The authors would like to acknowledge Janna Johnson for her work on the previous edition. Electrical stimulation (ES) is a commonly used modality in physical therapy, which is effective for many purposes, including increasing muscle strength, muscle reeducation, increasing range of motion (ROM), correction of structural abnormalities, improving muscle tone, enhancing function, pain control, accelerating wound healing, edema reduction, muscle spasm reduction, and enhancing transdermal administration of medication (iontophoresis).1 Terminology associated with electrical stimulation can be confusing, and, in 1990, a committee of the electrophysiologic section of the American Physical Therapy Association (APTA) developed the Standards of Electrotherapeutic Terminology, a document created to unify and standardize the terms and definitions used by biomedical engineers, researchers, educators, and clinicians. Historically, electrical stimulators and the terminology related to them have been referred to by their specific rehabilitative application, the use of the inventor’s name, or the commercial companies that produced them. Examples include galvanic current, faradic current, diadynamic current, high voltage, low voltage, low frequency, medium frequency, transcutaneous electrical nerve stimulation (TENS), electrical muscle stimulation (EMS), functional electrical stimulation (FES), Russian stimulation, and interferential stimulation. Unfortunately the multitude of names has created confusion regarding the physiologic effects and clinical results. Transcutaneous electrical nerve stimulation has been widely used to identify stimulators that modify pain, whereas neuromuscular electrical stimulation (NMES) or electrical muscle stimulation (EMS) has been identified with muscle reeducation, prevention of muscle atrophy, and enhanced joint movement. Accurate terminology dictates that almost all electrical stimulators are TENS units, as they work transcutaneously through surface electrodes to excite nerves. In the typical scenario where the muscle is innervated by a motor nerve, NMES is the appropriate terminology, and when a muscle is denervated and requires direct muscle fiber activation through electrical stimulation, the term EMS is used. Neuromuscular electrical stimulation, the primary focus of this chapter, is a form of clinical electrotherapy used to treat a wide variety of physiologic disorders or injuries in humans and is recognized as a promising treatment modality for similar disorders in veterinary patients. By definition, NMES is the administration of an electrical current generated by a stimulator that travels through leads to electrodes placed on the skin to depolarize the motor nerve and produce a skeletal muscle contraction. Stimulation of motor end plates with electrical current causes nerve depolarization and subsequent activation of muscle fibers.2,3 Electricity has been used therapeutically since Scribonius Largus used an electrical ray in a footbath to treat gout. Benjamin Franklin used electrical shock to treat a frozen shoulder in 1757.3 Electrical muscle stimulation has been specifically used in physical therapy since the mid-1700s, when electrostatic generators were used to treat patients with paralysis. In 1791 Galvani used galvanic current in in vitro experiments using neuromuscular preparations. By the early 1800s Faraday had invented the faradic current generator, which is the basis for most modern muscle stimulators. Documentation of NMES to reduce the loss of muscle weight and prevent atrophy in denervated muscles occurred in the early to mid-twentieth century. Electrotherapy has since become commonplace in physical medicine for the restoration of muscle function after injuries, before patients are capable of voluntary exercise training.4 To understand the benefits that electrical stimulation may provide in veterinary practice, a basic explanation and definition of the electrical current parameters used are summarized in Box 20-1. Three types of currents are commonly used: (1) continuous direct current (DC) (Figure 20-1, A), (2) continuous alternating current (AC) (Figure 20-1, B), and (3) pulsed current (either AC or DC) (Figure 20-1, C). Continuous direct current is a unidirectional electrical current that flows for 1 second or longer; alternating current changes the direction of flow at least once every second. Continuous direct current (also called galvanic current) has been used for wound healing, iontophoresis, and in the treatment of denervated muscle. In current