The level of conditioning of a dog constantly changes any time the body performs at a specific metabolic level proportionate to its current adaptation to physical stress (Figure 8-1). The systems of the body are conditioned to maintain homeostasis at this level. If the demands placed on the body increase over time, the body adapts and conditions itself to maintain homeostasis at this new level. An understanding of the physiologic changes involved in this process helps in the development of programs that allow regulation of the conditioning or reconditioning of the body. These programs may be used to condition the entire body or to focus on individual body segments. In physical rehabilitation, programs may be developed to treat injuries, to stimulate healing of the injuries, and to enhance reconditioning of the injured segment. Because of the biomechanical forces acting on the body during the reparative phases of healing, certain segments may become stronger than other segments. Exercise programs should be implemented to remedy any conditioning imbalances of the body. The basic goals should be to allow the tissues to heal, then to recondition the tissues so that they can accept predetermined workloads, and finally to recondition the entire body to balance any conditioning deficits. The circulatory system carries various oxygen, substrates, and chemical messengers; removes carbon dioxide and metabolic byproducts; assists with thermoregulation; and is an important mediator for acid-base balance.1 Blood consists of fluid and cellular components. Blood plasma is the liquid portion of blood and consists of water, proteins, electrolytes, dissolved gases, and nutrients.1 The cellular constituents consist of erythrocytes, leukocytes, and platelets. Oxygen and carbon dioxide (discussed in the next section) dissolve to a limited extent in blood plasma, but are largely carried bound to hemoglobin found in erythrocytes. The heart serves to pump blood to the pulmonary and peripheral circulation. Resting heart rate is approximately 80 beats per minute for a large dog.1 In response to exercise, heart rate and myocardial contractility increase, which concomitantly increases cardiac output. A large dog typically has increases of 30% for stroke volume, 300% for heart rate, and approximately 400% for cardiac output during vigorous exercise when compared with rest.1 Actin and myosin are two types of protein chains in the sarcomere. They interact as a result of enzymatic and chemical reactions to produce muscle contraction. Calcium and phosphate are chemical components involved with the production of muscle contractions. Phosphate is in the form of adenosine triphosphate (ATP). ATP binds to a receptor site at the end of the myosin leverage arm. The actin filament includes troponin, which is bound to strands of tropomyosin. A calcium ion attaches to the troponin molecule, which changes the shape of the tropomyosin. This action opens a myosin-specific binding site on the actin protein chain. Energy is emitted when ATP releases a phosphate ion, producing adenosine diphosphate (ADP). The resultant energy allows the ADP to create a bond between the open binding site on the actin filament and the myosin leverage arm. This bond changes the myosin structure, allowing leverage to produce a contraction between the two fibers. The ADP is released and the lever arm is freed. Energy is then required to add a phosphate group to the ADP, re-creating ATP, which is then used for further contractions (Figure 8-2). The accumulated contractions of the muscle fibers create contraction of an entire muscle. The glycolytic pathway provides energy from 5 to 20 seconds up to 2 minutes as a result of the anaerobic breakdown of glucose. This is a more complex form of energy production, using multiple enzymes and reactions. In the first phase of glycolysis, a glucose molecule enters the cell where the enzyme hexokinase adds a phosphate group to the glucose molecule, which creates glucose 6-phosphate (G6P). G6P then enters a series of reactions to produce fructose 1,6-biphosphate. One of the reactions involves the enzyme phosphofructokinase, which adds another phosphate to the molecule. As a result, two ATP molecules are used in phase 1. In the first reaction of the second phase of the glycolytic pathway, fructose 1,6-biphosphate is converted into two three-carbon molecules. Glyceraldehyde 3-phosphate is then phosphorylated and oxidized, which releases two hydrogen molecules and two electrons. The two electrons and one hydrogen molecule combine with nicotinamide adenine dinucleotide (NAD−) to form NADH, which can be used in oxidative metabolism. The four remaining reactions of phase 2 result in the production of two ATPs. Combined with two ATPs from the other three-carbon chain, this results in a total of four ATPs produced in phase 2. The net result of the glycolytic pathway is the production of pyruvate and two ATPs that may be used as energy (Figure 8-3).
