41 Assisted enteral and parenteral feeding
Introduction
The field of equine critical care has made many advances in the last few decades. Equine intensive care facilities are common in most referral hospitals and early recognition of disease coupled with more proactive and intensive therapy has resulted in increased success rates in the treatment of many surgical and medical diseases. Despite the significant changes in the way we approach the treatment of critical illness it is still relatively uncommon that we consider the nutritional status of the patient in our initial therapeutic plan. The nutritional status of a human prior to hospitalization, as well as early nutritional intervention during hospitalization, has clearly been linked to morbidity and mortality (Robert & Zaloga 2000, Ward 2003). Malnutrition has been shown to negatively impact survival, immune function, wound healing, and gastrointestinal function in humans as well as in animal models and most likely negatively affects numerous other processes (Keusch 2003, Robert & Zaloga 2000, Schroeder et al 1991, Studley 1936, Windsor & Hill 1988). Early nutritional intervention, whether by enteral or parenteral feeding, has been shown to improve measured outcomes in multiple human and animal studies. Unfortunately, it can be difficult to assess nutritional status of a horse prior to admission as information regarding intake and previous body condition may be lacking. However, a poor body condition or a history of recent weight loss can be indicators of inadequate nutritional intake. Despite the lack of data in the equine field, extrapolation from human medicine would strongly suggest that early nutritional support would likely decrease morbidity and mortality and improve long term recovery in the equine patient as well. This chapter discusses the metabolic consequences of food deprivation, the pathologic metabolic responses seen with illness, and the indications for, as well as the types of nutritional supplementation. Nutritional requirements will be mentioned when different from those of normal horses. Otherwise the reader is directed to other chapters in this book for more specific information on the core nutritional needs in the horse.
Effects of feed deprivation
Protein/calorie malnutrition
The average, healthy adult horse can apparently tolerate food deprivation (protein/calorie malnutrition (PCM) or simple starvation) for 24 to 72 hours with few systemic effects. The following description of metabolic alterations during starvation is derived from research in humans or other species unless otherwise stated. A decline in blood glucose concentration can occur with feed deprivation. Insulin concentrations decrease and energy demands are initially met via glycogenolysis resulting in an increase in the breakdown of liver glycogen stores. During the first hours to days of starvation glycogen stores are mobilized from various tissues (liver, kidney, muscle) for glucose production. Lipid mobilization is triggered by alterations in insulin/glucagon levels and the activity of hormone sensitive lipase. As glucose becomes limiting many body tissues begin to rely on fatty acid oxidation and the production of ketone bodies as energy sources. Glycerol produced from lipid degradation, lactate from the Krebs cycle and amino acids provided from muscle tissue breakdown continue to be utilized for gluconeogenesis to provide energy to glucose dependent tissues (central nervous system and red blood cells). This response to starvation correlates with an increase in circulating levels of growth hormone, glucagon, epinephrine, leptin and cortisol and a decrease in insulin and thyroid hormones. These hormone fluxes are an afferent stimulus for the hypothalamic response to starvation resulting in an increased drive to eat and a decrease in energy expenditure. Metabolism slows in an effort to conserve body fuels and the body survives primarily on fat stores, sparing lean tissue until such a time as re-feeding occurs (Hill 1992).
While no single definition of PCM, cachexia or malnutrition exists, a history of loss of 10–20% body mass is used by some authors to define cachexia (Corish & Kennedy 2000). Individuals with pre-existing PCM are at a disadvantage when intake is restricted due to surgery or illness. In both humans and laboratory animals nutritional supplementation has been shown to positively influence both survival and morbidity during critical illness (Stapleton et al 2007). Early nutritional supplementation should strongly be considered in horses presenting with preexisting PCM.