clinical practice it is used only for iontophoresis. Continuous alternating current is not used therapeutically, but is our typical line current (see Figure 20-1, B). Pulsed current consists of a unidirectional or bidirectional flow of charges (DC or AC) that periodically stops for a finite time period. All NMES devices are pulsed current stimulators. Phase duration (also referred to as phase width) is defined as the time in which the current flows from the baseline in one direction and back to the baseline (Figure 20-2). Pulse duration (also referred to as pulse width) is defined as the time during which charge flows in both directions (see Figure 20-2). In a monophasic current, the pulse duration and the phase duration are the same. In a biphasic current, two phases make up one pulse. Pulsed current consisting of a bidirectional flow of charge is called biphasic pulsed current. When the flow in each direction is the same in time and amplitude-dependent features, the biphasic current pulse is considered symmetric (Figure 20-3, A). If the time- and amplitude-dependent features differ, then the biphasic current is termed asymmetric (Figure 20-3, B). Balanced asymmetric biphasic pulsed current occurs when the total charge in one phase equals the total charge of the other phase (Figure 20-4). If the total charge in each phase is unequal, it is termed unbalanced (Figure 20-5). Both pulsed AC and DC current forms are commonly used in portable and clinical model NMES units. Current amplitude (also called magnitude or intensity) is defined as the vertical distance from the highest to the lowest peak during one electrical wave and is typically measured in milliamperes (mA) (Figure 20-6). Increasing the amplitude induces a stronger force of muscle contraction by recruiting additional muscle fibers at greater distances from the electrodes.1,3 Skin produces resistance to current flow by ohmic resistance and capacitive impedance. Capacitive impedance is patient dependent and cannot be modified. Clipping or shaving the hair coat and cleaning the skin with alcohol to wash away skin oils or other substances helps to lower ohmic resistance. Lowering the skin resistance diminishes the driving voltage that is necessary for current penetration of the skin, potentially making the treatment more comfortable.1,3 Pulse durations of 150 to 400 microseconds produce powerful contractions while minimizing the likelihood of recruiting many pain fibers.1,3 Some stimulators that are made only for NMES do not allow control of pulse duration, but are typically set in this range. As the pulse duration increases, smaller diameter pain fibers are recruited. One study has examined pulse durations in dogs and subsequently recommended pulse durations by individual muscles (Table 20-1). This study also examined the differences in needed pulse durations between people and dogs. Although they are similar, dogs in general do not require as long a pulse duration as human motor nerves to depolarize. This can potentially increase comfort by using the shortest recommended pulse duration.5 Table 20-1 Recommended Pulse Durations for 11 Studied Muscles in Dogs *These recommended pulse durations are intervals centered over the mean values calculated in this study with an approximate range of ±10% or ±20 µs. This range facilitates the selection of a single chronaxy value when stimulating several muscles in 1 region. From Sawaya SG, Combet D, Chanoit G, Thiébault JJ, Levine D, Marcellin-Little DJ: Assessment of impulse duration thresholds for electrical muscle stimulation (chronaxy) in dogs. Am J Vet Res 69:1305-1309, 2008. Pulse rate (also called frequency, rate and pulses per second [pps]) is the number of pulses delivered per second and is measured in hertz (Hz). Tetanic muscle contractions may be produced with frequencies as low as 20 Hz, but only submaximal muscle contractions are typically produced in this range. Maximal force of contraction generally occurs between 60 and 100 Hz. However, as frequency increases the rate of fatigue also increases. Lower tetanic frequencies in the range of 35 to 50 pps reduce muscle fatigue while still providing strong muscle contractions.1,3 Duty cycle is the ratio of on-time to total cycle time, expressed as a percentage (Figure 20-7). On-time is the period of time in which a series of pulses or bursts are delivered to the patient. Off-time is the time between on-times. A single on-time duration plus a single off-time duration constitutes the total cycle time. For example, a stimulator that causes a muscle