Chapter 49 The embryo forms from a single cell that gives rise to cells that have an increasingly narrower spectrum of differentiation.10 The mesenchymal stem cell is a stage along this path of differentiation by which the cell can follow osteoblastic, chondroblastic, adipocytic, tenocytic, or myoblastic lineages (Figure 49-1). A population of these stem cells is preserved in the adult in different tissues; the most accessible sources include the cambium layer of the periosteum, the bone marrow, and fat. Although the number of stem cells diminishes with age, stem cells are present throughout life and can be retrieved, isolated, and culture expanded. Mesenchymal stem cells can be manipulated in vitro or in vivo to follow osteoblastic lineages. Transforming growth factor-beta (TGF-β), of the transforming growth factor superfamily, is ubiquitous in the embryo and adult, but is especially common in bone, platelets, and cartilage. This growth factor can be expressed in different cells during bone healing, and therefore is thought to play roles throughout the entire healing process. Studies in several animal models, using several isoforms and doses of TGF-β, have resulted in contradictory and inconsistent conclusions on its ability to enhance bone healing.16,35,44 The dosing regimens needed are high, and frequent or continuous. In addition, this nonspecific growth factor does have an influence on several cell types, thereby confounding investigations and potential influences on different local and distant cell tissues. Also of the transforming growth factor superfamily, the many bone morphogenetic proteins (BMPs) have been discovered as have their roles in bone formation and healing studies.27,51,63 Most highly studied are BMP-2, -4, and -7.19,27,52 These have similar receptor affinity and cell-signaling mechanisms. Mice embryos deficient of these bone morphogenetic proteins often are not viable, or have various skeletal, skull, and kidney malformations.34 Originally isolated from demineralized bone, this protein was shown to cause ectopic new bone formation. Later, the human genetic sequence was defined for the various bone morphogenetic protein isoforms.67 From the human genetic codes, two isoforms have been most widely investigated, specially recombinant human BMP-2 (rhBMP-2), and recombinant human BMP-7, also known as recombinant human osteogenic protein-1, or rhOP-1. Recombinant human BMP-2 has been studied extensively in dog models for preclinical human applications such as treatment of segmental bone defects, nonunion, and spinal fusion.27 Information on dosage and carriers in these studies has helped define efficacious methods in veterinary medicine. Several studies used doses in the range of less than 1 mg. For example, in a study of 25-mm defects of the radius, subjects were treated with 0.15 mg, 0.6 mg, or 2.4 mg of rhBMP-2.52 Although each dosage was efficacious, biomechanical parameters of the healed bone were highest in the 0.15-mg dose, and cystlike lesions were noted with higher doses. Equally important as the dose is the carrier. Several carriers have been utilized, and several (including polylactic-polyglycolic acid carriers) were technically difficult to use; others resulted in inflammatory reactions and bony voids within the healed bone. Carriers that have been most efficacious include absorbable collagen sponge and some types of calcium phosphate.27 Bone morphogenetic proteins are soluble and are rapidly cleared from a local environment if not in a carrier that attenuates clearance. Some injectable carriers that are being investigated include proteins and calcium phosphate paste.7 Although not a specific entity, but a family of several structurally related polypeptides, the fibroblast growth factors (FGFs) are known to play a role in embryologic development and bone healing. They have a critical role in angiogenesis and proliferation of mesenchymal stem cells. The two most abundant in the adult are FGF-1 and FGF-2. Not only are these growth factors involved with mesenchymal stem cells and the osteoblastic lineage of cells, they are also involved with epithelial cells, myocytes, and chondrocytes. Mutations in fibroblast growth factor lead to defects in endochondral ossification and intramembranous ossification, including achondroplasia. As such, it has been postulated that the fibroblast growth factors are active in early stages of fracture healing and angiogenesis, and therefore may enhance the initiation of fracture repair.25,42 Insulin-like growth factor (IGF) is critical in skeletal development. Growth hormone–releasing hormone is produced by the hypothalamus and causes the production of growth hormone by the anterior lobe of the pituitary gland. Growth hormone travels via the circulation to the physis of bones, where target cells are stimulated to release insulin-like growth factor.32 Insulin-like growth factor is