Catabolic response to injury and inflammation
In contrast to PCM, in humans and laboratory animals, the metabolic response to injury (critical illness, sepsis, trauma, surgical manipulation, etc. is characterized by an increased metabolism and the onset of a catabolic process leading to excessive breakdown of tissue proteins. This metabolic state is the result of a complex interaction of inflammatory cytokines (interleukin (IL)-1, IL-2, IL-6, tumor necrosis factor (TNF)-α and γ-interferon) released at the site of injury or inflammation, circulating hormones released in response to stress and injury (hypothalamic-pituitary-adrenal axis), and neurotransmitters (sympathoadrenal axis) (Romijn 2000). Infusion of cytokines, including IL-6 and TNF-α, results in release of corticotrophin, cortisol, epinephrine and glucagon resulting in an increase in the resting metabolic rate and lipolysis (Stouthard et al 1995, Van der Poll et al 1991). TNF-α activation of NFκB results in stimulation of proteolytic pathways (Langhans 2002). In response to injury there is increased metabolic activity of the brain. Afferent nerve activity and brain stimulation can result in autonomic nerve stimulation with direct effects on hormone secretion, for example, splanchnic nerve stimulation as a result of injury results in increased glucagon secretion and hyperglycemia (Bloom & Edwards 1975). Afferent nerve activity from the injured site also results in hypothalamic-pituitary activation increasing activity of cortisol, catecholamines, growth hormone, aldosterone and ADH (Romijn 2000). In humans, prolonged infusions of glucagon, cortisol and epinephrine resulted in increased protein breakdown and elevated resting metabolic rate (Bessey et al 1984). Prolonged elevation in cortisol is associated with onset of insulin resistance. In addition, peripheral nerve endings have been shown to exist on adipocytes; stimulation of which results in increased lipolysis. These responses are designed to provide endogenous substrates for gluconeogenesis, wound healing, immune cell replication and synthesis of acute phase. While this response is beneficial, long-term muscle breakdown results in loss of muscle strength and function and compromise to the patient’s overall health.
During illness or after trauma, food intake frequently falls. However, despite this decline in intake the adaptive responses to starvation do not occur. Hepatic gluconeogenesis continues and rapid protein catabolism occurs. There is an increased mobilization of stored fuels and metabolic cycling resulting in heat production and energy depletion. Insulin resistance develops and hyperglycemia may occur despite the absence of food intake. In severe metabolic stress the body appears to preferentially utilize amino acids from the breakdown of skeletal muscle proteins as a metabolic fuel, in contrast to PCM where fat is the principal source of energy. The adaptive switch to fat utilization is limited due, in part, to increased concentrations of circulating insulin. The result is an increase in lean tissue breakdown, visceral organ dysfunction secondary to loss of structural and enzymatic proteins, impaired wound healing (due to loss of precursors for wound healing) and immunosuppression (Romijn 2000, Sternberg et al 2000). Nitrogen losses during this catabolic response can be as high as 20–30 g/day versus 4–5 g/day in the same human experiencing PCM (Rafael & Mora 1999). Muscle weakness and increased morbidity result from excess protein breakdown as well as muscle disuse due to inactivity. As sodium and water retention are a component of this response (thought to be the result of a fall in the activity of the sodium/potassium pump (Na/K-ATPase) and cortisol induced fluid retention, weight loss and muscle wasting frequently goes unnoticed (Vanhorebeek & Van den Berghe 2004). Cytokine production results in behavioral changes including anorexia and decreased activity. Food deprivation during this hypermetabolic/catabolic state results in a much greater loss of lean muscle mass and visceral protein than would be expected during simple starvation. The metabolic adaptations to starvation in a healthy human can result in a 75% decrease in protein loss whereas the metabolic responses to critical illness result in an increase in energy and protein metabolism.
Nutritional supplementation will reverse the catabolic processes occurring during simple starvation but will not completely reverse those occurring during metabolic stress because as long as tissue injury persists catabolic processes are maintained. In the critically ill patient protein catabolism continues despite protein supplementation in the diet. The goals of nutritional support in critical illness should be to save life, preserve and improve cellular function and speed recovery (Powell-Tuck 2007). More recently, research in nutritional therapy has been focused on attenuating the metabolic response to stress, preventing oxidative cellular injury and favorably modulating the immune response with the ultimate goal being to reduce disease severity, complications and length of stay and improve outcome.
