonly using simple visual images as the conditioned stimuli. However, during the fear acquisition phase, a burst of 90–100 dB of white noise will be delivered through headphones for 0.5–2 s as the US (52, 53). Studies that include a CS− may pair the CS− with a non-aversive tone (50–70 dB) with the same duration as the aversive US. Other studies have used small blasts of air directed at the participant’s throat as the US (54–56). This is accomplished by connecting a compressed air tank to plastic tubing with a solenoid valve set up so as to deliver puffs of air for about 100–250 ms, typically at an intensity of about 140 psi (55).
2.2.4 The Index of CR in Humans
Since the 1960s electrodermal measures, e.g., skin resistance, skin potential, and skin conductance, have been the most popular indices of aversive conditioning in humans. Over the years, various general terms have been used to refer to electrodermal activity, such as galvanic skin response (GSR), psychogalvanic reflex (PGR), and electrodermal response (EDR). Currently, skin conductance is the preferred measure of electrodermal activity. Ohm’s Law serves as the underlying principle for measuring skin conductance. Skin conductance level primarily reflects the amount of sweat present in the sweat glands. It is commonly measured from the fingers or palm of the hand; glands on the palms of the hands (and the soles of the feet) are known to be particularly responsive to emotional arousal. An increase in skin conductance is caused by sympathetic nervous system activation, which increases secretion from the eccrine sweat glands. When a human being becomes emotionally aroused, he or she produces small amounts of sweat that are more electrically conductive than dry skin. Skin conductance is measured by attaching two electrodes, separated by about 14 mm, on the subject’s non-dominant hand. A skin conductance coupler then passes a small electrical current at a constant voltage (typically, 0.5 V) through electrodes filled with isotonic paste. An analog-to-digital converter “digitizes” the voltage output of the skin conductance coupler, which is then recorded and translated into a numeric measure of skin conductance level by a computer program. A change in skin conductance level can then be calculated so as to provide a measure of the magnitude of the skin conductance response to a given stimulus. For example, one might calculate the average skin conductance level during the 1-s interval prior to onset of a CS and subtract this value from the peak skin conductance level during the CS interval. This change score would provide a measure of skin conductance response to the CS presentation. For an excellent overview and discussion of the electrodermal system and assessment of electrodermal activity, the reader is referred to Dawson et al. (57). In addition to skin conductance, some investigators record other physiological indices such as heart rate and facial electromyogram (EMG) of the corrugator muscle, a small muscle at the medial end of the eyebrow that is associated with furrowing of the eyebrows or frowning.
The human startle response is commonly measured from eye-blink-related activity using electromyography (EMG) of the right orbicularis oculi (e.g., 55, 58), the muscle that controls closing of the eyelids. To do so, Ag/AgCl electrodes filled with electrolyte gel are positioned about 1 cm under the pupil and 1 cm below the lateral canthus (the corner of the eye where the upper and lower lids meet). A reference electrode is then placed on the upper forehead, on the participant’s arm, or behind one ear over the jawbone. EMG activity is amplified and recorded using hardware and computer software specifically designed to sample EMG activity at 1,000 Hz beginning at startle stimulus onset (or just prior to) and ending a few hundred milliseconds after stimulus onset.
Several studies have used heart rate (HR) as a measure of conditioned responding (e.g., 44). This can be done by electrocardiogram (ECG or EKG), which measures electrical impulses associated with contraction of the myocardial muscles of the heart. The ECG is easily measured using surface electrodes placed on particular areas of the body (e.g., chest, arms, legs). ECG output appears in the form of a voltage between electrode pairs. The amount of time between heart beats, recorded in milliseconds between successive R wave components of the ECG, can be converted to a measure of heart rate. Some studies have monitored blood pressure during conditioning paradigms (59–61). Blood pressure is monitored using a sphygmomanometer, or blood pressure monitor, which displays pressure in terms of mmHg, or millimeters of mercury, based on the original devices that used the height of a column of mercury to deduce circulating pressure. Whereas skin conductance, EMG, and heart rate measurements can be continuously and unobtrusively obtained, the need to inflate and deflate a blood pressure cuff makes blood pressure a more intrusive and difficult measure.