needed along with growth hormone to facilitate bone and limb lengthening, and insulin-like growth factor is thought to act locally to encourage cellular proliferation. As an integral part of bone lengthening and growth, this growth factor has been investigated as used alone or with other hormone and growth factors in bone healing.25 Although insulin-like growth factor seems to be active in bone healing, no significant evidence indicates that speed of exogenous supplementation influences bone healing or regeneration of long bones. Platelet-derived growth factor (PDGF) is released by the alpha granules of platelets, and because a fracture clot contains activated callus, which may aid in initiating fracture healing, its role in fracture healing has been speculated, but not clearly defined. Exogenous application of platelet-derived growth has been investigated in several animal studies, some suggesting a degree of efficacy.43 In addition, the combined use of insulin-like growth factor and platelet-derived growth has been investigated, but clear efficacy in dogs or human beings has not been reported. Gene therapy is the introduction of genetic material via noncellular or cellular material. The goal is to introduce a gene into cells in a local environment where bone is intended to be regenerated.46 The gene is then intended to be expressed by cells, and the cells produce osteogenic proteins, such as bone morphogenetic protein.6 Genetic material can be introduced directly into the area, then can be translocated into the nucleus of the cells by physical mechanisms such as electric pulsed or ultrasonic waves. Alternatively, viral vectors containing the desired genetic material are introduced into the area, and the virus is used to transport the gene into the cell and facilitate its expression. Adenovirus vectors encoding for bone morphogenetic protein genes have been used.33,34 These techniques have been investigated in fracture repair models in rodents with some success. However, concern has been expressed regarding the safety of this delivery method, and research is still in early stages for this technology.26 A material or physical impetus that results in enhancement of regenerating bone is termed osteopromotive. Osteopromotion can function at various stages during bone healing and can provide different stimulatory signals to bone-regenerating tissues. Osteopromotion differs from osteogenesis and osteoconduction in that bone formation is enhanced without cells or a scaffold; however, osteopromotive stimuli alone cannot induce bone formation. Osteopromotion can be achieved by introduction of substances or materials that enhance bone regeneration, or by physical or mechanical strategies that induce proliferation and differentiation of mesenchymal stem cells and their progeny. The best example of an osteopromotive substance is platelet-rich plasma.2 Still recognized as the gold standard, autogenous cancellous bone grafting remains the mainstay of bone grafting, especially in veterinary medicine. This graft, taken from the host, usually at the time of surgery, uses all of the strategies of bone regeneration. Although the number of cells within a bone graft may be limited, the trabeculae are lined with osteoblasts that provide osteogenesis under the influence of local cytokines. The disrupted bone matrix of the cancellous bone releases an entire milieu of cytokines and growth factors from the extracellular matrix; these substances are osteoinductive. A properly harvested graft maintains a structural scaffold that serves as a special conduit on which new bone can form; this is osteoconduction. In addition, hemorrhage and the resulting clot contain activated platelets, and hence growth factors from the released platelet alpha granules, which contain IGF-1, PDGF, and TGF-β, serving as an osteopromotive function.8,15 Autogenous cancellous bone collection is well tolerated in veterinary patients, unlike in human beings, for whom pain at the donor site can persist for months or years. The most common donor sites are the proximal humerus and the wing of the ilium. These sites are popular because of the volume of graft material obtained from the proximal humerus, and because no consequence of iatrogenic fracture occurs during harvesting from the wing of the ilium. Common but less popular donor sites include the proximomedial region of the tibia, the subtrochanteric region of the femur, and the condyles of the femur. Other sites used less frequently include the caudoventral portion of the mandible (for periodontal surgery)56 and the rib. Complications associated with harvesting of cancellous bone graft are rare in dogs, but fracture and growth plate closure have been reported.18,47 Restoration of cancellous bone at the donor site has been evaluated. Restoration occurs more completely in the proximal humerus than in the proximal tibia,47 and is sufficient for