Key Points
• The metabolic response to prolonged protein/calorie malnutrition (starvation) in healthy individuals includes a fall in metabolic rate with sparing of body proteins and a switch by many tissues to the utilization of fat for energy
• The catabolic response to injury or inflammation may include an increased metabolic rate, continued utilization of protein and glucose as fuels despite a fall in nutritional intake
• Nutritional supplementation will not completely reverse the increased catabolism seen with injury and inflammation
Nutritional support
The need for nutritional support in the horse will depend on a number of factors. The healthy adult horse with a body condition score of 4 to 6/9 (Henneke system) with a minor injury or undergoing an elective surgery rarely requires nutritional supplementation even if the patient is unable or unwilling to eat for a few days. However, regardless of the type and complexity of the illness or injury, early nutritional support should be strongly considered in patients with increased metabolic demands, such as young growing animals, individuals presenting with a prior history of malnutrition or hypophagia, patients with underlying metabolic abnormalities that could worsen with feed deprivation and individuals with an illness such as severe trauma, sepsis or strangulating bowel obstructions that results in excess losses (ex-albumin loss through a compromised gastrointestinal tract) and an increased metabolic rate. Underweight horses (BCS of 3.5 out of 9 or less though this depends on the breed and individual characteristics) should ideally receive nutritional support early in the disease process rather than waiting to see if the individual will begin to eat on his/her own volition. Obese or over conditioned individuals particularly pony breeds, miniature horses and donkeys as well as lactating mares are at risk for developing hyperlipemia and should receive nutritional support if their serum triglycerides are above normal reference values (see Chapter 30). Older horses, or individuals diagnosed with equine Cushing’s syndrome or equine metabolic syndrome are insulin resistant and may be at greater risk for developing hyperlipemia, and fatty infiltration of their liver. Depending on the severity and type of underlying disease process and individual characteristics (breed, body condition) these individuals may develop severe hyperlipemia (triglycerides >500 mg/dl or >5.6 mmol/l) within days of onset. The combination of illness and metabolic effects of hyperlipemia can worsen the individual’s inappetance creating a vicious cycle. Consequently, if feed deprivation is prolonged (in some cases as little as 1–2 days of anorexia can result in moderate to severe hyperlipemia) or there is a concern regarding the individual’s desire or ability to eat, early intervention is indicated to prevent more severe malnutrition.
Nutritional needs of the sick or injured horse
While the energy requirements of horses of various ages, stages of growth and activity levels have been determined and equations are available to calculate these needs, the energy and protein requirements for the hospitalized, sick equine patient are not known and likely vary depending on disease state, environment and level of fitness of the individual. Historically, multipliers have been used to estimate the energy requirements in certain conditions including severe sepsis, trauma or burn injuries. However, the increased metabolic demand of illness or surgical trauma and recovery is likely to be balanced by the inactivity and decreased metabolic demands of digestion of the patient during hospitalization. Consequently, these multipliers likely overestimate the caloric requirement of certain illnesses. Studies in the human ICU and in sick neonatal foals suggest that the metabolic energy needs may fall more closely within the range of the resting to maintenance energy requirements of normal, healthy individuals (Cerra et al 1997, Paradis 2001, Stapleton et al 2007). The exceptions to this are individuals with extreme trauma, burns or severe sepsis, surgical conditions that require intestinal resection and patients with large areas of devitalized tissue (e.g., clostridial myositis patients that undergo multiple fasciotomy procedures). When estimating the energy requirements of the majority of other equine patients resting energy requirements are an acceptable target. Resting energy requirements (RER) are defined as the amount of energy needed to maintain an individual (no weight gain or loss) in a thermoneutral environment without the metabolic demands of digestion. Maintenance energy requirements include the demands of digestion in this equation and are ~30% higher than resting energy requirements. Resting energy requirements will vary slightly depending on the size of the horse but can be estimated using ~22–23 kcal/kg/day (92–96 kJ/kg/day) for the average full size horse. Maintenance energy requirements (as digestible energy) can be estimated using 30–35 kcal/kg/day. If a patient tolerates nutritional supplementation at resting energy requirements the feeding rate (i.e., calories/kg/day) can be gradually increased if needed.