3 Notes
This chapter is intended to introduce the reader to methodologies used in rodent and human experiments that target various aspects of fear conditioning. Though the principles are similar, choices regarding the type, duration, and number of CSs and USs vary substantially across studies. In addition, the number of days and the implementation of additional test phases (e.g., extinction, retention, or renewal) vary depending on the scientific question(s) being addressed. In this section of the chapter, we discuss some additional and important considerations regarding the many variants of the classical fear-conditioning paradigm.
3.1 Freezing Measurements
In the early years of fear-conditioning research, freezing behavior was always scored manually. Manual scoring is a procedure whereby the entire experimental session is videotaped; the investigator later reviews the tape and meticulously scores freezing behavior with a stopwatch. The number of seconds the animal spends motionless will be recorded, usually as a percentage score. For context conditioning, the percentage would be referenced to the total time spent in the chamber (∼3 min); for cued conditioning, it would be referenced to the duration of the cue. Often, if the cue is a tone, a light will be set up that is invisible to the animal, but serves to indicate the presence of the CS to the scorer. As previously mentioned, investigators using cued conditioning may also record freezing before the onset of the CS to confirm that the behavior is, at least in part, associative in nature.
Manual scoring requires a considerable amount of time and relies on subjective judgments of the experimenter. To address these issues, some labs will randomly select a subset of sessions and have a different researcher re-score them in order to corroborate the values. Some labs will require that two different researchers score every session and use the average of the raters’ scores. Although the methods of managing human error may vary among labs, comparisons within each lab will not be tainted as long as the scoring methods remain consistent over time. An additional limitation of manual scoring arises from experimenter bias, a tendency (usually subconscious or unintentional) to score or make judgments that favor an expected or hoped for result. Researchers attempt to control this bias by conducting blind experiments, in which the individual doing the scoring is not informed as to the experimental condition of the animals. The scorer is thereby unable to form a specific prediction regarding the expected behavior for a particular animal.
In recent years, computer software has been developed to score freezing automatically. As with manual scoring, the session is recorded and incorporates an indicator light, invisible to the animal, for signaling CS onset during cued conditioning. Motion-sensing software is then able to calculate how much time the animal spends completely still during the cue or session. An important drawback to this approach is that not all absence of motion is attributable to fear behavior, especially when operant conditioning is not used in conjunction with fear conditioning. It can be argued that human input is necessary to discriminate true freezing from incidental pauses in movement. However, introducing these subjective determinations is done at the expense of increasing variability between scorers. In general, automated scoring programs are becoming more popular in labs that study fear conditioning because they remove the elements of human error and experimenter bias and are much more time-efficient.
3.2 Floor and Ceiling Effects
As explained above, freezing scores during cued conditioning are often recorded as a percentage of the overall presentation time for the CS. For example, if an animal freezes for 24 s during a 30-s long tone presentation, it is said to exhibit 80% freezing for that trial. However, if the animals are consistently freezing for the entire duration of the cue (100% freezing for each trial), there is the risk of ceiling effects. Ceiling effects occur when variations in freezing time predominantly occur at time points beyond the CS presentation, thereby rendering such trials as unscorable by traditional methods. For example, if after conditioning an animal freezes for 45 s following the onset of a 30-s CS, this will be scored as 100% freezing because it was motionless for the entire duration of the cue (and longer). If a subsequent extinction procedure or drug manipulation reduces the animal’s freeze time to 30 s, a 33% reduction, it will again be scored as 100% freezing and it will appear as though the intervention had no effect whatsoever.