repeat grafting by 8 weeks in the proximal humerus47 and by 12 weeks in the proximal tibia.23 The donor site in the proximal humerus is the greater tubercle, and often the entire limb is prepared with a hanging leg prep, or at least is made accessible to the surgeon, so the limb can be grasped, stabilized, or manipulated to help in the procedure (Figure 49-2). The greater tubercle is palpated and a surgical approach made at the level of the tendon of insertion of the infraspinatus muscle. The approach is maintained with two self-retaining retractors, often Gelpi retractors, at orthogonal angles. The retractors should be placed as deep as possible, often between bone and periosteum or other deep soft tissue, to help with exposure, and so that cancellous bone particles are not lost in soft tissues. The lateral cortex is usually penetrated with a Steinmann pin or drill. A curette, usually a Brun or Spratt Volkmann type, is chosen on the basis of the size of the patient. The optimal size of the cancellous particles is between 3 and 6 mm, so a curette of comparable size would be approximately a #000 to a #2. The cancellous bone is scooped from the proximal humerus. The surgeon should try to remove particles rather than macerating the cancellous bone. A very convenient place to store the graft is in the barrel of a 5- or 10-mL syringe (Figure 49-3). The graft material is placed along the plunger, which keeps the graft material moist and protected. The blood will clot, forming wedge-shaped sections of graft material; having the graft material in this form is often convenient for placing the graft into a recipient bed. The graft should be used as soon after harvest as possible, as viable cell numbers will sharply decline even with optimal conditions. If the graft is to be stored for any length of time, it should be placed in blood or saline, as drying will significantly lower cell viability in as little as 2 hours.30,37 Allogeneic bone has both osteoinductive and osteoconductive properties and capabilities. Compared with autograft bone, however, it lacks the viable osteogenic cells that are typically derived from marrow. Bone is designed to heal by having growth factors that are dispersed throughout the collagen matrix, which underlies the mineral content. Urist’s landmark publication describing bone morphogenetic proteins serves as the basis for our current understanding of the complex hidden cascade of multiple growth factors that can be exposed through bone trauma and subsequent osteoclast activation.63 With its naturally occurring bone morphogenetic proteins exposed through demineralization, allograft can facilitate induction of bone growth through these same mechanisms. Modern bone allograft processing is designed to retain a full range of acid-stable bone morphogenetic proteins. In its cancellous form, allograft provides an osteoconductive scaffold that provides osteoblasts an ideal place to migrate. No shortage of allograft bone occurs, as may be seen in autograft; nor does allograft bone have the associated morbidity risks involved with autograft. It does, however, come with some risks of its own, including a small risk of disease transmission and remote concerns about histocompatibility issues. Histocompatibility issues are ameliorated by most processing methods for bone grafts. Viable cellular elements that can evoke an immunogenic reaction are periosteum, muscle, blood cells, and marrow.22 Immunogenic cellular elements are typically cell surface histocompatibility markers that would stimulate a cellular (rather than humoral) immune response in a living, viable allograft or xenograft; these are removed by current processing methods, which include scraping of periosteum, purging of marrow elements such as lipids, freeze-thawing, freeze-drying, and treatment with acids and other agents, as well as irradiation.13 Fortunately, bone morphogenetic proteins are acid-stable, and the processing methods mentioned previously have been shown to reduce or remove immunogenicity while preserving embedded bone morphogenetic proteins.27 Transplanting bone that is fresh and unprocessed stimulates a significant immune response in the host.13,22 This can cause complications such as rejection of the graft and resorption of the graft. In contrast to viable organ transplants, which require matching and immunosuppression, and blood transfusion, which at least requires group matching, matching for allograft bone is not necessary; in fact, it is not a consideration for bone allograft choice in human orthopedic or periodontic surgery, and tissue bank standards do not require gathering of this information or association with bone allografts.1,21 Although it is occasionally postulated that allograft bone carries risk of disease transmission, over the past two decades more than 10 million human musculoskeletal (bone, osteochondral, bone-tendon, and