to contract for 10 seconds followed by a 30-second rest would have a 25% duty cycle, or a 1 : 4 ratio. As on-time increases, muscle fatigue increases.2 Optimal duty cycles vary, depending on the patient. A patient with severe atrophy may require a longer off-time to recover between contractions. Many clinicians start with duty cycle ratios between 1 : 2 and 1 : 5 and watch for signs of fatigue, which indicates the need for a longer off-time. Ramp is a feature of NMES that helps improve patient comfort. It involves a gradual increase or decrease in current amplitude such that the number of recruited motor units gradually increases the force of muscle contraction or gradually decreases the force of contraction (Figure 20-8). The ramp time is the period of time over which the pulse is increasing or decreasing. No data to date have identified the optimal ramp time. However, a ramp up time of 2 to 4 seconds is commonly used to maximize comfort with a 1- to 2-second ramp down.1 Suggested current parameters for muscle strengthening are listed in Box 20-2. Some commercially available electrodes are effective for only a few uses, and some may be used 100 or more times (e.g., carbon-impregnated silicon rubber electrodes, metal, etc.). Conductive performance of any electrode decreases over time. Electrodes require a medium to transmit current. Commonly used media include gels, moistened sponges or paper towels; some electrodes have the media already applied. Sponges and paper towels tend to dry out, and rewetting is necessary every 30 minutes. Electrodes should be of the appropriate size to stimulate the desired muscle without stimulating unwanted muscles in close proximity. The smaller the electrode, the higher the current density that enters the muscle, and the more uncomfortable the stimulus may be. An alternative to traditional electrodes is the use of electrodes with multiple fine wires or prongs that make contact with the skin (see Fig. 20-13). The advantage of these types of electrodes is that the hair does not need to be clipped. Rather the fine wire prongs are worked down among the hair to contact the skin. Wetting the hair before treatment helps with conductivity. Neuromuscular electrical stimulators recruit Type II (fast twitch) fibers first, then Type I (slow twitch), which is the reverse of the muscle recruitment pattern in a volitional contraction.6–8 Increasing the pulse duration increases the recruitment of smaller diameter motor units at the same depth, but increasing the pulse duration too much (800 µsec) may stimulate undesirable fibers (small-diameter pain fibers). Increasing either the amplitude or the pulse duration affects the strength of contraction because additional muscle fibers are recruited. Increasing the frequency results in the existing motor units firing at a faster rate and will increase the strength of contraction, but it also causes more rapid fatigue. Application of NMES at an optimal frequency results in an optimal physiologic response (the desired contraction) while minimizing fatigue. In a healthy uninjured individual, a maximal voluntary muscle contraction produces a greater torque (more powerful contraction) than occurs in an electrically induced contraction. However, patients with injuries or immediately following surgery may be unable or unwilling to produce a maximum voluntary muscle contraction. In these patients NMES may produce a stronger muscle contraction. Neuromuscular electrical stimulation is commonly used in the rehabilitation of human patients who have had orthopedic or neurologic injury. A few examples are patients recovering from fracture repair, ACL reconstruction, and meniscal debridement or repair. Patients with neurologic conditions, such as cerebrovascular accidents, closed head injuries, spinal cord injuries, or other neurologic disease involving paralysis or paresis, may also benefit from NMES. NMES has been used to increase joint mobility, decrease joint contracture, decrease edema, enhance circulation, minimize disuse atrophy, improve muscle strength, retard loss of volitional control, improve sensory awareness, decrease spasticity, diminish pain, and correct gait abnormalities.9–12 In human patients with low thoracic spinal cord injury, functional NMES has been used to produce knee extension with locking of the knees to allow weight bearing. Within 3 weeks of spinal cord injury, up to 50% of quadriceps muscle loss may occur. In one study NMES returned quadriceps muscle mass to near normal.11 In a patient with an incomplete quadriplegic