Floor effects, on the other hand, tend to occur at the end of extinction trials or after a pharmaceutical manipulation that reduces fear behavior nearly to zero. In such cases, it is difficult or impossible to decipher the relative effects of two different experimental conditions, because both groups repeatedly display little or no freezing and are therefore said to have reached the “floor” level of fear behavior. Ceiling and floor effects can typically be avoided by selecting the proper conditioning paradigm and conditioning parameters, as outlined in this chapter and as is well documented in the conditioning literature. For example, ceiling effects might be the result of “over conditioning,” which could be corrected by reducing the frequency or intensity of the US. Alternatively, floor effects might be alleviated by reducing the number of extinction trials.
3.3 Context Conditioning
In some studies it is important to control for or measure the portion of the fear response that may be attributable to conditioning to contextual cues. This can be done by scoring fear behavior as a difference, rather than an absolute value. In other words, fear can be measured before the CS, i.e., when only the context is present, and after the CS presentation during the testing phase; a difference is then calculated that essentially removes the influence of fear associated with the context. Another way to control or measure the effects of context is to perform conditioning in one context and then test animals in either the same context or a novel one. If those tested in the novel environment express less-conditioned responding than those tested in the original environment, contextual conditioning is thought to be represented by difference between those groups.
The role of contextual stimuli on conditioned fear remains unclear. Several studies have found that testing in a context different from the one in which conditioning occurred causes very little, if any, disruption of conditioned responding (4, 62–64). In fact, one study found that the switch to a new context actually enhanced, rather than inhibited, conditioned responding to the CS (65). For this reason, there is little agreement as to how context should be regarded. On one hand, the Rescorla–Wagner model treats it merely as a second CS that is presented in concert with the intended CS (25). On the other hand, it has been suggested that contexts carry information about the relationships between events (i.e., CS–US pairings) that occur within them, but do not hold specific associations themselves.
3.3.1 Post-conditioning Tests
The conditioned responses that are triggered by a CS–US association can be diminished after repeated presentations of the CS in the absence of the US, a process known as extinction. Following conditioning, either the same day or the following day, fear extinction is produced by means of systematic presentations of the CS without the US, which gradually extinguishes the CR. While the amount of time between conditioning and extinction has not been standardized, long-term extinction memory is thought to develop only when extinction training occurs at least 6 h after conditioning (66). The number of extinction trials can vary greatly between studies. Sometimes there are fewer extinction trials than conditioning trials (9) and other times (6) the number of extinction trials exceeds that for conditioning. Contextual fear can be extinguished simply by repeatedly returning the animal to the chamber in the absence of shock. Typically, an extinction session will occur over about the same amount of time as the conditioning session (∼3 min) and must be repeated over a number of days in order for there to be an enduring reduction in fear behavior. As noted previously, there are several different fear memory-related phenomena that can be tested. The simplest test phase would involve a single CS or startle presentation following extinction, surgery, drug administration, or other experimental manipulations specific to a given study. Depending on the goal and limitations of the study, testing could occur as soon as an hour or as long as a month after conditioning/extinction.
Spontaneous recovery is the return of a previously extinguished CR that requires no experimental procedure aside from the passage of time. Reinstatement typically involves one to three US presentations and is tested the following day by presenting the previously extinguished CS (67). Renewal is tested when the CS–US pairings occur in one context and the CS is subsequently extinguished, i.e., presented alone, in another context. Returning the animal to the original context during testing will produce a renewed fear response. Renewal can also be observed when the animal is tested in a third, neutral context (3).