bone-ligament) allografts have been safely transplanted in the United States.39 Extremely few instances (approximately 35 among 10 million graft cases) of disease transmission were reported during that time. Diseases transmitted include bacterial disease, hepatitis, rabies, and human immunodeficiency virus (HIV). Since the first report of transmission of HIV (in 1988; note this was before HIV antibody testing was required), vigilance for transmission of disease has been very high. Each tissue bank is required to trace every allograft produced to the consignee.1 No cases of disease transmission using processed (e.g., freeze-dried and/or demineralized) bone products have been reported during the past 30 years. Since 1996, when commercially available allograft animal bone was first offered to veterinarians, no cases of disease transmission have been reported. The American Association of Tissue Banks (AATB) is the national standard-setting organization for tissue banking processes. This organization has been at the forefront of setting standards for the banking of tissues for 25 years.1 Consequently, techniques involved in donor screening, tissue processing and preservation, quality control, and traceability have evolved significantly. The AATB requires rigorous donor health history screening and behavioral risk assessments, as well as infectious disease testing and microbiologic cultures of final products. It also requires specific organizational structures, an extensive quality control/quality assurance program, and process validation studies. It specifies how tissues should be procured and processed and the types of documentation required.1 In the United States, commercial veterinary tissue bankers adhere where applicable to the standards established by the AATB. As in any sterile surgical procedure, aseptic processing should restrict or minimize contamination of tissue. Immediately upon procurement, each bone is thoroughly cultured, wrapped in barrier wrappings, sealed, labeled, and placed in a −70° C quarantine freezer, pending return of 14 day aerobic and anaerobic microbiology cultures. Any tissues with unacceptable organisms are moved to a discard or research-only inventory. Acceptable tissues are processed further to remove any soft tissue, including periosteum and cartilage; bone is then processed into individual graft types, including whole bone and large shaft sections for limb-sparing and buttressing applications, blocks or short cortical sections for cervical spinal fusions (Figure 49-4), and large bone loss settings; fascia and tendon allografts may also be processed, and morselized bone is further processed to make cancellous chips and cortical bone powder for demineralization.5 Cortical bone powders are sieved to specific particle sizes, depending on the final type of graft material desired (e.g., 125 to 1180 microns). All bone grafts are then purged to remove marrow, lipids, and other immunogenic elements. Further treatment of grafts may involve the application of antibiotic washes. The bone may be freeze-dried or frozen. Bulk graft materials are cultured again to ensure that they have not been contaminated during processing. Upon receipt of a 14 day negative in-process culture, tissues are final packaged and cultured once more. Final cultures are taken of the graft in its final packaging, and the swab is representative of the graft material, as well as the packaging that will be on the sterile field. Bulk cultures of the bone are also taken during packaging. All final packaging cultures are bacteriostasis/fungistasis-validated, U.S. Pharmacopeia (USP) sterility cultures (14 days, two different media, two temperatures). Final packaged grafts include a package insert to facilitate understanding of use and handling requirements by clinicians and their staff, as well as a transplant record and extra labels to facilitate tracking of patients in clinic medical records and to provide the return link for the tissue bank that links grafts to actual recipients. The final release of grafts occurs when all processing documents have been reviewed and found to be within the written specifications of the bank’s standard operating procedures. Frozen grafts are preserved at a storage temperature of −70° C. Freeze-dried grafts may be irradiated with gamma irradiation to facilitate room temperature storage.
Bone Grafts and Substitutes
Mesenchymal Stem Cells
Growth Factors
Transforming Growth Factor-Beta
Bone Morphogenetic Protein
Fibroblast Growth Factor
Insulin-Like Growth Factors
Platelet-Derived Growth Factor
Gene Therapy
Clinical Application of Bone Graft Techniques
Osteopromotion
Autogenous Cancellous Bone Grafts
Allograft-Based Bone Graft Substitutes
< div class='tao-gold-member'>
Stay updated, free articles. Join our Telegram channel

Full access? Get Clinical Tree
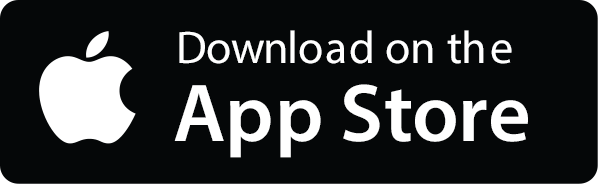
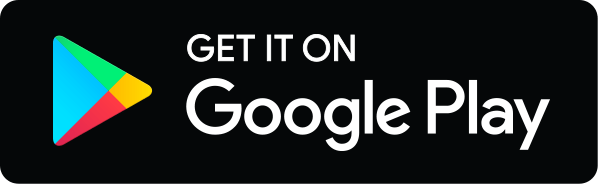