Basics of Exercise Physiology
Figure 8-1 Work capacity increases when physical stress increases and adequate time allows for adaptation.
Cardiopulmonary Physiology
Muscle Physiology
Muscle Energy Systems
Figure 8-3 Glycolytic pathway with production of pyruvate and adenosine triphosphate (ATP). ADP, Adenosine diphosphate; NADH, nicotinamide adenine dinucleotide, reduced.
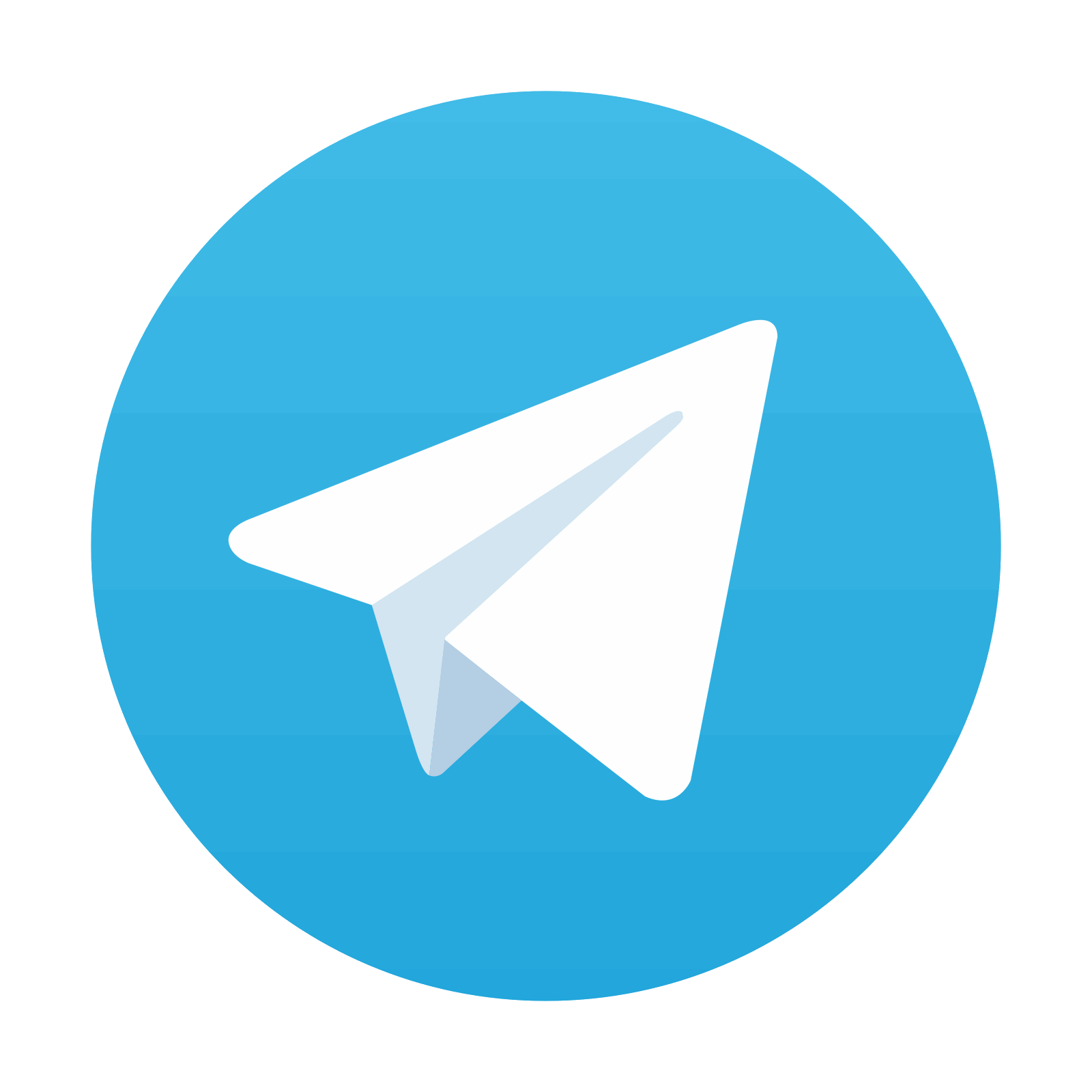