3.4 Combining Fear-Conditioning Paradigms with Operant Conditioning Procedures
In some fear-conditioning experiments, researchers will also include an operant conditioning procedure, whereby animals are trained to press a metal bar for a food reward repeatedly during the conditioning procedure. In order to establish a consistent motivation for a food reward, animals are fed systematically by investigators such that they are kept at about 80% of their original body weight. The classic approach is based on the method of successive approximations first demonstrated with pigeons by B.F. Skinner in 1937. Also referred to as “auto-shaping,” this method is executed by first manually delivering a food reward whenever the animal walks in the vicinity of the metal bar. As Skinner describes in The Behavior of Organisms, the investigator will subsequently deliver reward for “each of the following steps in succession: approaching the site of the lever, lifting the nose in the air toward the lever, lifting the fore-part of the body into the air, touching the lever with the feet, and pressing the lever downward.” (68). Throughout this process the rat gradually learns which actions will produce a reward, thereby increasing the frequency of these actions and demonstrating operant conditioning. After about 10 days of training, most rats will reach a level at which they press the bar very consistently even when pellets are not delivered after each press. Rather, pellets are delivered at a rate predetermined by a computer program to vary at an interval of about 60 s. Most investigators will exclude rats from their experiments that do not meet this standard. Alternative training methods have been proposed. One such method involves shining a photocell beam onto the Plexiglass bar and delivering the reward when the animal interrupts the light beam with its body (69). Though some methods have claimed to expedite the learning process, most studies continue to rely on Skinner’s classic method.
3.5 Why Use Operant Conditioning in Conjunction with Fear Conditioning?
There are two important reasons as to why an investigator might decide to train rats to press a bar for food while undergoing fear conditioning. First, this approach allows bar-press suppression to be used as a measure of fear, in addition to the classical measure of fear, freezing. Press-rate suppression has the benefit of providing a relative measure of fear. In other words, because the ratio is calculated by comparing conditions before and after the onset of the conditioned stimulus, a stronger argument can be made that changes in behavior result from the CS onset. Secondly, bar-pressing behavior serves to provide a consistent level of locomotion during the experiment, against which freezing can be measured. If the rodent does not have incentive to move about the chamber, it is more difficult to attribute a lack of motion as representing fear-induced freezing. This is not likely to be a problem for studies only examining the conditioning phase (fear learning). However, if the experiment will include additional training sessions that examine fear extinction, bar-press training may be useful. Extinction training requires many CS trials (15–20 trials) in the absence of the US and if the duration of the inter-trial interval is long, the animals may fall asleep. Implementing bar-press training protects against the possibility that the animal might fall asleep. However, including bar-press training carries the disadvantage of introducing the possibility that motivation for reward may compete with the fear-circuitry behavior. This makes it challenging to compare results from studies that include bar-press training with those that do not.
3.6 Fear Conditioning in Humans
While the US always follows the CS presentation in rodents, this is not necessarily the case in human studies (70–72). Reinforcement schedules may be either continuous, whereby the US follows each CS+ presentation, or partial (or intermittent), whereby only some CS+ presentations are paired with the US. When partial reinforcement is used, the participant learns that a shock never will be received following the CS−. However, there is less certainty associated with the CS+ as to whether or not a shock will occur. In other words, the CS+ will always be present before every US administration, but a US does not necessarily follow the CS+. The introduction of the uncertainty component makes partial reinforcement more resistant to extinction than continuous reinforcement, even though continuous reinforcement might involve a greater number of CS–US pairings overall. The US presentations can be administered on a fixed or random schedule. A fixed schedule would mean a predetermined ratio; for example, a US presentation for every third CS+ presentation. With partial reinforcement, random schedules produce conditioned responses that are more resistant to extinction than fixed schedules.