Protein requirement
The maintenance protein requirement of the healthy adult horse is estimated at approximately 0.5–1.5 g/kg/day (see Chapter 6). The needs of a growing foal are higher and may approach 7 g/kg/day during maximum growth periods (Oftedal et al 1983); additionally the lysine requirements of growing horses are higher than for mature horses. During critical illness or severe injury protein catabolism and utilization of amino acids as a source of fuel continue despite the presence of other energy stores. Consequently, when calculating protein requirements in human patients it is recommended to provide the higher end of the estimated need (Cerra et al 1997, Powell-Tuck 2007). Another consideration is supplementation of nonessential amino acids that may improve outcome in illness. In humans, glutamine is considered a conditionally essential amino acid and is used as a fuel for enterocytes and other rapidly dividing cells (Lacey & Wilmore 1990). In periods of inappetance the majority of glutamine is produced by mobilization of muscle stores (Gamrin et al 1996). Glutamine release during critical illness may act as a cell signaling molecule regulating the inflammatory and immune response, however, in periods of illness when glutamine requirements may actually increase endogenous sources may be inadequate resulting in relative glutamine deficiency (Wischmeyer 2007). In laboratory animals, glutamine has been shown to be critical in maintaining gut wall integrity, immune function and antioxidant supplies (Wischmeyer 2007). Glutamine supplementation has been shown to improve clinical outcome in both laboratory and human studies (Novak et al 2002). In humans, glutamine supplementation at 0.2 g/kg/day is recommended when using the parenteral route. The amount and ideal route of glutamine supplementation has not been determined in the horse. In one study glutamine improved mucosal restitution in an oxidant injured equine colon model (Rotting et al 2004). Other recommendations for amino acid supplementation include branched chain amino acids and arginine supplementation. Arginine is a precursor to nitric oxide an important vasodilating agent, upregulates immune function, secretion of several hormones and may reduce ischemia-reperfusion injury (Potenza et al 2001, Ruth 1998). Studies looking at specific amino acid supplementation in critically ill or injured horses are currently lacking. Parenteral amino acid solutions that contain nonessential amino acids are available. When feeding by the enteral route, protein needs can be met by feeding a complete feed (which generally contain nonessential or conditionally essential amino acids) or by addition of specific amino acids to a component diet. For example, Critical Care Meals for horses (MD’s Choice, Louisville, TN) provides a separate component that contains glutamine, arginine and other non-essential amino acids. Alternatively, glutamine can be purchased separately and added to a diet though recommendations for amounts are currently not available. Caution may be required in horses with liver failure as some studies in humans have demonstrated that deamination of glutamine is a major contributor to hyperammonemia (Romero-Gomez et al. 2009).
Routes of nutritional support