spinal cord injury NMES was used to strengthen a paretic hand. Two weeks of NMES produced a 33% increase in muscle force with no loss of strength 4 weeks after the treatment was discontinued.11 Children with mild cerebral palsy treated with NMES had statistically significant improvements in gross motor, locomotor, and receipt/propulsion skills.10 Neuromuscular electrical stimulation has also been used on the tibial muscles of children with Duchenne and Becker muscular dystrophy. Neuromuscular electrical stimulation resulted in mild, short-term increases in muscle strength, but it did not alter muscle fatigue. Whether chronic stimulation produces beneficial long-term effects has yet to be determined.12 In addition to the clinical use of NMES in people with neurologic and orthopedic disorders, it has been used in denervated muscle to retard atrophy and improve recovery after reinnervation in rats with surgically severed peroneal nerves. The use of NMES in improving reinnervation recovery in other species warrants further study because it may be useful to those with denervation injuries.13 There are a number of circumstances in which electrical stimulation should not be used (contraindications), and other circumstances in which electrical stimulation should be used only with caution (precautions). Box 20-3 outlines these contraindications and precautions. In a study regarding the effects of low-frequency electrical stimulation on the metabolic profile of skeletal muscle, a portable NMES unit was used to stimulate the knee extensor muscles of sedentary men and women 6 days a week for 6 weeks.14 No changes in creatine kinase concentrations were found in vastus lateralis muscle samples before or after NMES. There was a small decrease in phosphofructokinase, the rate-limiting enzyme of glycolysis in human muscle, which was only statistically significant in men. Hexokinase, a regulatory enzyme of skeletal muscle glucose phosphorylation, was only significantly increased in the vastus lateralis of women. Krebs’ cycle and electron transfer chain marker enzyme activity of fatty acid oxidation increased significantly in both sexes, with the greatest change occurring in women. The authors concluded that NMES can significantly increase the skeletal muscle aerobic-oxidative potential of sedentary subjects. In a study by Arvidsson et al.15 there was no difference between a postoperative ACL reconstruction–NMES-treated group and an isometric exercise group in the activity of the oxidative enzyme citrate synthase or phosphofructokinase. Research by Wigerstad-Lossing et al.16 showed no significant reduction in the activity of the oxidative and glycolytic enzymes citrate synthase and triphosphate dehydrogenase in NMES patients following knee ligament surgery compared with untreated control knee ligament surgery patients, which had decreased enzyme activity.
Electrical Stimulation
Terminology Used in Electrical Stimulation
History of Electrical Stimulation
Basic Concepts of Electrical Stimulation
Electrical Current/Waveforms
Figure 20-1 A, Continuous direct current. B, Continuous alternating current. C, Pulsed currents (AC and DC).
Stimulator Parameters
Muscle
Chronaxy Value (µs)
Range of Recommended Pulse Durations for Use in Dogs* (µs)
Reported Human Chronaxy Range (µs)
Range of Recommended Pulse Durations for Use in Humans (µs)
Supraspinatus
167 ± 55 (133-200)
150-190
80-160
100-300, 150-250, 250
Infraspinatus
151 ± 61 (113-188)
130-170
80-160
100-300, 150-250, 250
Deltoideus
180 ± 60 (138-221)
160-200
80-160
100-300, 150-250, 200
Triceps brachii (lateral head)
217 ± 74 (171-262)
200-240
160-320
100-300, 150-250, 200
Extensor carpi radialis
190 ± 69 (141-238)
170-210
200-360
300, 150-250, 200
Gluteus medius
179 ± 69 (136-221)
160-200
80-160
300, 250-400, 400
Biceps femoris
207 ± 63 (167-246)
180-220
440-720
300, 250-400, 400
Semitendinosus
166 ± 25 (150-181)
150-190
440-720
300, 250-400, 400
Vastus lateralis
233 ± 73 (181-282)
210-250
80-160
300, 250-400, 400
Tibialis cranialis
238 ± 111 (160-314)
210-250
200-360
300, 250-400, 400
Erector spinae
205 ± 79 (155-255)
180-220
160-320
250, 150-250, 300
Electrodes
Recruitment
Indications and Contraindications for Neuromuscular Electrical Stimulation
Effects of Neuromuscular Electrical Stimulation in the Rehabilitation of Patients with Reconstructed Anterior Cruciate Ligaments
Effect on Muscle Enzymes
Stay updated, free articles. Join our Telegram channel

Full access? Get Clinical Tree
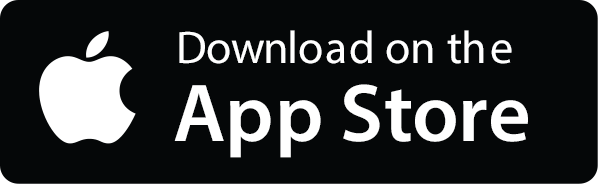
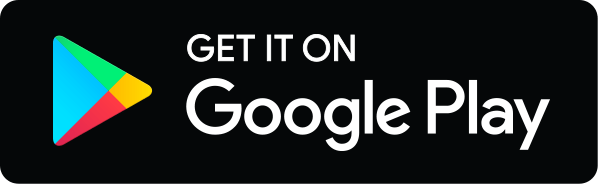