< div class='tao-gold-member'>
Only gold members can continue reading. Log In or Register a > to continue
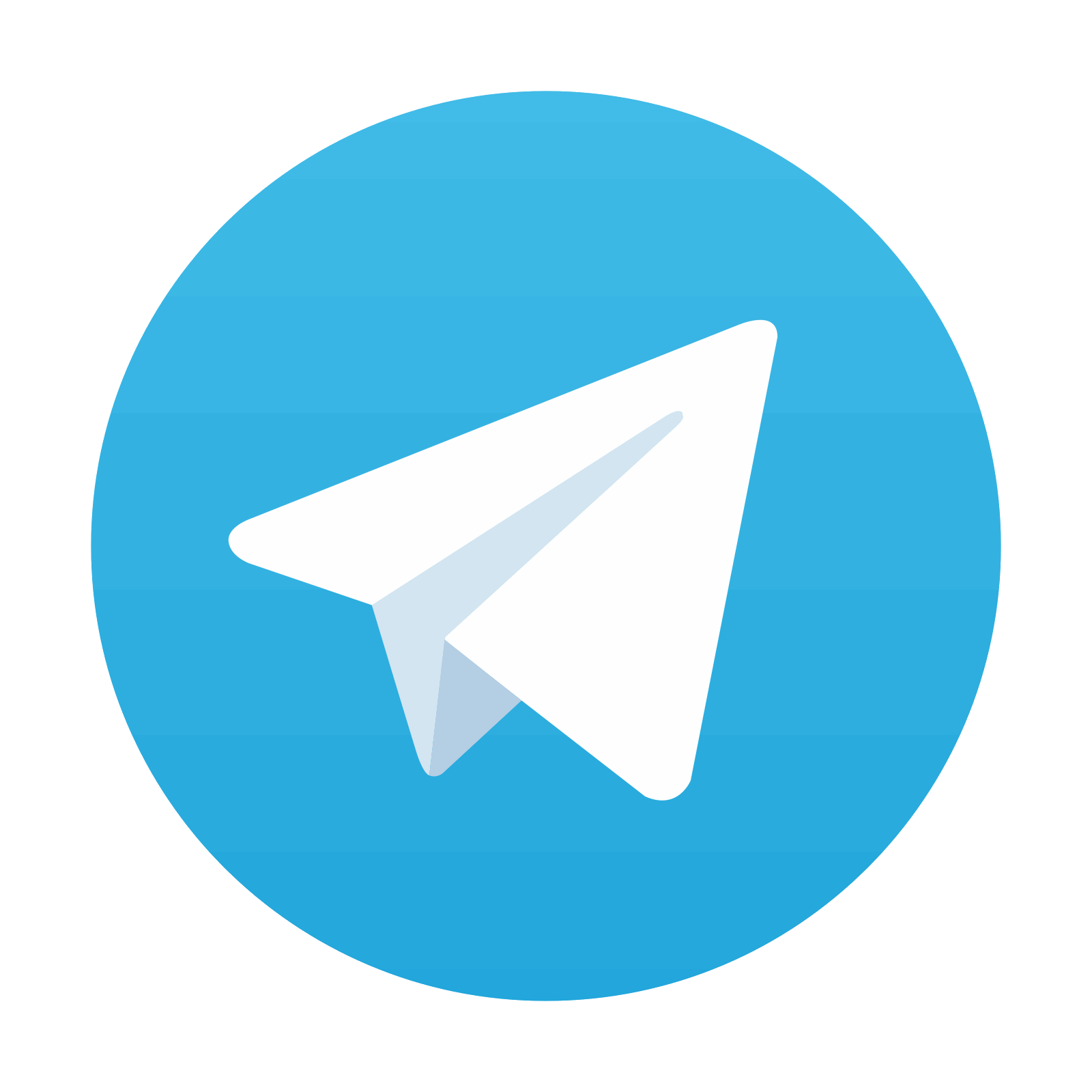
Stay updated, free articles. Join our Telegram channel

Full access? Get Clinical Tree
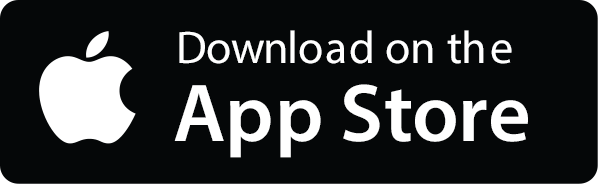
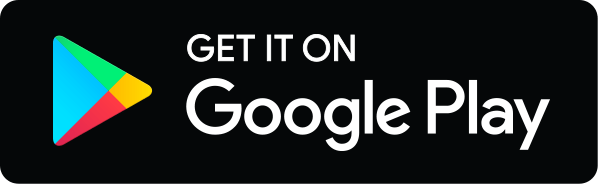