The choice whether to use the enteral or parenteral route of feeding can be summarized by the simple statement “if the gut works use it.” While there is conflicting data regarding the pros and cons of parenteral feeding verses enteral feeding, it is clear that enteral feeding has some distinct benefits. Enteral nutrients provide the majority of nutrition to the gut which is responsible for 15–35% of the total body oxygen consumption and protein turnover (Stoll et al 1998). Enteral feeding offers the advantage of maintaining the gastrointestinal tract, and is generally less expensive. Enteral feeding has been shown to improve gut barrier integrity, gut mass, protein content, motility, and function in piglets (Burrin et al 2000). The complete absence of enteral nutrition results in mucosal atrophy, increased gut permeability and enzymatic dysfunction in critically ill human patients (Hernandez et al 1999). Even small amounts of enteral feeding may provide benefits. In a neonatal piglet model, provision of 5–10% of nutritional needs by enteral feeding resulted in improved intestinal motility and lactose digestion and decreased mucosal permeability. Enteral provision of 20% of energy and nutrient needs for normal growth prevented loss of gut protein mass whereas 40–60% of nutritional needs were needed to maintain normal gastrointestinal growth (Burrin et al 2000).
The enteral route of nutritional support is simpler as there is less concern of fluid and electrolyte overload and this route allows fiber feeding, which cannot be achieved parenterally. That said, clinicians are often hesitant to use the enteral route in horses with gastrointestinal disorders. In the critically ill patient with poor perfusion and decreased oxygen delivery to the tissues the gastrointestinal tract is one of the most vulnerable organs to ischemia. Decreased oxygen delivery has been shown to increase mucosal permeability resulting in increased translocation of bacteria and absorption of bacterial toxins (Luyer et al 2004, Saito et al 1987). Inflammatory mediators, produced in the gut as a result of ischemia, are absorbed across the damaged mucosa and enter the portal and systemic circulations; this absorption has been implicated in the onset of septic shock or multi-organ failure (Rokyta et al 2003). Inflammation and enterocyte injury can result in decreased motility and nutrient absorption. In this situation institution of forced enteral feeding may result in colic, bacterial overgrowth, diarrhea and other complications. Because digestion increases the metabolic activity of the enterocytes enteral feeding during states of poor oxygen delivery may actually worsen the oxygen debt and ischemic injury. In contrast, enteral nutrition has been shown to increase total hepatosplanchnic blood flow in healthy patients resulting in greater oxygen delivery to the mucosa suggesting that enteral feeding may be beneficial in the poorly perfused gut (Rokyta et al 2003). In a rat Escherichia coli sepsis model, enteral feeding of glucose resulted in improved intestinal perfusion rates (Gosche et al 1990
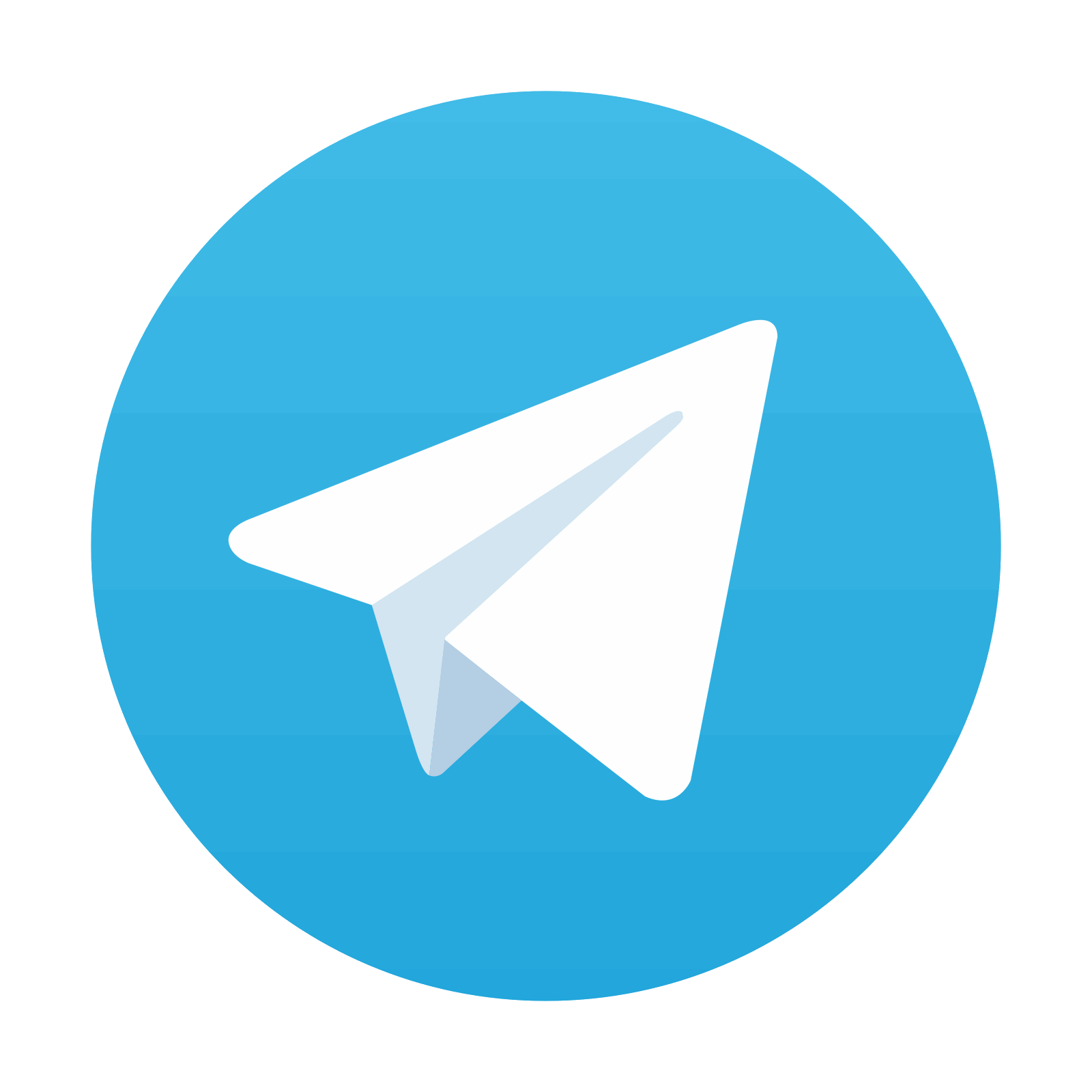
Stay updated, free articles. Join our Telegram channel

Full access? Get Clinical Tree
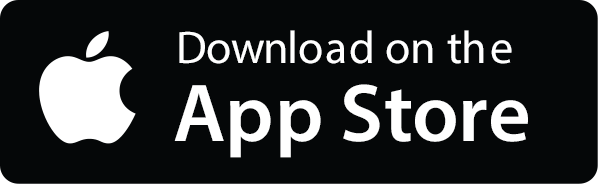
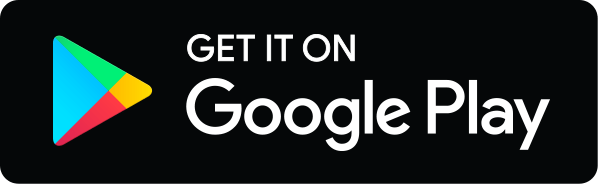